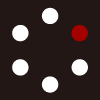
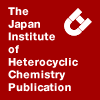
HETEROCYCLES
An International Journal for Reviews and Communications in Heterocyclic ChemistryWeb Edition ISSN: 1881-0942
Published online by The Japan Institute of Heterocyclic Chemistry
e-Journal
Full Text HTML
Received, 23rd December, 2014, Accepted, 28th January, 2015, Published online, 6th February, 2015.
DOI: 10.3987/REV-14-816
■ Directed Lithiation and Substitution of Pyridine Derivatives
Gamal A. El-Hiti,* Keith Smith,* and Amany S. Hegazy
Department of Optometry, College of Applied Medical Sciences, King Saud University, P.O. Box 10219, Riyadh 11433, Saudi Arabia
Abstract
Directed lithiation of substituted pyridines containing a directing metalating group (DMG) with a lithium reagent in anhydrous solvent at low temperature gives the corresponding lithium intermediates in-situ, which on reactions with electrophiles produce the corresponding substituted derivatives. The method is simple, general, convenient and often high yielding.Contents
1. Introduction
2. Directed lithiation and substitution of 2-substituted pyridines
3. Directed lithiation and substitution of 3-substituted pyridines
4. Directed lithiation and substitution of 4-substituted pyridines
5. Conclusion
6. Acknowledgements
7. References
1. INTRODUCTION
Pyridine derivatives are interesting compounds1 that have a broad range of biological activities.2,3 Therefore, it is not surprising that a range of substituted pyridine derivatives continue to be synthesised. One useful approach for the synthesis of substituted pyridines involves lithiation followed by treatment with an appropriate electrophile.4−7 However, reactions of the parent heterocycle, pyridine (1), with organolithium reagents (RLi) usually result in addition to the C=N bond of the ring. For example, reaction of pyridine with PhLi gives 1-lithio-2-phenyl-1,2-dihydropyridine (2; R = Ph; Scheme 1).4,5 When the intermediate 2 (R = Ph) was isolated and allowed to react with iodomethane in tetrahydrofuran (THF) at 0 °C it gave 5-methyl-2-phenylpyridine (3; E = Me; R = Ph) in 34% yield5 due to electrophilic attack of iodomethaneon 2 (R = Ph) followed by aromatization. Similar results were obtained when other electrophiles (iodobenzene, benzyl chloride, bromine) were used to give the corresponding 2,5-disubstituted pyridines 3 (R = Ph).5
When the reactions described in Scheme 1 were carried out without isolation of the lithium intermediates 2, 2,5-disubstituted pyridines 3 were obtained in lower yields along with other substituted products.5−7
The situation is somewhat different for many substituted pyridines. In such cases, organolithium intermediates can often be generated cleanly and used for the efficient production of ortho-disubstituted aromatic products in a simple and convenient process involving directed metalation.8–21 The reactions of the substituted pyridines with lithium reagents usually take place at low temperatures in anhydrous diethyl ether (Et2O) or THF. When addition of lithium reagent to the C=N bond of the pyridine ring remains a problem, it can often be avoided by use of a hindered lithiating reagent such as lithium diisopropylamide (LDA) or lithium 2,2,6,6-tetramethylpiperidide (LTMP) at low temperature. If all such variations are unsuccessful, an alternative approach is to use bromine-lithium exchange or some similar exchange reaction rather than direct lithiation, but this requires prior synthesis of the appropriately substituted derivative and this is beyond the scope of this review, which concentrates on direct lithiation (i.e. hydrogen-lithium exchange).
