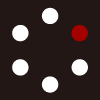
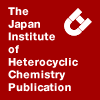
HETEROCYCLES
An International Journal for Reviews and Communications in Heterocyclic ChemistryWeb Edition ISSN: 1881-0942
Published online by The Japan Institute of Heterocyclic Chemistry
e-Journal
Full Text HTML
Received, 18th December, 2014, Accepted, 26th January, 2015, Published online, 5th February, 2015.
DOI: 10.3987/COM-14-13155
■ Novel Total Synthesis of the 2-Azaanthraquinone Alkaloid Scorpinone Using a Tandem Oxidation and Azaelectrocyclic Reaction
Tominari Choshi,* Miharu Hironaka, Miki Goto, Kaori Shimizu, Yuhki Kurata, Takashi Nishiyama, Noriyuki Hatae, and Satoshi Hibino*
Faculty of Pharmacy and Pharmaceutical Sciences, Fukuyama University, Fukuyama, Hiroshima 729-0292, Japan
Abstract
A new tandem oxidation and electrocyclic reaction of a 1-aza 6π-electron system derived from a known 3-hydroxynaphthalene derivative was developed for the synthesis of 2-azaanthraquinones. Total synthesis of scorpinone (1a) was achieved in seven steps using this new tandem reaction. In addition, the positional isomer, 7,9-dimethoxy-4-methylbenzo[g]isoquinoline-5,10-dione (1b) was also synthesized in the same way.INTRODUCTION
Naturally occurring 2-azaanthraquinones are a class of polyketide-derived heterocycles produced by fungi or lichens1-3 and are of special interest due to their important biologic properties,4,5 includimg anti-HIV activity.6 Bostrycoidin, tolypocladin, scopinone and their related natural compounds are members of the 2-azaanthraquinone family based on studies of their biosynthesis.1- 3 An unsubstituted 2-azaanthraquinone, benz[g]isoquinoline-5,10-dione, that inhibits the growth of multi-drug resistant pathogens has also been isolated from Psychotria componutans and Mitracarpus scaber.4,5
In 1954, naturally occurring 2-azaanthraquinone bostrycoidin (2) possessing antibiotic properties was first isolated from Fusarium bostrycoides7 and the structure was assigned by Arsenault in 1965.8 Bostrycoidin was subsequently also isolated from several Fusarium species8-10 and produced from Nectria haematococca.11 The physiologic importance of 2-azaanthraquinones led to the development of several synthetic methods. The first total synthesis of bostrycoidin (2) was reported by Cameron.12 In 1987, Watanabe reported the formal total synthesis of 2 using 4-selective lithiation of N,N-diisopropylnicotinamide, followed by condensation with N,N-dimethyl-2,3,5-trimethoxybenzamide.13 Most pathways involved a Diels-Alder cycloaddition reaction, followed by an intramolecular Friedel-Crafts reaction to obtain the appropriate building blocks, in which the nitrogen is already incorporated.14-17
The metal-chelating properties of tolypocladin (3), from the mycelium of the cyclosporine producing fungus Tolypocladium inflatum, was demonstrated in 1990.18a Graefe also reported that bostrycoidin (2) and tolypocladin (3) inhibit Ca2+ - and calmodulin-dependent cAMP phosphodiesterase.18b In 1997, the intermolecular Friedel-Crafts acylation of 1,2,4-trimethoxybenzene and 2-methylpyridine-4,5- dicarboxylic acid anhydride, followed by the subsequent intramolecular Friedel-Crafts reaction, was used for the first synthesis of tolypocladin (3).15 The synthesis of tolypocladin (3) was also reported by Krapcho16 and De Kimpe,17 using the synthetic route for bostrycoidin (2).
Scopinone (1a) was isolated from the mycelium of a Bispora-like tropical fungus,19 and from cultures of the mycobionts of the lichen Haematomma sp., respectively, in 2001.20 Scorpinone (1a) was also recently independently isolated from the fungus Amorosia littoralis21 and from the fungus Ascomycete IBWF79B-90A.22 Anke22 reported that scorpinone (1a) exhibited cytotoxic activities against several cell lines. In 2005, the first total synthesis of scorpinone (1a) was established by De Kimpe17 through the construction of a naphthoquinone skeleton based on a [4 + 2] cycloaddition of an appropriate electron-rich and oxygenated diene, followed by cyclization of 2-phenoxymethyl-3- acetonylnaphthoquinones with ammonia according to the modified Parisot procedure.23
We are performing the synthesis of bioactive nitrogen-containing fused-heteroaromatic compounds including natural products with a thermal electrocyclic reaction using either a 6π- or aza 6π-electron system including an aromatic or heteroaromatic double bond in principle.24 Among them, we reported the total synthesis of furo[3,2-h]isoquinoline,25 phenanthridine,26 β-carboline,27 2-azaanthraquinone,28 benzo[c]phenanthridine,29 indolo[3,2-c]quinolone,30 and pyrano[2,3,4-ij]isoquinoline31 alkaloids by the construction of fused pyridine ring systems using a microwave-assisted thermal electrocyclic reaction of an aza 6π-electron system. In 2008, we reported the total synthesis of the 2-azanthraquinone alkaloid scopinone (1a) in nine steps (8% overall yield) using two key reactions of a microwave-assisted thermal electrocyclic reaction for the synthesis of 8-oxygenated isoquinoline skeleton from a 1-azahexatriene system, followed by a regioselective microwave-assisted [4+2] cycloaddition for construction of the 2-azaanthraquinone framework (Scheme 1). The 7-bromoisoquinolinequinone 8 was obtained from the 8-hydroxyisoquinoline 6 in somewhat low yield (23% in two steps), because the 5,7-dibromo- isoquinoline 7 was very unstable. Therefore, we planned a new synthetic route.
