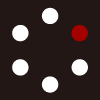
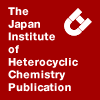
HETEROCYCLES
An International Journal for Reviews and Communications in Heterocyclic ChemistryWeb Edition ISSN: 1881-0942
Published online by The Japan Institute of Heterocyclic Chemistry
e-Journal
Full Text HTML
Received, 21st December, 2014, Accepted, 27th January, 2015, Published online, 3rd February, 2015.
DOI: 10.3987/COM-14-13159
■ Intramolecular Methionine Residue and Its Peptide Segments Stabilize Dehydroandrographolide Succinate-Methoxypolyethylene Glycol Conjugates
Senhao Li, Siliang Feng, Lei Qiao, Qingbin Meng, Huajin Dong, Zehui Gong, Dongqin Quan, and Keliang Liu*
Department of Medicinal Chemistry, Beijing Institute of Pharmacology and Toxicology, No. 27 Taiping Road, Haidian District, Beijing 100850, China
Abstract
The antipyretic, analgesic, and anti-inflammatory activities of dehydroandrographolide succinate (DAS), an andrographolide derivative, are superior to its parent compound. However, its instability and poor water solubility have restricted its clinical use. To solve these problems, a series of DAS conjugates with functional amino acids or peptides coupled to methoxypolyethylene glycol (mPEG) were designed and synthesized. Conjugation of DAS directly to mPEG improved the compound solubility in aqueous solution, but the thermostability of the conjugate was reduced compared with the parent compound. Introduction of methionine (Met) or oligomethionyl peptides, DAS-Metn-mPEG (n = 1, 2, or 3), protected the DAS skeleton from ring opening and double bond oxidation, thus improving the anti-inflammatory properties of these derivatives compared with DAS.INTRODUCTION
Andrographolide, chemically designated as (3-[2-[decahydro-6-hydroxy-5-(hydroxymethyl)-5, 8-adimethyl-2-methylene-1-napthalenyl]ethylidene]dihydro-4-hydroxy-2(3H)-furanone), is one of the major diterpene lactone components of Andrographis paniculata Nees (Acanthaceae). Recent pharmacological studies have shown that andrographolide and its derivatives have antibacterial,1 anti-inflammatory,2 antiviral,3 anticancer,4 anti-HIV,5 liver-protecting,6 and antioxidant activities.1 Dehydroandrographolide succinate (DAS, Figure 1A) is obtained from esterification and dehydration of andrographolide, and it has greater antibacterial and anti-inflammatory activities compared with the parent compound.7 Preliminary structure-activity relationship studies have shown that the dicycloditerpenoid and the five-membered lactone ring of DAS are important pharmacophores and that the number and location of the α, β-double bonds influence the antibacterial and anti-inflammatory activities.8 However, DAS is unstable due to oxidation of the double bond and hydrolysis of the γ-lactone as well as the C-3 and C-19 ester bonds.9 Moreover, its water solubility is less than 1 mg/mL, which is unsatisfactory for clinical use. Poor water solubility and stability make it an unattractive drug candidate. Therefore, to modify DAS and make derivatives with better physicochemical properties, modifications should be carefully designed to maintain the bioactivity of the parent structure.
Polyethylene glycol (PEG) has been approved by the U.S. Federal Drug Administration for use as an injectable medicinal polymer with amphipathic properties and no apparent side effects. The properties of PEG-drug molecule or PEG-protein conjugates are often better than those of the drug molecule or protein alone.10 PEG-drug conjugates have several advantages, including a dramatically improved water solubility, an extended exposure time in the body, a decreased degradation by metabolic enzymes, and a reduction or elimination of protein immunogenicity.11 Several PEG-drug conjugate molecules have been approved for clinical use.12 The oral PEG-naloxone (NKTR-118) conjugate, jointly developed by Therapeutics and AstraZeneca, is currently in Phase III clinical trials for the treatment of opioid-induced bowel dysfunction.13 PEG-SN38,14 developed by Enzon Therapeutics, and NKTR-102 (PEG-IRINOTECAN),15 developed by Nektar Therapeutics, are in Phase II clinical trials.