Directed lithiation of pyridine derivatives 4 possessing a directing metalating group (DMG) involves removal of a proton from a site ortho to the DMG by means of a lithiating reagent, which gives the ortho-lithium species 6 through initial coordination of the base to the DMG (5, Scheme 2). Reaction of 6 with electrophiles gives the corresponding substituted pyridines 7.16 The complexation between the lithium reagent and the DMG prior to lithiation brings the lithium reagent into closer proximity to the proton in the adjacent position, which is then selectively removed.22,23 The DMG should be a poor electrophilic site for attack by the lithium reagent and at the same time a good coordinating site for the lithium reagent. Strong DMGs that encourage ortho-lithiation include SO2NR2, NHCOR, CONR2, CSNHR, CONHR, OCONR2, CO2R, CH2NHR, OCH2OMe. Moderate DMGs include OR, NR2, SR, CF3 and F, while weak DMGs include CH2OH and CH(OR)2.24
2. DIRECTED LITHIATION AND SUBSTITUTION OF 2-SUBSTITUTED PYRIDINES
Directed lithiation of pyridines containing a DMG at the C-2 (α) position takes place at the 3-position to provide the corresponding lithium intermediates, which on further reactions with electrophiles give the corresponding substituted derivatives.25–47 For example, C-3 lithiation of 2-(pivaloylamino)pyridines (8, Scheme 3) has been achieved with n-BuLi (2.2 mole equivalents) in anhydrous THF at 0 or –50 °C to give monolithium intermediates 9 and then dilithium reagents 10, which on reactions with electrophiles gave the corresponding substituted pyridines 11 (Scheme 3) in 40–94% yields (Table 1).25,26
Carbonylation of 10 (R = H) with carbon monoxide at 0 °C gave 3-tert-butyl-7-azadioxindole (12; Figure 1) in 62% yield following cyclization and rearrangement of the initial carbon monoxide adduct.20
Lithiation of 6-fluoro-2-(pivaloylamino)pyridine with n-BuLi (two mole equivalents) at –78 to 0 °C in THF followed by reaction with dimethyl disulfide gave a mixture of products due to substitution at the 3-position (45%) and the 5-position (30%).25 However, when t-BuLi was used at –78 °C in Et2O, substitution took place exclusively at the 3-position to give 6-fluoro-3-methylthio-2-(pivaloylamino)pyridine in 70% yield.25
Lithiation of 2-(benzoylamino)-4-chloropyridine (13) with two mole equivalents of LDA in THF at –78 °C produced the 3-lithio reagent 14, which on reactions with electrophiles gave the corresponding 2,3,4-trisubstituted pyridines (15; Scheme 4 and Table 2).27 Lithiation of 13 was also successful with n-BuLi (2.1 mole equivalents) at –20 to –78 °C.28
Directed lithiation of N,N-dialkylpicolinamides 16 (Scheme 5) with 1.1 mole equivalents of sec-butyllithium (sec-BuLi) or LDA in THF or Et2O at –78 °C, followed by reactions with electrophiles, gave the corresponding 2,3-disubstituted derivatives 18 (Table 3) via lithium intermediates 17.29–31
Directed lithiation and substitution of 2-(substituted sulfinyl)pyridines 19 was successful with LDA (one mole equivalent) in THF at –78 °C to give the corresponding substituted derivatives 21 (Scheme 6) in 20–93% yields (Table 4).32–35
Directed lithiation of pyridine-2-carboxylic acid (22) has been achieved by the use of n-BuLi (one mole equivalent) at –75 or –50 °C followed by the addition of LTMP (three mole equivalents) at 0 °C in THF (Scheme 7; Table 5).36,37
Lithiation of 2-halopyridines has received much attention.38–44 For example, lithiation of 2-chloropyridine (25) with three mole equivalents of LTMP at –78 °C in THF followed by reaction with DCl/D2O gave2,3-disubstituted derivative 27 (E = D; Scheme 8) in 70% yield, indicating that lithium reagent 26 was formed in-situ.38 Lithiation was found to be general with the use of 2.0 mole equivalents of n-BuLi (Table 6).39 Moreover, directed lithiation of 25 has been achieved with one mole equivalent of LDA at –78 °C in THF.40 By contrast, lithiation of 25 with n-BuLi–LiDMAE (lithium 2-dimethylaminoethoxide; 3.0 mole equivalents) at –78 °C in hexane followed by reaction with representative electrophiles gave the corresponding 2,6-disubstituted derivatives in 65−98% yields.38 It seems that such a lithium reagent diverts lithiation to the 6-position as a result of a combination of high basicity, a low propensity for coordination to the DMG, and the steric bulk of the reagent.