In this report, we describe an alternative synthesis of scorpinone (1a) together with its positional isomer of the methyl group, 7,9-dimethoxy-4-methylbenzo[g]isoquinoline-5,10-dione (1b) by the construction of the 2-azaanthraquinone ring system based on a new tandem oxidation and a thermal electrocyclic reaction of a 1-aza 6π electron system.
RESULTS AND DISCUSSION
In the retrosynthetic analysis (Scheme 2), we envisaged that scorpinone (1a) and its positional isomer (1b) of methyl group could be derived from 5-oxyganated 2-azaanthracene 10 by oxidation. 2-Azaanthracene 10 might be obtained by a microwave-assisted thermal electrocyclic reaction of 3-alkenylnaphtharene-2-aldoxime methyl ether 11, 1-azahexatriene system, derived from a cleavage of the 2,3-bond of the 2-azaanthracene framework. The aldoxime methyl ether 11 could be easily obtained from the known naphthalene 13.32
To synthesize an 1-azahexatriene system, the naphthalene 13 was prepared according to Greene’s procedure.32 As shown in Scheme 3, treatment of 3-hydroxynaphthalene 13 with trifluoromethanesulfonic anhydride (Tf2O) afforded the triflate 12 (96%), which was subjected to the Stille reaction with two kind of alkenyltributyltins in the presence of PdCl2(PPh3)2 to give 3-alkenylnaphthalenes 14a (92%) and 14b (91%), respectively. The reduction of naphthalenes 14 with LiAlH4 followed by oxidation with active MnO2 gave naphthalene-2-carbaldehydes 16a and 16b in 89% and 63% yields. Subsequent cleavage of methyl ethers of 16a and 16b with BBr3 afforded 1-hydroxynaphthalenes 17a (85%) and 17b (38%), which were treated with O-methylhydroxylamine to produce aldoximes 11a (81%) and 11b (78%), respectively.
We next examined the construction of 2-azaanthracene 10 using a microwave (MW)-assisted thermal electrocyclic reaction to the aldoxime 11a (Scheme 4). However, the desired 2-azaanthracene 10 could not be yielded. Therefore, we attempted to convert the naphthalene aldoxime 11a into a naphoquinone aldoxime 18a by an oxidation. The naphthalene aldoxime 11a was subjected to the oxidation reaction using cerium ammonium nitrate (CAN). As a result, scorpinone (1a) was isolated as the sole product in 19% yield, however the expected naphth-1,4-quinone 18a could be not detected. This experimental fact suggested that not only the oxidation reaction occurred, but an azaelectrocyclic ring closure also occurred at the room temperature, consecutively.
As shown in Table 1, treatment of 11a with Fremy’s salt afforded scorpinone (1a) in low yield (run 2). When salcomine with oxygen33 was used, product 1a was obtained in 64% yield (run 3). Although oxidation reactions at slightly high temperatures under the same conditions were investigated (runs 4 and 5), yields of both reactions were not improved. When MeCN or DMSO was used as a solvent instead of DMF was used under the same conditions (runs 6, 7), scorpinone (1a) was obtained in 42% and 61% yields, respectively. The condition of run 3 was the most effective procedure in terms of the yield. The physical and spectroscopic data of our synthetic scorpinone (1a) were identical to the previously reported data. Furthermore, treatment of the aldoxime 11b with the best conditions (run 3) afforded the positional isomer of the methyl group, 7,9-dimethoxy-4-methylbenzo[g]isoquinoline-5,10-dione (1b) in 49% yield (run 8).
In this experiment, the azaelectrocyclic reaction of 1-azahexatriene containing 2,3-double bond of naphthalene 11 did not proceed at 180 oC in 1,2-dichlorobenzene25-28 with or without microwave irradiation due to the stable naphthalene 11 of the 10π electron system. By contrast, we have considered that the azaelectrocyclic reaction of naphthoquinone 18 proceeded at room temperature due to the lack of aromaticity.
CONCLUSION
In the present study, we achieved a new and efficient total synthesis of scorpinone (1a) in 31% overall yield in seven steps from the readily available known starting material 13, using a new tandem oxidation and azaelectrocyclic reaction. The overall yield was remarkably improved over that of our earlier report (8% in nine steps).28 In addition, this new tandem reaction was applied to the synthesis of the positional isomer 1b of the methyl group. This new tandem reaction would be applicable procedure for a synthesis of other alkaloid possessing a fused pyridine ring system.
