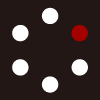
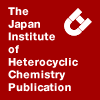
HETEROCYCLES
An International Journal for Reviews and Communications in Heterocyclic ChemistryWeb Edition ISSN: 1881-0942
Published online by The Japan Institute of Heterocyclic Chemistry
e-Journal
Full Text HTML
Received, 26th December, 2014, Accepted, 26th January, 2015, Published online, 3rd February, 2015.
DOI: 10.3987/COM-14-13162
■ Decarboxylative Halogenation of Indolecarboxylic Acids Using Hypervalent Iodine(III) Reagent and Its Application to the Synthesis of Polybromoindoles
Hiromi Hamamoto,* Hideaki Umemoto, Misako Umemoto, Chiaki Ohta, Emi Fujita, Akira Nakamura, Tomohiro Maegawa, and Yasuyoshi Miki*
Department of Applied Biological Chemistry, Faculty of Agriculture, Meijo University, 1-501 Shiogamaguchi, Tempaku-ku, Nagoya, Japan
Abstract
Hypervalent iodine mediated decarboxylative halogenation of indoledicarboxylic acid derivatives was studied. The treatment of 1-methylindole-2,3-dicarboxylic acid with phenyliodine diacetate (PIDA) in the presence of lithium bromide gave 1-methyl-3,3-bromooxindole. However, the reaction of 1-(phenylsulfonyl)indole-2,3-dicarboxylic acid with PIDA in the presence of lithium bromide afforded 2,3-dibromo-1-(phenylsulfonyl)indole. In a similar manner, the 2,3-dichloro- and 2,3-diiodoindole derivatives could be obtained by the reaction of the indole-2,3-dicarboxylic acids with PIDA in the presence of lithium chloride and iodide. This method was optimized to the synthesis of polybromoindole alkaloids.INTRODUCTION
The decarboxylative halogenation that can provide halogenated organic compounds from carboxylic acids by simple chemical transformation has become an increasingly important and attractive tool in organic synthesis.1,2 The Hunsdiecker reaction, the reaction of dry silver (I) salts of aliphatic carboxylic acids with bromine to give organic bromide, is an example of classical decarboxylative halogenation reaction.1 Because of the difficult preparation of anhydrous silver carboxylates, several Hunsdiecker-type reaction methods have thereafter been developed to simplify the procedure by using the various reagent conditions such as thallium(I) or mercury(II) salts. To avoid the use of highly toxic reagents, Suárez introduced the decarboxylative iodination using hypervalent iodine reagent, phenyliodine diacetate (PIDA), and iodine under UV photolysis.3-5 Although such metal salt free approaches constitute an important method in chemistry and several groups also report the devising methodology of hypervalent iodine mediated decarboxylative halogenations, their utility is limited for the reaction of aliphatic and α,β-unsaturated carboxylic acids in many cases.6
Halogenated indole derivatives are promising sources of new biologically active molecules.7 Several halogenated indole derivatives have been isolated as the secondary metabolites of marine organisms tunicates (Figure 1).8-11 Some of them are known to have a variety of actions including antibiotic and anticancer activities. Alternatively, halogenated indole derivatives could be attractive synthetic sources for conversion to other indole alkaloid derivatives.
In the course of our synthetic studies on indoles and related compounds, we have shown the utilities of indole-2,3-carboxylic acid derivatives and applied to the synthesis of indole alkaloids such as murrayaquinone-A, ellipticine, olivacine, caulersin and cryptosanguinolentine.12-19 There is no approach to halogenated indole derivatives via decarboxylative halogenations except for the synthesis of the 2,3-diiodoindoles by the decarboxylative iodination of indole-2-carboxylic acids, but in the case of indole-2-carboxylic acids without having an electron-donating substituent, the yields of the 2,3-diiodoindoles are low.20
Previously, we reported the synthesis of the 2,3-dibromoindoles by the decarboxylative bromination of indole-2,3-dicarboxylic acids by using Oxone® and lithium bromide, but 2,3-dichloro- or 2,3-diiodoindoles were not obtained.21 Very recently, we found the novel ability of hypervalent iodine (III)-LiX combination in decarboxylative halogenation.22-24 In this paper, we provide a full account of our studies on the hypervalent iodine mediated decarboxylative halogenation of indolecarboxylic acid derivatives.22 In addition, an effective utilization of this system for the synthesis of polybromoindole alkaloids was also described.