Lithiation of 2-methoxypyridne (28; R = OMe) with LTMP (3.2 mole equivalents) at –78 °C in THF followed by reaction with DCl/D2O gave the corresponding 3-deuteriated derivative in 92% yield.38 However, the use of n-BuLi–LiDMAE (3 mole equivalents) in hexane at –78 °C followed by reaction with DCl/D2O again diverted the reaction to the 6-deuteriated derivative 30 (Scheme 9: R = OMe, E = D) in 68% yield.38,45–47 The latter reaction was found to be general with other electrophiles.38,45–47 The effect of the amount of n-BuLi–LiDMAE on the lithiation of 2-methoxypyridne (28; R = OMe) is interesting. With 4 equivalents of n-BuLi–LiDMAE at 0 °C in hexane the 6-substituted derivatives 30 (Scheme 9) were obtained in 30–71% yields along with 2-butyl-2,5-dihydro-6-methoxypyridine (14–64%), formed by addition of n-BuLi to the imine bond of the pyridine nucleus.45
When two mole equivalents of n-BuLi–LiDMAE were used in the presence of lithium bromide as an additive, the 2,6-disubstituted derivatives 30 were obtained in better yields (41–88%, Table 7) along with 2-butyl-2,5-dihydro-6-methoxypyridine (5–53%).45 Under similar conditions, lithiation of 2-(methylthio)- and 2-(dimethylamino)- pyridines followed by reactions with electrophiles provided 30 (Scheme 9; R = SMe and R = NMe2) in 44–80% yields (Table 7).45
3. DIRECTED LITHIATION AND SUBSTITUTION OF 3-SUBSTITUTED PYRIDINES
In contrast to 2-substituted pyridines, 3-substituted pyridines have two possible sites for DMG-encouraged lithiation, namely the 2- and 4-positions. Lithiation at the 2-position could benefit from the stabilisation effect of the pyridine nitrogen atom as well as the DMG effect, while lithiation at the 4-position would benefit only from the DMG effect (Figure 2). Therefore, a mixture of products might be expected as a result of such lithiation and the 2-position might be expected to be favored.
In the case of lithiation of 3-methoxypyridine (31) it is indeed the case that substitution mainly takes place at the 2-position to give the corresponding 2,3-disubstituted pyridines 33 (Scheme 10; Table 8).48,49 The yields of 2-substituted products are dependent on the reaction conditions and the type of lithium reagent used, but the formation of lithium intermediate 32 is clearly favored.
However, the presence of an additional 2-methoxy group in 2,3-dimethoxypyridine (34) causes lithiation with n-BuLi (2.2 mole equivalents) at 0 °C in THF to be diverted to the 4-position to give lithium intermediate 35, which on reactions with electrophiles gave 2,3,4-trisubstituted pyridines 36 (Scheme 11) in 60–99% yields (Table 9).50
The site of lithiation for 3-chloropyridine (37) is much more variable and is highly influenced by the type of lithium reagent, temperature, and/or solvent.40,41,51−57 For example, lithiation of 37 with n-BuLi/TMEDA (one mole equivalent) in diethyl ether at –60 °C for 1 h followed by reaction with trimethylsilyl chloride gave a mixture of products due to lithiation and substitution at the 2- and 4-positions in 60 and 9% yields, respectively.55,56 However, use of n-BuLi/18-crown-8 (one mole equivalent) in diethyl ether at –60 °C for 1 h followed by reaction with trimethylsilyl chloride gave the corresponding 2,3- and 3,4-disubstituted products in 20 and 75% yields, respectively,55,56 while lithiation of 37 with n-BuLi/LiDMAE (three mole equivalents) in hexane at –60 °C for 1 h followed by reactions with several electrophiles gave exclusively the corresponding 2,3-disubstituted pyridines 39 (Scheme 12) in 47–83% yields (Table 10).54
Predominant lithiation at the 2-position with n-BuLi/LiDMAE presumably reflects the greater stability of the 2-lithio reagent 38, which may be further enhanced via complexation with an extra molecule of the lithiating reagent (Figure 3).54
On the other hand, lithiation of 3-halopyridines 40 with LDA in THF at –78 °C took place predominantly at the 4-position (Scheme 13, Table 11).30,40,54
For the 3-fluoropyridine case, the presence of an additional 5-fluoro substituent or a (2-(tert-butylcarbonylamino)phenyl) group at the 2-position and use of n-BuLi in THF or Et2O at –78 °C also results in lithiation of 3-fluoropyridines 43 at the 4-position (Scheme 14, Table 12).51,52
Similarly, and despite the presumed additional stability of the 2-lithio species, directed lithiation of many 3-substituted pyridines 46 takes place predominately at C-4 to afford the corresponding lithium intermediates 47 (Scheme 15), reactions of which with electrophiles give the corresponding 3,4-disubstituted pyridines 48.25,30,31,35–38,58–68 It seems likely, therefore, that in these cases the kinetic lithiation products are being generated and trapped before they rearrange to the more stable 2-lithio species.