EXPERIMENTAL
General Methods: All non-aqueous reactions were carried out under an atmosphere of nitrogen in dried glassware unless otherwise noted. Solvents were dried and distilled according to standard protocols. Analytical thin-layer chromatography was performed with Silica gel 60PF254 (Merck). Silica gel column chromatography was performed with Silica gel 60N (63-210 mm, KANTO CHEMICAL Co. Ltd.). All melting points were determined on Yanagimoto micro melting point apparatus and are uncorrected. Proton nuclear magnetic resonance (1H NMR) spectra were recorded on a JEOL AL-300 at 300 MHz. Chemical shifts are reported relative to Me4Si (δ 0.00). NMR spectra were measured with CDCl3 unless otherwise noted. Carbon nuclear magnetic resonance (13C NMR) spectra were recorded on a JEOL AL-300 at 75 MHz. Chemical shifts are reported relative to CDCl3 (δ 77.0). Infrared spectra were recorded with ATR method using a Shimadzu FTIR-8000 spectrophotometer and technologies DuraScop. Low and high resolution mass spectra were recorded on JEOL JMS-700 spectrometers by direct inlet system. The reaction of microwave (MW) irradiation was carried out by Discover of CEM Co. Ltd. with 2450 MHz.
Methyl 3-trifluoromethylsulfonyloxy-1,6,8-trimethoxynaphthalene-2-carboxylate 12
Trifluoromethanesulfonic anhydride (0.34 mL, 2.0 mmol) was added to a solution of the 2-naphthol 13 (0.54 mg, 1.9 mmol) and pyridine (0.29 mL, 3.7 mmol) in CH2Cl2 (30 mL) under cooling with ice-water. After stirring at rt for 30 min, the reaction mixture was quenched with saturated aqueous NaHCO3 solution. The mixture was extracted with CH2Cl2. The organic layer was washed with water, brine, and dried over Na2SO4, and concentrated under reduced pressure. The residue was purified by column chromatography (silica gel, 20 g) using EtOAc-hexane (2:8) as an eluent to give the triflate 12 (750 mg, 96%). mp 119-120 oC. IR (ATR) ν: 1724, 1619 cm–1. 1H NMR (300 MHz CDCl3) δ: 3.92 (3H, s), 3.93 (3H, s), 3.96 (3H, s), 3.98 (3H, s), 6.59 (1H, d, J=2.0 Hz), 6.73 (1H, d, J= 2.0 Hz), 7.39 (1H, s). 13C NMR (75 MHz CDCl3) δ: 52.7, 55.5, 56.2, 64.5, 99.2, 100.5, 100.5, 114.6, 115.0, 138.1, 143.9, 157.45, 157.8, 160.4, 164.2. MS (EI) m/z: 424 (M+); HRMS (EI) Calcd for C16H15F3O8S: 424.0440. Found: 424.0450.
Methyl 1,6,8-trimethoxy-3-(prop-1-en-1-yl)naphthalene-2-carboxylate 14a
A solution of tributyl(propenyl)tin (1.34 g, 4.1 mmol) was added to a mixture of the triflate 12 (1.15 g, 2.7 mmol), PdCl2(PPh3)2 (51 mg, 0.27 mmol), and Et4NCl (674 mg, 4.1 mmol) in DMF (25 mL) at rt under an argon atmosphere. The stirred mixture was heated at 80 oC for 1.5 h, which was cooled to rt. After being quenched with an aqueous solution of 30% KF (20 mL), and then the mixture was stirred at rt for 30 min. The mixture was filtered off through Celite pad and the filtrate was extracted with EtOAc. The EtOAc layer was washed with water and brine, dried over Na2SO4, and concentrated under reduced pressure. The residue was purified by column chromatography (silica gel, 20 g) using EtOAc-hexane (1:4 v/v) as an eluent to give 3-propenylnaphthalene 14a (790 mg, 92%). mp 60-62 oC. IR (ATR) ν: 1731 cm–1. 1H NMR (300 MHz CDCl3) δ: 1.87 (3H/2, dd, J= 1.7, 7.5 Hz), 1.90 (3H/2, d, J= 5.6 Hz), 3.86 (3H/2, s), 3.87 (3H/2, s), 3.90 (3H/2, s), 3.91 (3H, s), 3.95 (3H/2, s), 3.96 (3H/2, s), 3.97 (3H/2, s), 5.85-5.96 (1H/2, m), 6.24-6.45 (3H/2, m), 6.49 (1H/2, d, J= 2.1 Hz), 6.52 (1H/2, d, J= 2.1 Hz), 6.70 (1H/2, d, J= 2.1 Hz), 6.71 (1H/2, d, J= 2.1 Hz), 7.36 (1H/2, s), 7.53 (1H/2, s). MS (EI) m/z: 316 (M+); HRMS (EI) Calcd for C18H20O5: 316.1311. Found: 316.1318.