The water solubility of many hydrophobic drugs has been improved by conjugation with methoxypolyethylene glycol (mPEG), which has physicochemical properties that enable the formation of a hydration layer with water through hydrogen bonds.16 However, the introduction of mPEG can reduce the temperature stability of the compound;17 and mPEG tends to get oxidized, releasing free radicals, and is degraded at high temperatures.18 Therefore, to overcome the problems associated with mPEG conjugation, we analyzed the stabilities of DAS, DAS-mPEG conjugates, and DAS-mPEG conjugates with methionine (Met) residues inserted between DAS and mPEG (Figure 1B). We hypothesized that the Met residues may protect DAS from conjugated double bond oxidation and ring opening caused by free radicals. Met2, Met3, phenylalanine (Phe), leucine (Leu), lysine (Lys), and glutamate (Glu) residues were also incorporated into the conjugates to investigate DAS protection. We hypothesized that the benzyl side chain of Phe and the isobutyl side chain of Leu may protect the conjugated double bond and lactone ring of DAS by hydrophobic interactions, thus increasing stability, and that introducing water-soluble Lys and Glu with a positively charged ε-amino group and a negatively charged carboxyl group, respectively, may increase electrostatic interactions to enhance stability.
RESULTS AND DISCUSSION
Water solubility
To increase the thermostability and water solubility of DAS, we designed the series of DAS-X-mPEG conjugates shown in Figure 1B. The aqueous solubility of DAS and its potassium salt (DAS-K), a drug used clinically in China, were extremely poor (<1 g·L-1); while the solubility of the conjugates were higher than that of DAS (Table 1). The conjugates with amino acids or peptides (4–10) showed water solubility comparable to those of the PEGylated conjugates (1–3) (Table 1). The ability of mPEG to form a hydration layer with water through hydrogen bond association has been documented.19
Activity evaluation
All conjugates administered intravenously to mice at a dosage of 175 μmol·kg-1 significantly inhibited auricular swelling caused by xylene (Table 1), compared with the control group (P < 0.05). Compared with DAS-K, 1, 7, and 8 had the greatest anti-inflammatory activities. Significant differences (P < 0.05) in the swelling degree between DAS-K and these three conjugates indicated that the introduction of mPEG1000 may be the critical factor that increased the anti-inflammatory activities of these compounds. Compared with 1, the anti-inflammatory activities of the conjugates with functional amino acids or peptides were similar (P > 0.05). Inhibition of auricular swelling in mice after the administration of 2 was significantly less than that induced by the other conjugates. It is possible that the two long PEG side chains delayed drug release. In summary, all conjugates had similar or improved anti-inflammatory activities compared with DAS-K.
Stability
The stabilities of the conjugates were studied according to the experimental design (Table 2). The stabilities of 1, 2, and 3 were investigated in aqueous solution. DAS-K was barely soluble in water, so it was prepared with excipients. The results revealed that the introduction of mPEG affected the thermal stability of DAS and that mPEG1000 was a relatively better conjugate group compared with the others. The stability of 2 was significantly less than those of 1 and 3. The functional amino acids and carefully designed peptides were chosen to further improve the properties of conjugate 1. As expected, the stabilities of the conjugates with Met residues were superior to the DAS and DAS-mPEG conjugates, possibly due to the antioxidant activity of Met and the reduced incidence of DAS oxidation.