For example, lithiation of N-tert-butylpyridine-3-sulfonamide (49) was achieved with LDA (3.0 mole equivalents) at –70 °C in Et2O to give lithium reagent 50.35 Reactions of 50 with benzophenone and carbon dioxide as representative electrophiles gave 51, in both cases in 80% yield (Scheme 16).35
Directed lithiation of N-(pyridin-3-ylmethyl)pivalamide (52; R = t-Bu), tert-butyl N-(pyridin-3-ylmethyl)carbamate (52; R = t-BuO) and N'-(pyridin-3-ylmethyl)-N,N-dimethylurea (52; R = NMe2) with 3.3 mole equivalents of t-BuLi at −78 °C in THF gave the corresponding 4-lithio intermediates 53 (Scheme 17).62 Reactions of 53 with several electrophiles afforded 54 in high yields (Table 13). By contrast, lithiation of 52 with LDA at −20 to 0 °C in THF followed by reactions with representative electrophiles gave side-chain substituted products in 52−75% yields.62
Directed lithiation of O-pyridin-3-yl diethylcarbamothioate (55; Scheme 18) with LTMP (1.1 mole equivalents) in THF at –78 °C followed by reactions with electrophiles gave 57 (Table 14) via lithium intermediate 56.68
Directed lithiation of nicotinic acids 58 (Scheme 19) with LTMP (two mole equivalents) or a mixture of n-BuLi (one mole equivalent) and LTMP (three mole equivalents) at –75 to –50 °C in THF followed by reactions with electrophiles gave 60 (Table 15).36,37 This product is still the major one isolated even when there is a Cl or Br substituent elsewhere in the molecule. Lithiation of 58 (R = 5-Br) followed by reaction with benzaldehyde under similar conditions and then treatment with acetic acid gave the corresponding lactone via cyclization of the initially produced substituted derivative.36,37
Similarly, directed lithiation of 3-(2-methyloxetan-2-yl)pyridines 61 with n-BuLi (1.4 mole equivalents) and TMEDA (3.0 mole equivalents) in Et2O at –78 °C gave the corresponding 4-lithio intermediates 62 even with a chloro or methoxy substituent in the 6-position (Scheme 20).69 Reactions of 62 with various electrophiles gave substituted pyridines 63 in 50–95% yields (Table 16).69
In some other cases the situation is more confused, because only products of 4-substitution have been reported, but in many examples there is already a substituent at the 2-position, so regioselectivity is probably not an issue. Sometimes when there is no 2-substituent, the reported yields of 4-substituted products may be relatively low, so it is not clear whether there is actual selectivity for lithiation at the 4-position or whether 2-substituted product was formed, but removed during isolation. For example, lithiation of N-(pyridin-3-yl)pivalamides (64; R = t-Bu) and tert-butyl pyridin-3-ylcarbamates (64; R = t-BuO) with two equivalents of n-BuLi at –78 to –10 °C in a mixture of Et2O and TMEDA, or with t-BuLi or n-BuLi in THF at –78 °C (Scheme 21) gave the yields of 4-substituted products 66 reported in Table 17.25,58–61
Similarly, lithiation of N,N-dialkylnicotinamides 67 (Scheme 22) with 1.5–3.0 mole equivalents of various lithiating reagents in THF or Et2O at low temperature gave only modest yields of 4-substituted products unless there was a further substituent at the 2-position (Table 18).31,63–66
This is also the situation for lithiation of enantiopure 3-(4-tolylsulfinyl)pyridines 70 (Scheme 23) with LDA (3.2 mole equivalents) in THF at –75 °C to give the corresponding lithium reagents 71.67 Reactions of 71 with aldehydes gave 72 (Table 19) as mixtures of diastereoisomers,67 but yields were not good in cases where there was no 2-substituent in the substrate. The diastereoselectivity was high (>99%) with somewhat hindered aldehydes (benzaldehyde and 2,2-dimethylpropanal), but low (0−38%) with unhindered ones (acetaldehyde and propionaldehyde). The new stereogenic center in the major diastereoisomer had the opposite designation of configuration to that of the sulfoxide unit.