Methyl 3-isopropenyl-1,6,8-trimethoxynaphthalene-2-carboxylate 14b
The same procedure as above was carried out using the triflate 12 (100 mg, 0.24 mmol) and tributyl(isopropenyl)tin (0.12 g, 0.35 mmol) to give the 3-isopropenylnaphthalene 14b (68 mg, 91%). mp 99-100 oC. IR (ATR) ν: 1724 cm–1. 1H NMR (300 MHz CDCl3) δ: 2.13 (3H, br s), 3.87 (3H, s), 3.88 (3H, s), 3.90 (3H, s), 3.96 (3H, s), 5.06-5.07 (1H, m), 5.175.18 (1H, m), 6.52 (1H, d, J= 2.1 Hz), 6.70 (1H, d, J= 2.1 Hz), 7.34 (1H, s). 13C NMR (75 MHz CDCl3) δ: 23.8, 52.1, 55.3, 56.1, 64.0, 98.8, 99.3, 114.4, 115.5, 121.7, 123.4, 138.1, 140.0, 143.6, 154.3, 157.4, 159.1, 169.0. MS (EI) m/z: 316 (M+); HRMS (EI) Calcd for C18H20O5: 316.1311. Found: 316.1291.
[1,6,8-Trimethoxy-3-(prop-1-en-1-yl)naphthalen-2-yl]methanol 15a
A solution of 3-propenylnaphthalene 14a (1.21 g, 3.84 mmol) in THF (20 mL) was added to a suspension of LiAlH4 (291 mg, 7.68 mmol) in THF (20 mL) under ice-water. The mixture was stirred at rt for 2 h, which was quenched with water, and then the mixture was extracted with EtOAc. The EtOAc layer was washed with brine, dried over Na2SO4, and concentrated under reduced pressure. The residue was purified by column chromatography (silica gel, 20 g) using EtOAc-hexane (3:7 v/v) as an eluent to give the alcohol 15a (1 g, 89 %). mp 61-63 oC. IR (ATR) ν: 2938 cm–1. 1H NMR (300 MHz CDCl3) δ: 1.80 (6H/5, dd, J=1.2, 6.8 Hz), 1.94 (9H/5, dd, J=1.7, 6.4 Hz), 3.86 (9H/5, s), 3.89 (6H/5, s), 3.89 (9H/5, s), 3.90 (6H/5, s), 3.97 (9H/5, s), 3.99 (6H/5, s), 4.82 (4H/5, s), 4.89 (6H/5, s), 5.96 (2H/5, dq, J=7.2, 11.4 Hz), 6.24 (3H/5, dq, J=6.6, 15.6 Hz), 6.48 (3H/5, d, J=2.3 Hz), 6.52 (2H/5, d, J=2.3 Hz), 6.69 (1H, d, J=2.3 Hz), 6.70 (2H/5, d, J=11.4 Hz), 6.83 (3H/5, d, J=15.6 Hz), 7.31 (2H/5, s), 7.51 (3H/5, s). MS (EI) m/z: 288 (M+); HRMS (EI) Calcd for C17H20O4: 288.1362. Found: 288.1364.
[3-Isopropenyl-1,6,8-trimethoxynaphthalen-2-yl]methanol 15b
The same procedure as above was carried out using the 3-isopropenylnaphthalene 14b (739 mg, 2.34 mmol) to give the oily alcohol 15b (458 mg, 68%). IR (ATR) ν: 2939 cm–1. 1H NMR (300 MHz CDCl3) δ: 2.14-2.15 (3H, m), 2.49 (1H, t, J=5.8 Hz), 3.90 (3H, s), 3.92 (3H, s), 3.99 (3H, s), 4.79 (2H, d, J=5.8 Hz), 5.00-5.01 (1H, m), 5.27-5.28 (1H, m), 6.52 (1H, d, J=2.1 Hz), 6.70 (1H, d, J=2.1 Hz), 7.28 (1H, s). 13C NMR (75 MHz CDCl3) δ: 25.3, 55.3, 56.0, 58.5, 62.9, 98.7, 98.9, 114.7, 115.7, 122.1, 126.5, 137.5, 143.9, 145.0, 155.7, 156.9, 158.4. MS (EI) m/z: 288 (M+); HRMS (EI) Calcd for C17H20O4: 288.1362. Found: 288.1376.