Oxidation was one of the main causes of conjugate degradation. HPLC analysis of compound 4 after 10 days at 40 °C in aqueous solution showed the introduction of a new peak (Figure 2). Identification of this new peak by MALDI-TOF-MS indicated that its molecular weight was 16 Da more than that of 4, indicating that the Met residue was oxidized from the thioether to the sulfoxide. PEG is also susceptible to free radical oxidative attack because strain at the chain folds lowers the activation energy of hydrogen abstraction.18 The introduction of Met captured oxygen and prevented PEG from further degradation. In fact, Met is prone to oxidation to Met-R,S-sulfoxide by reactive oxygen species from intracellular and extracellular origins.20 For the other conjugates, a series of minor impurities was found in the HPLC traces and there was no main impurity, possibly due to the degradation of PEG, which generates impurities with different molecular weights.
Most organisms have one methionine sulfoxide reductase A (msrA) gene and one to three methionine sulfoxide reductase B (msrB) genes (msrB1–3). These genes encode enzymes that are different in sequence and structure. MsrA and MsrB reduce Met-S-sulfoxide and Met-R-sulfoxide, respectively.21 Both enzymes have similar catalytic mechanisms, and their reactive center conformations are mirror images. These reductases are widely distributed in mammalian organs and subcellular structures, indicating that the oxidation products of Met-containing conjugates are unlikely to cause toxicity. Therefore, we introduced Met dipeptides and tripeptides to increase the antioxidant activity, thus further protecting the DAS conjugates. With the addition of Met, the stability of the conjugates in solution increased. The degradation of the conjugates protected with dipeptides and tripeptides at 40 °C after 10 days was less than 5%, indicating better stability compared with Met alone. The stability of 6 was significantly better than those of 1 and DAS-K.
Considering that hydrolysis is also one of the main causes of drug degradation and that DAS-K could be dissolved in ethanol, ethanol was investigated as a low dielectric constant solvent. The stabilities of 1, 6, and DAS-K in 10% ethanol solution were tested (Table 3). The stability of 1 was superior to that of DAS-K in the same solution, indicating that the introduction of mPEG1000 could increase the stability of the DAS core. The stability of 6 was also high in the ethanol solution. Thus, low dielectric constant solvents such as ethanol reduce conjugate hydrolysis.
We expected that the addition of a hydrophobic residue or a residue with a positive or negative charge would also improve conjugate stability. On the contrary, introduction of Phe, Leu, Lys, or Glu reduced the conjugate stability. Compared to the conjugate containing a Met residue (4), we confirmed that the degradation of conjugate 1 was mainly caused by oxidation. Therefore, none of the other amino acids had antioxidant effects. The hydrophobic groups in the side chains of Phe and Leu as well as the electrostatic interactions of the positively charged Lys amino group and the negatively charged Glu carboxyl group did not inhibit the oxidation. In contrast, they undermined the stability of the conjugate and accelerated DAS degradation.
CONCLUSIONS
Our present work focused on improving the poor water solubility, anti-double bond oxidation, and hydrolysis of the γ-lactone as well as the C-3 and C-19 ester bonds of DAS by conjugation of DAS with mPEG using various amino acids and peptides as functional linkers. We successfully designed and synthesized DAS-X-mPEG conjugates that have improved physicochemical properties compared to DAS, without losing activity. By introducing different functional amino acids or oligopeptides, the poor thermal stabilities of the mPEG-conjugates were greatly improved. The antioxidant activities of the Met residues reduced the oxidation of DAS and PEG, and the anti-inflammatory activities of all conjugates were similar or better than that of DAS. The incorporation of mPEG played a leading role in increasing the solubility of the conjugates, and all DAS derivatives met the solubility requirements for drug research and development, eliminating the need for excipients that may cause adverse reactions. This strategy may be effective for the modification of other poorly soluble and unstable drugs.
EXPERIMENTAL
Materials. DAS and DAS-K were obtained from YiPing Pharmaceutical Technical Development Limited Company (Chengdu, China). mPEGs (1000 Da, 2000 Da, and 5000 Da) were purchased from Sigma-Aldrich (USA). Boc-Met-OH, H-Met-OMe·HCl, Boc-Phe-OH, Boc-Leu-OH·H2O, Fmoc-Lys(Boc)-OH, Boc-Glu(OtBu)-OH, N-ethyl-N’-3-dimethylaminocarbodiimide hydrochloride (EDCI), and 4-dimethylaminopyridine (DMAP) were purchased from GL Biochem Ltd. (Shanghai, China). Glucose solution (5%) (500 mL/bottle, batch number: 20080228) was purchased from KeMiOu Limited Company (Tianjin, China). Chromatographic grade reagents were used for RP-HPLC analysis, and other reagents were analytical grade.