Reactions of methoxypyridinecarboxaldehydes with lithium dialkylamides gave the corresponding α-aminoalkoxide addition products, which could be lithiated in-situ with an alkyllithium.70 Reactions of the lithiated species with iodomethane gave methylated derivatives in 65−82% yields. The site of lithiation was found to be next to either the methoxy or carboxaldehyde group. Also, it was found to be dependent on the type of lithium dialkylamides used.70 For example, lithiation of 6-methoxy-3-pyridinecarboxaldehyde with lithium N,N',N'-trimethylethylenediamide and n-BuLi followed by reaction with iodomethane gave a mixture of 3- and 5-methylated derivatives in 65% yield in a 97 to 3 ratio, respectively.70 On the other hand, when the reaction was carried out using lithium N-methylpiperazide, t-BuLi and TMEDA a mixture of 3- and 5-methylated derivatives was produced in 70% yield in a 7 to 93 ratio, respectively.70
4. DIRECTED LITHIATION OF 4-SUBSTITUTED PYRIDINES
Lithiation of 4-substituted pyridines 73 takes place at C-3 to produce the 3-lithio intermediates 74. Reactions of 74 with electrophiles give the corresponding 3,4-disubstituted pyridines 75 (Scheme 24).25,28,30,31,36,37,49,53,71,72
Directed lithiation of 4-(pivaloylamino)pyridine (76) with n-BuLi (two mole equivalents) at 0 or –78 °C in THF followed by reactions with electrophiles gave 3,4-disubstituted pyridines 78 in 54–92% yields (Scheme 25; Table 20).25,72 Carbonylation of 77 at 0 °C gave 3-tert-butyl-6-azadioxindole (79; Figure 4) in 65% yield via cyclization and rearrangement of the initial carbon monoxide adduct.20
Directed lithiation of N,N-diisopropylisonicotinamide (80) was successful with LDA in Et2O at –78 °C to give lithium reagent 81, which was reacted with electrophiles to give the corresponding substituted derivatives 82 in modest yields (Scheme 26; Table 21).30,31
Directed lithiation of 2-chloro-N-phenylisonicotinamide (83) occurs at position 3 with n-BuLi (2.1 mole equivalents) in THF at –78 to 0 °C (Scheme 27).28 A dimethylated product was obtained in 83% yield when lithium reagent 84 was reacted with excess iodomethane (3.0 mole equivalents) due to methylation at both nitrogen and C-3.28 The simple adducts obtained from use of aldehydes as electrophiles were not isolated, but were cyclized under acidic conditions to give the corresponding lactones.28
Directed lithiation of isonicotinic acid (86) was achieved with a mixture of n-BuLi (one mole equivalent) and LTMP (three mole equivalents) in THF at –75 to –25 °C (Scheme 28; Table 22).36,37
Directed lithiation of 4-methoxypyridine (89) has been achieved with phenyllithium (PhLi; 2.2 mole equivalents) in THF at 0 °C and subsequent reaction with electrophiles gave 91 (Scheme 29) in 67–90% yields (Table 23).71 Lithiation of 3,4-dimethoxypyridine with n-BuLi (2.2 mole equivalents) at –78 to 0 °C in THF took place at the 2-position.71
5. CONCLUSION
Directed lithiation of various DMG-substituted pyridines by lithium reagents at low temperatures followed by reactions with electrophiles often produces the corresponding pyridines substituted adjacent to the DMG. Directed lithiation of pyridines containing a DMG at the C-2 or C-4 position takes place at the 3-position, while under similar reaction conditions directed lithiation often takes place at the C-4 position for 3-substituted pyridines. The process is efficient for the production of various substituted pyridine derivatives in a single step. Such derivatives might be difficult to prepare by other means.