1,6,8-Trimethoxy-3-(prop-1-en-1-yl)naphthalene-2-carbaldehyde 16a
A mixture of the alcohol 15a (374 mg, 1.3 mmol) and activated MnO2 (1.13 g, 13 mmol) in CH2Cl2 (20 mL) was stirred at room temperature for 27 h. The reaction mixture was then filtered through a Celite pad and the Celite pad was washed with CH2Cl2. The combined CH2Cl2 was concentrated under reduced pressure. The residue was purified by column chromatography (silica gel, 30 g) using EtOAc-hexane (1:9) as an eluent to give the aldehyde 16a (331 mg, 89%). mp 61-63 oC. IR (ATR) ν: 1670 cm–1. 1H NMR (300 MHz CDCl3) δ: 1.82 (21H/37, dd, J=2.0, 7.2 Hz), 1.95 (90H/37, dd, J=2.0, 6.0 Hz), 3.93 (180H/37, s), 3.94 (21H/37, s), 3.95 (21H/37, s), 4.01 (90H/37, s), 4.02 (21H/37, s), 5.90 (7H/37, dq, J=7.2, 11.8 Hz), 6.18 (30H/37, dq, J=6.0, 15.5 Hz), 6.15 (30H/37, d, J=2.8 Hz), 6.54 (7H/37, d, J=2.8 Hz), 6.71 (1H, d, J=2.8 Hz), 6.94 (7H/37, d, J=11.8 Hz), 7.29 (30H/37, d, J=15.5 Hz), 7.47 (1H, s), 10.62 (7H/37, s), 10.65 (30H/37, s). MS (EI) m/z: 286 (M+); HRMS (EI) Calcd forC17H18O4: 286.1205. Found: 286.1180.
3-Isopropenyl-1,6,8-trimethoxynaphthalene-2-carbaldehyde 16b
The same procedure as above was carried out using the alcohol 15b (384 mg, 1.33 mmol) to give the oily aldehyde 16b (260 mg, 63%). IR (ATR) ν: 1678 cm–1. 1H NMR (300 MHz CDCl3) δ: 2.05-2.06 (3H, m), 3.92 (3H, s), 3.95 (3H, s), 4.01 (3H, s), 4.92-4.93 (1H, m), 5.15-5.16 (1H, m), 6.54 (1H, d, J=2.2 Hz), 6.71 (1H, d, J=2.2 Hz), 7.24 (1H, s), 10.55 (1H, s). 13C NMR (75 MHz CDCl3) δ: 24.1, 55.5, 56.2, 65.1, 99.3, 99.4, 113.5, 14.3, 123.0, 123.8, 140.9, 143.0, 146.8, 158.3, 161.0, 163.7, 190.9. MS (EI) m/z: 286 (M+); HRMS (EI) Calcd forC17H18O4: 286.1205. Found: 286.1216.
1-Hydroxy-6,8-dimethoxy-3-(prop-1-en-1-yl)naphthalene-2-carbaldehyde 17a
To a solution of aldehyde 16a (331 mg, 1.16 mmol) in CH2Cl2 (15 mL) at -78 oC was added BBr3 (165 mL, 1.74 mmol), and then the mixture was stirred at an ambient temperature for 3 h. The reaction mixture was quenched with aqueous NH4Cl, and the mixture was extracted with EtOAc. The EtOAc layer was washed with brine, dried over Na2SO4, and concentrated under reduced pressure. The residue was purified by column chromatography (silica gel, 10 g) using EtOAc-hexane (3:17 v/v) as an eluent to give the 1-hydroxynaphthalene 17a (267 mg, 85%). mp 62-64 oC. IR (ATR) ν: 2938, 1600 cm–1. 1H NMR (300 MHz CDCl3) δ: 1.75 (3H/3, dd, J=2.0, 7.0 Hz), 1.95 (6H/3, dd, J=2.0, 7.6 Hz), 3.92 (6H/3, s), 3.93 (3H/3, s), 4.00 (6H/3, s), 4.01 (3H/3, s), 4.02 (21H/37, s), 6.03 (1H/3, dq, J=7.0, 11.4 Hz), 6.17 (2H/3, dq, J=7.6, 15.5 Hz), 6.45 (2H/3, d, J=2.6 Hz), 6.49 (1H/3, d, J=2.6 Hz), 6.62 (1H, d, J=2.6 Hz), 6.68 (1H/3, d, J=11.4 Hz), 6.86 (1H/3, s), 6.89 (2H/3, d, J=15.5 Hz), 7.03 (2H/3, s), 10.05 (1H/3, s), 10.21 (2H/3, s), 14.05 (2H/3, br s), 14.25 (1H/3, br s). MS (EI) m/z: 272 (M+); HRMS (EI) Calcd for C16H16O4: 272.1049. Found: 272.1023.
1-Hydroxy-3-isopropenyl-6,8-dimethoxynaphthalene-2-carbaldehyde 17b
The same procedure as above was carried out using the aldehyde 15b (365 mg, 1.27 mmol) to give the 1-hydroxynaphthalene 17b (132 mg, 38%). mp 84-85 oC. IR (ATR) ν: 2939, 1604 cm–1. 1H NMR (300 MHz CDCl3) δ: 2.15-2.16 (3H, m), 3.91 (3H, s), 4.00 (3H, s), 5.03-5.04 (1H, m), 5.38-5.39 (1H, m), 6.47 (1H, d, J=2.2 Hz), 6.62 (1H, d, J=2.2 Hz), 6.90 (1H, s), 10.00 (1H, s), 14.28 (1H, s). 13C NMR (75 MHz CDCl3) δ: 25.6, 55.5, 56.2, 98.5, 99.6, 110.3, 111.4, 117.0, 117.0, 117.8, 141.8, 142.2, 144.1, 161.1, 162.4, 162.4, 165.7, 195.0. MS (EI) m/z: 272 (M+); HRMS (EI) Calcd for C16H16O4: 272.1049. Found: 272.1068.