Analytical RP-HPLC runs were carried out on an Agilent Series 1200 system (USA) using a C18 column (150 × 4.6 mm ID; Venusil ASB, Agela Technologies, Tianjin, China) at a flow rate of 1.0 mL·min-1. For all the RP-HPLC procedures, the solvent system used was 0.1% TFA/H2O (A) and 0.1% TFA/70% MeCN/H2O (B). Separations were achieved by applying a linear gradient of B from 60% to 100% over 10 min and monitoring at 210 nm and 251 nm. 1H-NMR data were obtained using a JNM-ECA-400 type superconducting nuclear resonance spectrometer (Japan). The molecular weight distribution data were obtained on a MALDI-TOF-MS from Bruker using α-cyano-4-hydroxycinnamic acid as the matrix (Germany).
Animals. Kunming mice (SPF grade, male, 18–22 g) were provided by the Laboratory Animal Centre of the Beijing Institute of Pharmacology and Toxicology (permission number: SCXK-2007-004). Animals were housed at 22–26 ºC with 40–60% humidity in a 12-h light-dark cycle (illumination from 8 am to 8 pm). Each cage housed 6–8 mice, which were allowed access to water and food ad libitum. The experimental protocol was approved by the Ethics Committee on Animal Research of the Beijing Institute of Pharmacology and Toxicology (Beijing, China), and animal experiments were performed according to the Guiding Principles for the Care and Use of Laboratory Animals of the Beijing Institute of Pharmacology and Toxicology.
Synthesis of DAS-X-mPEG conjugates
DAS-(mPEG1000)2 (1). To make 1, DAS (2.53 g, 5 mmol) was dissolved in dichloromethane (DCM) (100 mL) and EDCI (2.14 g, 11 mmol) was added. The mixture was stirred for 30 min, and mPEG1000 (11.02 g, 11 mmol) and DMAP (0.061 g, 0.5 mmol) were added. The reaction was complete after 8 h. The mixture was concentrated, applied to a silica gel column, and eluted with a solution of MeOH in DCM (MeOH:DCM = 1:30). A total of 5.68 g of pure product was obtained as a white solid in 45.2% yield. 1H NMR (400 MHz, CDCl3) δ7.18 (s, 1H), 6.91 (m, 1H), 6.13 (d, J = 15.6 Hz, 1H), 4.82 (s, 2H), 4.80 (s, 1H), 4.63 (m, 1H), 4.56 (s, 1H), 4.25 (m, 6H), 3.4–3.9 (m, 198H), 3.38 (s, 6H), 2.66 (m, 8H), 2.45 (d, J = 12.1 Hz, 1H), 2.34 (d, J = 10.0 Hz, 1H), 2.03 (m, 1H), 1.85 (d, J = 12.1 Hz, 1H), 1.68 (m, 2H), 1.55 (m, 2H), 1.27 (m, 2H), 1.02 (s, 3H), 0.88 ppm (s, 3H). MALDI-TOF-MS: m/z calc. 2496, [M+H]+ normally distributed from 2080.72 to 3359.67.