6. ACKNOWLEDGEMENTS
The authors extend their appreciation to the Deanship of Scientific Research at King Saud University for its funding for this research through the research group project RGP-VPP-239.
References
1. J. A. Joule and K. Mills, Heterocyclic Chemistry, 4th ed., England: Blackwell Science Publishers, 2000.
2. M. de Candia, F. Fiorella, G. Lopopolo, A. Carotti, M. R. Romano, M. D. Lograno, S. Martel, P.-A. Carrupt, B. D. Belviso, R. Caliandro, and C. Altomare, J. Med. Chem., 2013, 56, 8696. CrossRef
3. S. A. Thorat, D. W. Kang, H. Ryu, M. S. Kim, H. S. Kim, J. Ann, T. Ha, S.-E. Kim, K. Son, S. Choi, P. M. Blumberg, R. Frank, G. Bahrenberg, K. Schiene, T. Christoph, and J. Lee, Eur. J. Med. Chem., 2013, 64, 589. CrossRef
4. C. S. Giam and J. L. Stout, J. Chem. Soc. D: Chem. Commun., 1969, 142. CrossRef
5. C. S. Giam and J. L. Stout, J. Chem. Soc. D: Chem. Commun., 1970, 478. CrossRef
6. R. Levine and W. M. Kadunce, J. Chem. Soc. D: Chem. Commun., 1970, 921. CrossRef
7. C. S. Giam, E. E. Knaus, and F. M. Pasutto J. Org. Chem., 1974, 39, 3565. CrossRef
8. G. A. El-Hiti, K. Smith, A. S. Hegazy, M. B. Alshammari, and A. Masmali, ARKIVOC, 2015, iv, 19.
9. K. Smith, G. A. El-Hiti, and M. B. Alshammari, Synthesis, 2014, 46, 394; CrossRef K. Smith, G. A. El-Hiti, and M. B. Alshammari, Synlett, 2013, 24, 117. CrossRef
10. L. I. Beleńkii and Yu. B. Evdokimenkova, Adv. Heterocycl. Chem., 2014, 111, 147. CrossRef
11. J. Board, J. L. Cosman, T. Rantanen, S. R. Singh, and V. Snieckus, Platinum Metals Rev., 2013, 57, 234. CrossRef
12. Stereochemical Aspects of Organolithium Compounds: Topics in Stereochemistry; ed. by J. S. Siegel, Vol. 26, 2010.
13. R. Chinchilla, C. Nájera, and M. Yus, Chem. Rev., 2004, 104, 2667. CrossRef
14. K. Smith and G. A. El-Hiti, Curr. Org. Synth., 2004, 1, 253; CrossRef K. Smith, G. A. El-Hiti, M. F. Abdel-Megeed, and M. A. Abdo, J. Org. Chem., 1996, 61, 647. CrossRef