1-Hydroxy-6,8-dimethoxy-3-(prop-1-en-1-yl)naphthalene-2-carbaldehyde O-methyloxime 11a
A mixture of the 1-hydroxynaphthalene 17a (267 mg, 0.98 mmol), MeONH2•HCl (164 mg, 1.96 mmol), and AcONa (161 mg, 1.96 mmol) in EtOH (20 mL) was heated at 80 oC for 3 h. After removal of solvent followed by addition of water, the mixture was extracted with EtOAc. The EtOAc layer was washed with water and brine, dried over Na2SO4, and concentrated under reduced pressure. The residue was purified by column chromatography (silica gel, 10 g) using EtOAc-hexane (1:4 v/v) as an eluent to give the oxime 11a (238 mg, 81%). IR (ATR) ν: 2935 cm–1. 1H NMR (300 MHz CDCl3) δ: 1.63 (9H/4, dd, J=1.8, 6.9 Hz), 1.85 (3H/4, dd, J=1.8, 6.7 Hz), 3.82 (3H/4, s), 3.82 (9H/4, s), 3.90 (9H/4, s), 3.92 (3H/4, s), 3.93 (3H/4, s), 3.93 (9H/4, s), 5.86 (3H/4, dq, J=6.9, 11.4 Hz), 6.05 (1H/4, dq, J=6.7, 16.5 Hz), 6.37 (1H/4, d, J=2.0 Hz), 6.40 (3H/4, d, J=2.0 Hz), 6.49 (3H/4, d, J=11.4 Hz), 6.55 (3H/4, d, J=2.0 Hz), 6.56 (1H/4, d, J=2.0 Hz), 6.70 (1H/4, d, J=16.5 Hz), 6.89 (1H, s), 7.08 (1H/4, s), 7.19 (3H/4, s), 11.12 (1H/4, br s), 11.49 (3H/4, br s). MS (EI) m/z: 301 (M+); HRMS (EI) Calcd for C17H19NO4: 301.1314. Found: 301.1318.
1-Hydroxy-3-isopropenyl-6,8-dimethoxynaphthalene-2-carbaldehyde O-methyloxime 11b
The same procedure as above was carried out using the 1-hydroxynaphthalene 17b (87 mg, 0.32 mmol) to give the oxime 11b (76 mg, 78%). mp 72-74 oC. IR (ATR) ν: 2935 cm–1. 1H NMR (300 MHz CDCl3) δ: 2.08 (3H, br s), 3.90 (3H, s), 3.97 (3H, s), 4.01 (3H, s), 4.95-6.96 (1H, m), 5.30-5.31 (1H, m), 6.48 (1H, d, J=2.2 Hz), 6.62 (1H, d, J=2.2 Hz), 6.98 (1H, s), 8.45 (1H, s), 11.67 (1H, s). 13C NMR (75 MHz CDCl3) δ: 25.4, 55.3, 56.2, 62.3, 98.4, 98.8, 106.8, 111.0, 116.8, 117.1, 138.4, 143.2, 143.9, 150.5, 157.1, 159.3, 159.7. MS (EI) m/z: 301 (M+); HRMS (EI) Calcd for C17H19NO4: 301.1314. Found: 301.1325.
Scorpinone 1a
A mixture of oxime 11a (20 mg, 0.066 mmol) and salcomine (4.3 mg, 0.013 mmol) in DMF (5 mL) under bubbling O2 was stirred at rt for 3 h. After being quenched with water, a reaction mixture was extracted with EtOAc. The EtOAc layer was washed with water and brine, dried over Na2SO4, and concentrated under reduced pressure. The residue was purified by column chromatography (silica gel, 10 g) using EtOAc-hexane (1:1 v/v) as an eluent to give scorpinone 1a (12 mg, 64%). mp 193-196 oC. IR (ATR) ν: 1678, 1673 cm–1. 1H NMR (300 MHz CDCl3) δ: 2.79 (3H, s), 3.99 (3H, s), 4.03 (3H, s), 6.82 (1H, d, J=2.7 Hz), 7.44 (1H, d, J=2.7 Hz), 7.81 (1H, s), 9.41 (1H, s). 13C NMR (75 MHz CDCl3) δ: 25.1, 56.1, 56.6, 103.6, 105.5, 115.7, 117.5, 125.5, 137.0, 137.5, 149.8, 162.8, 164.2, 165.0, 180.6, 183.6. MS (EI) m/z: 283 (M+); HRMS (EI) Calcd for C16H13NO4: 283.0845. Found: 283.0819.