DAS-(mPEG2000)2 (2). 2 was made according to the methods described for 1 in 39.5% yield. 1H NMR (400 MHz, CDCl3) δ7.18 (s, 1H), 6.92 (m, 1H), 6.13 (d, J = 15.6 Hz, 1H), 4.82 (s, 2H), 4.80 (s, 1H), 4.62 (m, 1H), 4.56 (s, 1H), 4.26 (m, 6H), 3.4–3.9 (m, 488H), 3.38 (s, 6H), 2.66 (m, 12H), 2.45 (d, J = 12.1 Hz, 1H), 2.34 (d, J = 10.0 Hz, 1H), 2.01 (m, 1H), 1.85 (d, J = 12.1 Hz, 1H), 1.68 (m, 2H), 1.55 (m, 2H), 1.27 (m, 2H), 1.02 (s, 3H), 0.87 ppm (s, 3H). MALDI-TOF-MS: m/z calc. 4496, [M+H]+ normally distributed from 3973.46 to 4855.09.
DAS-mPEG5000 (3). 3 was made according to the methods described for 1 in 31.2% yield. MALDI-TOF-MS: m/z calc. 5514, [M +H]+ normally distributed from 4709.04 to 5548.11. Although the reaction to make 3 was performed under the same conditions as those for 1, the dimer was not obtained, possibly due to steric hindrance.
DAS-(Met-mPEG1000)2 (4). To make 4, Boc-Met-mPEG1000 was made first. mPEG1000 (3 g, 3 mmol) was dissolved in DCM (50 mL) and Boc-Met-OH (2.24 g, 9 mmol), EDCI (1.73 g, 9 mmol), and DMAP (0.11 g, 0.9 mmol) were added successively. The mixture was incubated at room temperature overnight with stirring and then applied to a silica gel column. Boc-Met-mPEG1000 (2.70 g) was obtained in 73.1% yield as a white powder after precipitation with Et2O. The Boc protecting group was removed by 50% TFA/DCM to give 1.74 g of H-Met-mPEG1000·TFA as a white powder. Next, DAS (0.37 g, 0.7 mmol) was dissolved in DCM (30 mL), and EDCI (0.40 g, 2.1 mmol) and HOBt (0.19 g, 1.4 mmol) were added. The mixture was stirred for 30 min, and then H-Met-mPEG1000·TFA (1.90 g, 1.5 mmol) and DIEA (0.25 mL, 1.5 mmol) were added. The reaction was complete 2 h later. DCM was evaporated, and the mixture was dissolved in water and applied to a reverse-phase silica gel column. A total of 0.66 g of was obtained in 34.4% yield, with a purity of 97.5% according to HPLC analysis. 1H NMR (400 MHz, CDCl3) δ7.18 (s, 1H), 6.90 (m, 1H), 6.81 (m, 1H), 6.13 (d, J = 15.6 Hz, 1H), 4.82 (s, 2H), 4.80 (s, 1H), 4.73 (m, 1H), 4.65 (m, 1H), 4.56 (s, 1H), 4.26 (m, 6H), 3.4–3.9 (m, 208H), 3.39 (s, 6H), 2.56 (m, 12H), 2.09 (m, 11H), 1.81 (d, 1H), 1.60 (m, 4H), 1.29 (m, 3H), 1.00 (s, 3H), 0.86 ppm (s, 3H). MALDI-TOF-MS: m/z calc. 2758, [M +H]+ normally distributed from 2651.24 to 3311.38.
DAS-(Met-Met-mPEG1000)2 (5). To make 5, Boc-Met-Met-OMe was made first. A solution of Boc-Met-OH (12.47 g, 50 mmol) was dissolved in DCM (150 mL), and EDCI (14.38 g, 75 mmol) and HOBt (6.75 g, 50 mmol) were added. The mixture was stirred for 30 min, and H-Met-OMe·HCl (9.99 g, 50 mmol) and DIEA (8.27 mL, 50 mmol) were added. The reaction was complete after 2 h. The mixture was applied to a silica gel column, and 11.76 g of Boc-Met-Met-OMe was obtained as a white solid in 59.6% yield. The methyl ester was hydrolyzed by 2 M sodium hydroxide solution to give 10.76 g of Boc-Met-Met-OH as a white powder in 97.6% yield.