15. R. J. Anderson, J. B. Hill, and J. C. Morris, J. Org. Chem. 2005, 70, 6204. CrossRef
16. J. Clayden, Organolithiums: Selectivity for Synthesis, Pergamon: Oxford, 2002.
17. M. Schlosser, Organometallics in Synthesis, 2nd ed.; Wiley: Chichester, 2002, pp. 1–352.
18. E. J.-G. Anctil and V. Snieckus, J. Organomet. Chem., 2002, 653, 150. CrossRef
19. F. Mongin and G. Queguiner, Tetrahedron, 2001, 57, 4059. CrossRef
20. K. Smith, G. A. El-Hiti, G. J. Pritchard, and A. Hamilton, J. Chem. Soc., Perkin Trans. 1, 1999, 2299. CrossRef
21. V. Snieckus, Chem. Rev., 1990, 90, 879. CrossRef
22. J. D. Roberts and D. Y. Curtin, J. Am. Chem. Soc., 1946, 68, 1658. CrossRef
23. G. Quéguiner, F. Marsais, V. Snieckus, and J. Epsztajn, Adv. Heterocycl. Chem., 1991, 52, 187. CrossRef
24. A. I. Meyers and W. B. Avila, Tetrahedron Lett., 1980, 21, 3335. CrossRef
25. J. A. Turner, J. Org. Chem., 1983, 48, 3401. CrossRef
26. K. Smith, D. Anderson, and I. Matthews, Sulfur Lett., 1995, 18, 79.
27. M. V. N. de Souza and R. H. Dodd, Heterocycles, 1998, 47, 811. CrossRef
28. J. Epsztajn, A. Bieniek, and J. A. Kowalska, Tetrahedron, 1991, 47, 1697. CrossRef
29. M. Villacampa, E. de la Cuesta, and C. Avendaño, Tetrahedron, 1995, 51, 1259. CrossRef
30. J. Epsztajn, Z. Berski, J. Z. Brzeziński, and A. Joźwiak, Tetrahedron Lett., 1980, 21, 4739. CrossRef
31. J. Epsztajn, A. Bieniek, J. Z. Brzeziński, and A. Joźwiak, Tetrahedron Lett., 1983, 24, 4735. CrossRef
32. C. Quesnelle, T. Iihama, T. Aubert, H. Perrier, and V. Snieckus, Tetrahedron Lett., 1992, 33, 2625. CrossRef
33. T. Shibutani, H. Fujihara, and N. Furukawa, Tetrahedron Lett., 1991, 32, 2943. CrossRef
34. T. Shibutani, H. Fujihara, and N. Furukawa, Tetrahedron Lett., 1991, 32, 2947. CrossRef
35. B. I. Alo, O. B. Familoni, F. Marsais, and G. Quéguiner, J. Heterocycl. Chem., 1992, 29, 61. CrossRef
36. F. Mongin, F. Trécourt, and G. Quéguiner, Tetrahedron Lett., 1999, 40, 5483. CrossRef
37. J. Lazaar, A.-S. Rebstock, F. Mongin, A. Godard, F. Trécourt, F. Marsais, and G. Quéguiner, Tetrahedron, 2002, 58, 6723. CrossRef
38. P. Gros, S. Choppin, J. Mathieu, and Y. Fort, J. Org. Chem., 2002, 67, 234; CrossRef P. Gros and Y. Fort. Eur. J. Org. Chem., 2002, 3375. CrossRef
39. M. Mallet, J. Organomet. Chem., 1991, 406, 49. CrossRef
40. G. W. Gribble and M. G. Saulnier, Heterocycles, 1993, 35, 151. CrossRef
41. P. Rocca, C. Cochennec, F. Marsais, L. Thomas-dit-Dumont, M. Mallet, A. Godard, and G. Quéguiner, J. Org. Chem., 1993, 58, 7832. CrossRef
42. G.-Q. Shi, S. Takagishi, and M. Schlosser, Tetrahedron, 1994, 50, 1129. CrossRef
43. F. Mongin, A. Tognini, F. Cottet, and M. Schlosser, Tetrahedron Lett., 1998, 39, 1749. CrossRef
44. R. Radinov, C. Chanev, and M. Haimova, J. Org. Chem., 1991, 56, 4793. CrossRef