7,9-Dimethoxy-4-methylbenzo[g]isoquinoline-5,10-quinone 1b
The same procedure as above was carried out using the oxime 11b (25 mg, 0.086 mmol) to give the benzoisoquinoline-5,10-dione 13 (12 mg, 49%). mp 235-237 oC. IR (ATR) ν: 1655, 1577 cm–1. 1H NMR (300 MHz CDCl3) δ: 2.75 (3H, s), 4.00 (3H, s), 4.03 (3H, s), 6.82 (1H, d, J=2.7 Hz), 7.40 (1H, d, J=2.7 Hz), 8.81 (1H, s), 9.41 (1H, s). 13C NMR (75 MHz CDCl3) δ: 19.1, 56.0, 56.6, 103.4, 104.9, 115.3, 128.2, 132.4, 134.7, 138.0, 148.3, 157.0, 162.4, 165.2, 180.9, 185.2. MS (EI) m/z: 283 (M+); HRMS (EI) Calcd for C16H13NO4: 283.0845. Found: 283.0833.
ACKNOWLEDGEMENTS
This work was supported in part by Grant-in Aid for Scientific Research (C) (No. 15590033 for T.C., No.22590010 for N.H., and No. 24590040 for S.H.) from the Ministry of Education, Culture, Sports, Science and Technology of Japan.
References
1. R. M. Van Wagoner, P. G. Mantle, and J. L. C. Wright, J. Nat. Prod., 2008, 71, 426. CrossRef
2. E. M. K. Wijeratne, B. P. Bashyal, M. K. Gunatilaka, A. E. Arnold, and A. A. L. Gunatilaka, J. Nat. Prod., 2010, 73, 1156. CrossRef
3. T. Awakawa, T. Kaji, T. Wakimoto, and I. Abe, Bioorg. Med. Chem. Lett., 2012, 22, 4338. CrossRef
4. A. L. Okunade, A. M. Clark, C. D. Hufford, and B. O. Oguntimein, Planta Med., 1999, 65, 447. CrossRef
5. A. J. Nok, Cell Biochem. Funct., 2002, 20, 205. CrossRef
6. H. Hong, N. Neamati, H. E. Winslow, J. L. Christensen, A. Orr, Y. Pommier, and G. W. A. Milne, Antivial Chem. Chemothe., 1998, 9, 46; Z. Yang, J. Ding, K. Ding, D. Chen, S. Cen, and M. Ge, Phytochemi. Lett., 2013, 6, 257. CrossRef
7. F. A. Cajori, T. T. Otani, and M. A. Hamilton, J. Biol. Chem., 1954, 208, 107; M. A. Hamilton, M. S. Knorr, and F. A. Cajori, Antibiot. Chemother., 1953, 3, 853.
8. G. P. Arsenault, Tetrahedron Lett., 1965, 4033; CrossRef G. P. Arsenault, Tetrahedron, 1968, 24, 4745. CrossRef
9. I. Kurobane, L. C. Vining, A. G. Mclnnes, and N. N. Gerber, J. Antibiot., 1980, 33, 1376. CrossRef
10. D. Parisot, M. Devys, and M. Barbier, Phytochemistry, 1990, 29, 3364. CrossRef
11. Y. Yamamoto, Y. Kinoshita, T. G. Ran, M. Hasumi, K. Kinoshita, K. Koyama, K. Takahashi, and I. Yoshimura, Phytochemistry, 2002, 60, 741. CrossRef
12. D. W. Cameron, K. R. Deutscher, G. I. Feutrill, and D. E. Hunt, Aust. J. Chem., 1982, 35, 1451; CrossRef D. W. Cameron, K. R. Deutscher, and G. I. Feutrill, Aust. J. Chem., 1982, 35, 1349; D. W. Cameron, K. R. Deutscher, and G. I. Feutrill, Tetrahedron Lett., 1980, 21, 5089; CrossRef V. H. Deshpande, R. A. Khan, and N. R. Ayyanger, Indian J. Chem., 1996, 35B, 965.
13. M. Watanabe, E. Shinoda, Y. Shimizu, S. Furukawa, M. Iwao, and T. Kuraishi, Tetrahedron, 1987, 43, 5281. CrossRef
14. E. Ohgaki, J. Motoyoshima, S. Narita, T. Kakurai, S. Hayashi, and K.-I. Hirakawa, J. Chem. Soc., Perkin Trans. 1, 1990, 3109. CrossRef
15. W. Werner, U. Gräfe, W. Ihn, D. Tresselt, S. Winter, and E. Paulus, Tetrahedron, 1997, 53, 109. CrossRef
16. A. P. Krapcho and D. J. Waterhouse, Heterocycles, 1999, 51, 737. CrossRef
17. S. Claessens, G. Vermiest, J. Jacobs, E. V. Hende, P. Habonimana, T. N. Van, L. V. Puyvelde, and N. De Kimpe, Synlett, 2007, 829; V. T. Nuguyen, G. I. Vernist, S. Claessens, and N. De Kimpe, Tetrahedron, 2005, 61, 2295; CrossRef B. Kesteleyn and N. De Kimpe, J. Org. Chem., 2000, 65, 640; CrossRef B. Kesteleyn, N. Tuyen, and N. De Kimpe, Tetrahedron, 1999, 55, 2091; CrossRef S. Claessens, J. Jacobs, and N. De Kimpe, Synlett, 2007, 741.