Next, Boc-Met-Met-OH (3.42 g, 9 mmol), EDCI (1.73 g, 9 mmol), and DMAP (0.11g, 0.9 mmol) were added successively to a solution of mPEG1000 (3 g, 3 mmol) dissolved in DCM (50 mL). The mixture was incubated at room temperature overnight and then applied to a silica gel column. A total of 3.95 g of Boc-Met-Met-mPEG1000 was obtained as a white powder in 73.1% yield. The Boc protecting group was removed by 50% TFA/DCM to give 3.70 g of H-Met-Met-mPEG1000·TFA as a white powder in 98.2% yield.
Finally, EDCI (0.58 g, 3 mmol) and DMAP (0.03 g, 0.2 mmol) were added to a solution of DAS (0.53 g, 1 mmol) dissolved in DCM (30 mL). The mixture was stirred for 30 min, and H-Met-Met-mPEG1000·TFA (2.73 g, 2.1 mmol) and DIEA (0.35 mL, 2.1 mmol) were added. Two hours later, HPLC analysis indicated that the reaction was complete. DCM was evaporated, and the residue was dissolved in water and applied to a reverse-phase silica gel column; 1.33 g of 5 was obtained. The purity, as measured by HPLC, was 97.6%, and the yield was 44.0%. 1H NMR (400 MHz, CDCl3) δ7.19 (s, 1H), 6.90 (m, 1H), 6.13 (d, J = 15.6 Hz, 1H), 4.82 (s, 2H), 4.80 (s, 1H), 4.56 (m, 8H), 3.4–3.9 (m, 202H), 3.38 (s, 6H), 2.56 (m, 16H), 2.09 (m, 21H), 1.66 (m, 4H), 1.27 (m, 2H), 1.02 (s, 3H), 0.85 ppm (s, 3H). MALDI-TOF-MS: m/z calc. 3020, [M +H]+ normally distributed from 3000.86 to 3441.52.
DAS-(Met-Met-Met-mPEG1000)2 (6). 6 was obtained according to the methods described for 5. A total of 0.59 g of 6 was obtained in 17.99% yield, with a purity of 99.4% according to HPLC analysis. 1H NMR (400 MHz, CDCl3) δ7.19 (s, 1H), 6.90 (m, 1H), 6.13 (d, J = 15.6 Hz, 1H), 4.82 (s, 2H), 4.80 (s, 1H), 4.56 (m, 8H), 3.4–3.9 (m, 192H), 3.38 (s, 6H), 2.56 (m, 20H), 2.09 (m, 31H), 1.66 (m, 4H), 1.25 (m, 2H), 1.02 (s, 3H), 0.83 ppm (s, 3H). MALDI-TOF-MS: m/z calc. 3282, [M +H]+ normally distributed from 3307.99 to 3793.59.
DAS-(Phe-mPEG1000)2 (7) and DAS-(Leu-mPEG1000)2 (8).7 and 8 were made according to the methods described for 4. Compound 7 was obtained in 54.6% yield, with a purity of 98.9% according to HPLC analysis. 1H NMR (400 MHz, CDCl3) δ7.21 (m, 11H), 6.90 (m, 1H), 6.40 (m, 2H), 6.13 (d, J = 15.6 Hz, 1H), 4.82 (s, 2H), 4.80 (s, 1H), 4.62 (m, 1H), 4.55 (s, 1H), 4.25 (m, 6H), 3.4–3.9 (m, 240H), 3.38 (s, 6H), 3.11 (m, 4H), 2.52 (m, 10H), 2.03 (m, 1H), 1.82 (d, J = 12.1 Hz, 1H), 1.61 (m, 4H), 1.27 (m, 2H), 1.01 (s, 3H), 0.85 ppm (s, 3H). MALDI-TOF-MS: m/z calc. 2790, [M +H]+ normally distributed from 2507.05 to 3035.48.