45. P. Gros, Y. Fort, and P. Caubère, J. Chem. Soc., Perkin Trans. 1, 1997, 3071. CrossRef
46. S. Choppin, P. Gros, and Y. Fort, Org. Lett., 2000, 2, 803. CrossRef
47. H. K. Khartabil, P. C. Gros, Y. Fort, and M. F. Ruiz-López, J. Am. Chem. Soc., 2010, 132, 2410. CrossRef
48. S. E. Watson and A. Markovich, Heterocycles, 1998, 48, 2149. CrossRef
49. A. Numata, Y. Kondo, and T. Sakamoto, Synthesis, 1999, 306. CrossRef
50. F. Trécourt, M. Mallet, O. Mongin, and G. Quéguiner, J. Org. Chem., 1994, 59, 6173. CrossRef
51. R. D. Chambers, C. W. Hall, J. Hutchinson, and R. W. Millar, J. Chem. Soc., Perkin Trans. 1, 1998, 1705. CrossRef
52. E. Arzel, P. Rocca, F. Marsais, A. Godard, and G. Quéguiner, J. Heterocycl. Chem., 1997, 34, 1205. CrossRef
53. E. Arzel, P. Rocca, F. Marsais, A. Godard, and G. Quéguiner, Heterocycles, 1999, 50, 215. CrossRef
54. S. Choppin, P. Gros, and Y. Fort, Eur. J. Org. Chem., 2001, 603. CrossRef
55. F. Marsais, P. Bréant, A. Ginguéne, and G. Quéguiner, J. Organomet. Chem., 1981, 216, 139. CrossRef
56. F. Trécourt, F. Marsais, T. Güngör, and G. Quéguiner, J. Chem. Soc., Perkin Trans. 1, 1990, 2409. CrossRef
57. G. W. Gribble and M. G. Saulnier, Tetrahedron Lett., 1980, 21, 4137. CrossRef
58. T. A. Kelly and U. R. Patel, J. Org. Chem., 1995, 60, 1875. CrossRef
59. A. Godard, P. Rocca, V. Pomel, L. Thomas-dit-Dumont, J.-C. Rovera, J.-F. Thaburet, F. Marsais, and G. Quéguiner, J. Organomet. Chem., 1996, 517, 25. CrossRef
60. V. Pomel, J.-C. Rovera, A. Godard, F. Marsais, and G. Quéguiner, J. Heterocycl. Chem., 1996, 33, 1995. CrossRef
61. T. Güngör, F. Marsais, and G. Quéguiner, Synthesis, 1982, 499. CrossRef
62. K. Smith, G. A. El-Hiti, M. B. Alshammari, and A. Fekri, Synthesis, 2013, 45, 3426. CrossRef
63. X. Wang and V. Snieckus, Tetrahedron Lett., 1991, 32, 4883. CrossRef
64. J.-R. Dormoy and A. Heymes, Tetrahedron, 1993, 49, 2885. CrossRef
65. F. Guillier, F. Nivoliers, J. Bourguignon, G. Dupas, F. Marsais, A. Godard, and G. Quéguiner, Tetrahedron Lett., 1992, 33, 7355. CrossRef
66. F. Guillier, F. Nivoliers, A. Godard, F. Marsais, G. Quéguiner, M. A. Siddiqui, and V. Snieckus, J. Org. Chem., 1995, 60, 292. CrossRef
67. P. Pollet, A. Turck, N. Plé, and G. Quéguiner, J. Org. Chem., 1999, 64, 4512. CrossRef
68. F. Beaulieu and V. Snieckus, Synthesis, 1992, 112. CrossRef
69. G. Rouquet, D. C. Blakemore, and S. V. Ley, Chem. Commun., 2014, 50, 8908. CrossRef
70. D. L. Comins and M. O. Killpack, J. Org. Chem., 1990, 55, 69. CrossRef
71. F. Trécourt, M. Mallet, O. Mongin, B. Gervais, and G. Quéguiner, Tetrahedron, 1993, 49, 8373. CrossRef
72. K. Smith, C. M. Lindsay, I. K. Morris, I. Matthews, and G. J. Pritchard, Sulfur Lett., 1994, 17, 197.