18. U. Gräfe, W. Ihn, D. Tresselt, N. Miosga, B. Kaden, B. Schlegel, E. J. Bormann, P. Sedmera, and J. Novak, Biol. Metals, 1990, 3, 39; CrossRef D. Gräfe and W. Römer, Pharmazie, 1991, 46, 297.
19. A. Miljkovic, P. G. Mantle, D. J. Williams, and B. Rassing, J. Nat. Prod., 2001, 64, 1251. CrossRef
20. Y. Moriyasu, H. Miyagawa, N. Hamada, H. Miyawaki, and T. Ueno, Phytochemistry, 2001, 56, 239. CrossRef
21. R. M. Van Wagoner, P. G. Mantle, and J. L. C. Wright, J. Nat. Prod., 2008, 71, 426; CrossRef P. G. Mantle, D. Hawksworth, S. Pazoutova, L. M. Collinson, and B. R. Rassing, Mycological Res., 2006, 110, 1371. CrossRef
22. A. Schüffler, J. C. Liermann, H. Kolshorn, T. Opatz, and H. Anke, Z. Naturforsch., 2009, 64c, 25.
23. D. Parisot, M. Devys, and M. Barbier, J. Antibiot., 1989, 42, 1189. CrossRef
24. T. Choshi and S. Hibino, Heterocycles, 2011, 83, 1205; CrossRef T. Choshi and S. Hibino, Heterocycles, 2009, 77, 85; CrossRef T. Choshi, Yakugaku Zasshi, 2001, 121, 487; CrossRef S. Hibino and E. Sugino, In Advances in Nitrogen Heterocycles, ed. by C. J. Moody, JAI Press, Greenwich, CT (USA), 1995, 1, 205; T. Kawasaki and M. Sakamoto, J. Indian Chem. Soc., 1994, 71, 443; Y. Hieda, T. Choshi, H. Fujioka, and S. Hibino, Eur. J. Org. Chem., 2013, 7391. CrossRef
25. T. Kumemura, T. Choshi, A. Hirata, M. Sera, Y. Takahashi, J. Nobuhiro, and S. Hibino, Chem. Pharm. Bull., 2005, 53, 393; CrossRef T. Kumemura, T. Choshi, J. Yukawa, A. Hirose, J. Nobuhiro, and S. Hibino, Heterocycles, 2005, 66, 87; CrossRef T. Choshi, T. Kumemura, H. Fujioka, Y. Hieda, and S. Hibino, Heterocycles, 2012, 84, 587. CrossRef
26. T. Kumemura, T. Choshi, J. Yukawa, A. Hirose, J. Nobuhiro, and S. Hibino, Heterocycles, 2005, 66, 87. CrossRef
27. K. Omura, T. Choshi, S. Watanabe, Y. Satoh, J. Nobuhiro, and S. Hibino, Chem Pharm. Bull., 2008, 56, 237; CrossRef S. Tagawa, T. Choshi, A. Okamoto, T. Nishiyama, S. Watanabe, N. Hatae, and S. Hibino, Heterocycles, 2013, 87, 357; CrossRef S. Tagawa, T. Choshi, A. Okamoto, T. Nishiyama, S. Watanabe, N. Hatae, M. Ishikura, and S. Hibino, Eur. J. Org. Chem., 2013, 1805. CrossRef
28. T. Choshi, T. Kumemura, J. Nobuhiro, and S. Hibino, Tetrahedron Lett., 2008, 49, 3725. CrossRef
29. K. Kohno, S. Azuma, T. Choshi, J. Nobuhiro, and S. Hibino, Tetrahedron Lett., 2009. 50, 590; CrossRef Y. Ishihara, S. Azuma, T. Choshi, K. Kohno, K. Ono, H. Tsutsumi, T. Ishizu, and S. Hibino, Tetrahedron, 2011, 67, 1320. CrossRef
30. K. Hayashi, T. Choshi, K. Chikaraishi, A. Oda, R. Yoshinaga, N. Hatae, M. Ishikura, and S. Hibino, Tetrahedron, 2012, 68, 4274. CrossRef
31. Y. Tazaki, Y. Tsuchiya, T. Choshi, T. Nishiyama, N. Hatae, H. Nomoto, and S. Hibino, Heterocycles, 2014, 89, 427. CrossRef
32. A. Piettre, E. Chevenier, C. Massardier, Y. Gimbert, and A. E. Greene, Org. Lett., 2002, 4, 3139. CrossRef
33. R. H. Bailes and M. Calvin, J. Am. Chem. Soc., 1947, 69, 1886; CrossRef H. M. VanDort and H. J. Geursen, Recl. Trav. Chim. Pays-Bas., 1967, 86, 520; CrossRef N. Kuwabara, H. Hayashi, N. Hiramatsu, T. Choshi, T. Kumemura, J. Nobuhiro, and S. Hibino, Tetrahedron, 2004, 60, 2943 CrossRef