RESULTS AND DISCUSSION
As our initial approach, the decarboxylative halogenation of 1-(phenylsulfonyl)indole-2,3-dicarboxylic acid (1)25 was studied. The reaction using 1-3 equivalents of PhI(OAc)226 in the presence of the same equivalents of lithium bromide in THF gave a 3-bromoindole-2-carboxylic acid (3a) or a mixture of 3a and 2,3-dibromoindole (5a),27 but the treatment of 1 with PhI(OAc)2 (4 equivalents) afforded 5a in 86% yield (Table 1, entries 1-3). However, when the reaction of 1 with PhI(OAc)2 was carried out using potassium bromide instead of lithium bromide, 5 equivalents of PhI(OAc)2 or CH2Cl2 as the solvent, the yields of 5a were relative low (33–72%, entries 4-6). The catalytic hypervalent iodine oxidation condition using m-CPBA (m-chloroperoxybenzoic acid)28 was ineffective for this reaction (entry 7). To extend our procedure to the decarboxylative iodination and chlorination, the PhI(OAc)2 mediated reaction was carried out using lithium chloride or iodide, and dichloro- or diiodoindoles 529 was successfully obtained in good yield, where other reagents such as Oxone® and CAN (Cerium (IV) ammonium nitrate) 21 were ineffective for these reactions (entries 8-13).
Next, we examined the decarboxylative halogenation of 1-methylindole-2,3-dicarboxylic acid (2).30 The reaction using 1 equivalent or 2 equivalents of PhI(OAc)2 in the presence of the same equivalents of lithium bromide in THF gave 3-bromoindole-2-carboxylic acid (4a) in 57% or 74% yields, respectively (entries 14, 15). When the reaction of 2 with 3 equivalents of PhI(OAc)2 was examined, the corresponding 2,3-dibromoindole (6a), the desired product, was not isolated and 3,3-dibromo-1-methyloxindole (8a)31 was obtained as the sole product in 78% yield (entry 16). In addition, the reaction of 2 with 3 equivalents of PhI(OAc)2 in CH2Cl2 instead of THF also afforded 8a in slightly lower yield (73%, entry 17). As for the decarboxylative iodination and chlorination of 2, the PhI(OAc)2 mediated reaction in the presence of lithium chloride gave 3,3-dichloro-1-methyloxindole (8b)32 in 77 % yield, and 2,3-diiodo-1-methylindole (6c)33 was isolated in 87% yield in the presence of lithium iodide (entries 18, 21). In each case, other reagents such as Oxone® and CAN were ineffective for these reactions (entries 19, 20, 22, 23).
One possible explanation for the formation of 3,3-dihalo-1-methyloxindole (8a and 8b) is envisaged as shown in Scheme 1. The halogenation of 2,3-dihalo-1-methylindole (6), which would obtained after the second Hunsdiecker-type decarboxylative halogenation of 4, lead to intermediate 9. Treatment of 9 with water by workup provides 8, where 8c was not obtained, probably due to steric hindrance. The absence of 3,3-dihalo-1-(phenylsulfonyl)oxindole on the reaction with 1, possessing electronwithdrawing N-substituent may support this explanation. Although the detailed reaction mechanism is still not clear, the dibromooxindole derivatives could be also potentially attractive synthons in indole alkaloid syntheses.
The reaction of 1-(phenylsulfonyl)indole-2-carboxylic acid (10) or 1-(phenylsulfonyl)indole-3-carboxylic acid (11) with 4 equivalents of PhI(OAc)2 in the presence of 4 equivalents of lithium bromide in THF gave 5a in 81-83% yields (Table 2, entries 1, 2), but from 1-methylindole-2-carboxylic acid (12) or 1-methylindole-3-carboxylic acid (13), 8a was isolated in 80% yields (entries 3, 4). Thus, the same results were obtained with the reaction of indole-2,3-dicarboxylic acids (1 and 2) with PhI(OAc)2 and lithium bromide. The reaction of 3a with 2 equivalents of PhI(OAc)2 in the presence of 2 equivalents of lithium bromide gave 5a in 80% yield, while the reaction of 4a with 2 equivalents of PhI(OAc)2 in the presence of 2 equivalents of lithium bromide gave 8a in 90% yield (entries 5, 6).
The attractive results obtained in the hypervalent iodine mediated decarboxylative halogenation of indole-2,3-dicarboxylic acid derivatives prompted us to extent our procedure to the synthesis of polybromoindole alkaloids. When the reaction of 5,6-dibromo-1-(phenylsulfonyl)indole-2,3-dicarboxylic acid (14)21 with 4 equivalents of PhI(OAc)2 in the presence of 4 equivalents of lithium bromide (optimal condition in Table 1) was examined, 1-(phenylsulfonyl)-2,3,5,6-tetrabromoindole (16), the desired product, was obtained in low yield and 3,5,6-tribromo-1-(phenylsulfonyl)indole-2-carboxylic acid (15) was obtained as a major product (Table 3, entry 1). Further optimization of the reaction conditions revealed that the use of 5 equivalents of PhI(OAc)2 in the presence of 5 equivalents of potassium bromide in THF-TFE (trifluoroethanol) afforded 16 in 83% yield (entries 2-7). The conversion of 16 to 2,3,5,6-tetrabromoindole9 was achieved by treatment with tetrabutylammonium fluoride (TBAF) in THF in 92% yield (Scheme 2).