Compound was obtained in 73.5% yield, with a purity of 98.3% according to HPLC analysis. 1H NMR (400 MHz, CDCl3) δ7.18 (s, 1H), 6.91 (m, 1H), 6.50 (m, 2H),6.13 (d, J = 15.6 Hz, 1H), 4.82 (s, 2H), 4.80 (s, 1H), 4.62 (m, 2H), 4.25 (m, 6H), 3.9-3.39 (m, 198H), 3.38 (s, 6H), 2.61 (m, 8H), 2.45 (d, J = 12.1 Hz, 1H), 2.34 (d, J = 10.0 Hz, 1H), 2.03 (m, 1H), 1.83 (d, J = 12.1 Hz, 1H), 1.60 (m, 10H), 1.27 (m, 2H), 0.99 (s, 3H), 0.92 (s, 6H), 0.86 ppm (s, 3H). MALDI-TOF-MS: m/z calc. 2722, [M +H]+ normally distributed from 2438.5 to 2923.0.
DAS-(Lys-mPEG1000)2 (9). To obtain 9, DAS-(Lys(Boc)-mPEG1000)2 was first synthesized. Then, Boc was removed in a 20% TFA/DCM solution at 0 °C for 30 min. Compound 9 was obtained in 10.4% yield, with a purity of 95.1% according to HPLC analysis. MALDI-TOF-MS: m/z calc. 2752, [M +H]+ normally distributed from 2445.88 to 2975.36.
To obtain DAS-(Glu-mPEG1000)2 (10), H-Glu-mPEG1000 was first prepared and then reacted with activated DAS. Compound 10 was obtained in 26.4% yield, with a purity of 96.7% according to HPLC analysis. MALDI-TOF-MS: m/z calc. 2754, [M +H]+ normally distributed from 2558.64 to 3132.24.
Evaluation of water solubility and stability
Water solubility and stability of the conjugates were investigated according to published methods.22 The stabilities of the conjugates were evaluated by determining the percentage of conjugate remaining after various treatments according to HPLC analysis.
Evaluation of anti-inflammatory activity
To evaluate the anti-inflammatory activities of the conjugates in mice, auricular swelling caused by xylene was measured. Mice were randomly divided into a control group, a DAS-K group, and conjugate-drug groups (n = 10 each group). The control group received 0.9% sodium chloride, and the other groups received intravenous drugs at 175 μmol·kg-1. At 30 min after administration, both sides of the left auricle were uniformly smeared with 20 μL of xylene. Thirty minutes later, the mice were euthanized by cervical vertebral luxation, and the ears were cut off along the auricle baseline. Auricles were taken from the same section on the right and left ears using a YLS-Q4 ear swelling ear device. The auricles were weighed, and differences between the right auricle and the left auricle were recorded. Swelling inhibition = (the mean swelling value in the control group – the mean swelling value in the drug administered group) / the mean value of swelling in the control group × 100.
Statistical analyses
All experiments evaluating activity were repeated ten times, and data were expressed as mean ± standard deviation (x±s). Statistical data were analyzed by one-way analysis of variance. Differences were considered statistically significant at P values < 0.05.
ACKNOWLEDGEMENTS
This research was supported, in part, by grants from the National Science and Technology Major Project of China (2012ZX09301003) and the National Science Foundation of China (81202465).
References
1. M. Arifullah, N. D. Namsa, M. Mandal, K. K. Chiruvella, P. Vikrama, and G. R. Gopal, Asian Pac. J. Trop. Biomed., 2013, 3, 604. CrossRef
2. W. W. Chao, Y. H. Kuo, and B. F. Lin, J. Agric. Food Chem., 2010, 58, 2505. CrossRef
3. S. Seubsasana, C. Pientong, T. Ekalaksananan, S. Thongchai, and C. Aromdee, Med. Chem., 2011, 7, 237. CrossRef
4. S. H. Liu, C. H. Lin, F. P. Liang, P. F. Chen, C. D. Kuo, M. M. Alam, B. Maiti, S. K. Hung, C. W. Chi, C. M. Sun, and S. Fu, Biochem. Pharmacol., 2014, 87, 229. CrossRef
5. M. M. Uttekar, T. Das, R. S. Pawar, B. Bhandari, V. Menon, S. K. Gupta, and S. V. Bhat, Eur. J. Med. Chem., 2012, 56, 368. CrossRef
6. G. J. Shi, Z. J. Zhang, R. Zhang, X. F. Zhang, Y. Lu, J. Yang, D. Zhang, Z. G. Zhang, X. Y. Li, and G. Ning, Naunyn-Schmiedebergs Arc. Pharmacol., 2012, 385, 69.
7. G. F. Dai, J. Zhao, Z. W. Jiang, L. P. Zhu, H. W. Xu, W. Y. Ma, X. R. Chen, R. J. Dong, W. Y. Li, and H. M. Liu, Int. Immunopharmacol., 2011, 11, 2144. CrossRef
8. C. Aromdee, Expert Opin. Ther. Pat., 2012, 22, 169; CrossRef S. Wei, Y. B. Tang, H. Hua, E. Ohkoshi, M. Goto, L. T. Wang, K. H. Lee, and Z. Xiao, Bioorg. Med. Chem. Lett., 2013, 23, 4056. CrossRef
9. X. W. Liu, Y. Fang, Q. Wang, R. Li, J. J. Tan, and R. B. Chao, Yao Xue Xue Bao, 2010, 45, 641.
10. S. Arpicco, B. Stella, O. Schiavon, P. Milla, D. Zonari, and L. Cattel, Int. J. Pharm., 2013, 454, 653. CrossRef
11. S. S. Banerjee, N. Aher, R. Patil, and J. Khandare, J. Drug Deliv., 2012, 2012, 103973.
12. F. M. Veronese and G. Pasut, Drug Discov. Today, 2005, 10, 1451. CrossRef
13. L. Webster, S. Dhar, M. Eldon, L. Masuoka, J. Lappalainen, and M. Sostek, Pain, 2013, 154, 1542. CrossRef
14. A. Patnaik, K. P. Papadopoulos, A. W. Tolcher, M. Beeram, S. Urien, L. J. Schaaf, S. Tahiri, T. Bekaii-Saab, F. M. Lokiec, and K. Rezaï, Cancer Chemother. Pharmcol., 2013, 71, 1499. CrossRef
15. I. B. Vergote, A. Garcia, J. Micha, C. Pippitt, J. Bendell, D. Spitz, N. Reed, G. Dark, P. M. Fracasso, and E. N. Ibrahim, J. Clin. Oncol., 2013, 31, 4060. CrossRef
16. J. Wu and S. F. Chen, Langmuir, 2012, 28, 2137. CrossRef
17. B. Treetharnmathurot, C. Ovartlarnporn, J. Wungsintaweekul, R. Duncan, and R. Wiwattanapatapee, Int. J. Pharm., 2008, 357, 252. CrossRef
18. S. Han, C. Kim, and D. Kwon, Polymer, 1997, 38, 317. CrossRef
19. J. M. Harris and R. B. Chess, Nat. Rev.Drug Discov., 2003, 2, 214. CrossRef
20. H. Weissbach, L. Resnick, and N. Brot, Biochim. Biophys. Acta, 2005, 1703, 203.
21. V. S. Sharov and C. Schöneich, Free Radic. Bio. Med., 2000, 29, 986; CrossRef J. Moskovitz, H. Weissbach, and N. Brot, Proc. Natl. Acad. Sci. USA, 1996, 93, 2095. CrossRef
22. Chinese Pharmacopoeia Commission, ‘Pharmacoeia of the People’s Republic of China’, China Medical Science Press, Beijing, 2010, 2010 edition, Volume II, pXIV; Chinese Pharmacopoeia Commission, ‘Pharmacoeia of the People’s Republic of China’, China Medical Science Press, Beijing, 2010, 2010 edition, Volume II, Appendix XIX C