The encouraging results obtained in Table 3 and Scheme 2 prompted us to extend our procedure to several polybromoindole alkaloid syntheses. Dimethyl bromoindole-2,3-dicarboxylates (17 and 18) were prepared according to the reported method.34 The treatment of 17 boron tribromide gave the corresponding dicarboxylic acids (19) in 65% yield (Scheme 3A). The PhI(OAc)2 mediated decarboxylative bromination of 19 afforded tribromo-1-(phenylsulfonyl)indole (20) in 80% yield. 20 could be converted to 2,3,5-tribromoindole8 by treatment with tetrabutylammonium fluoride in 83%. In a similar manner, the synthesis of 2,3,6-tribromoindole8 from 18 was also successfully completed via PhI(OAc)2 mediated decarboxylative bromination (Scheme 3B).
In conclusion, we have demonstrated the decarboxylative halogenation of indolecarboxylic acid derivatives using the Hunsdiecker-type reaction. The exciting result obtained with the reaction of the indole-2,3-dicarboxylic acids (1) with PhI(OAc)2-MX system prompted us to extend our procedure to the synthesis of polybromoindole alkaloids such as 2,3,5,6-tetrabromoindole, 2,3,5-tribromoindole, and 2,3,6-tribromoindole. Further studies along this line are now in progress.
EXPERIMENTAL
All melting points were determined on a Yanagimoto micromelting point apparatus and are uncorrected. 1H NMR spectra were recorded at 300 MHz. Infrared (IR) absorption spectra (cm-1) were recorded using a JASCO FT/IR-7000 spectrophotometer. The high MS were recorded by a JEOL-HX100 spectrometer. Column chromatography was performed on Silica gel 60 N (Kanto Chemical Co., Inc.). Compounds 10-13 were commercially available. Compounds 1,25 2,30 14,21 17,34 and 1834 were prepared by known methods.
Compounds 3a, 4a, 5a, 8a, 16, and 2,3,5,6-Tetrabromoindole have been previously reported by us and the spectral data were in full agreement with those reported.21
Typical Procedure for the decarboxylative halogenation of indole-2,3-dicarboxylic acid with PhI(OAc)2 in the presence of lithium halide: To a mixture of PhI(OAc)2 and lithium halide in THF (10 mL) was added indole-2,3-dicarboxylic acids (1 mmol) at 0 °C and then the reaction mixture was stirred at room temperature. Water was added to the reaction mixture and the mixture was extracted with CH2Cl2. The combined extracts were washed with 2-3% sodium thiosulfate solution, then water, and dried over Na2SO4. The extracts were concentrated under reduced pressure to give a solid, which was purified by column chromatography on silica gel to afford the halogenoindole derivatives.
2,3-Dichloro-1-(phenylsulfonyl)indole (5b). Mp 122 °C; 1H NMR (CDCl3) δ: 7.30-7.63 (6H, m), 7.84-7.92 (2H, m), 8.28 (1H, br d, J = 8.0 Hz, H-7 or H-4); 13C NMR (DMSO-d6) δ: 168.80, 140.58, 131.85, 129.16, 125.13, 124.70, 124.14, 109.08, 26.98; HRMS (EI) m/z: Calcd for C14H9NO2Cl2S: 324.9677. Found: 324.9737; CAS Registry Number [1260070-91-4].
2,3-Diiodo-1-(phenylsulfonyl)indole (5c).29 Mp 165-167 °C (lit.,29 mp 166-167 °C); 1H NMR (CDCl3) δ: 7.25-7.60 (6H, m), 7.90 (2H, br d, J = 8.0 Hz), 8.28 (1H, br d, J = 8.0 Hz, H-7); CAS registry number [80360-26-5].
3,3-Dichloro-1-methyloxindole (8b).32 Mp 144-147 °C (lit.,32 143 °C); IR (Nujol) n: 1740 cm-1; 1H NMR (CDCl3) δ: 3.25 (3H, s, CH3), 6.85 (1H, d, J = 8.0 Hz, H-4 or H-7), 7.17 (1H, t, J = 8.0 Hz, H-5 or H-6), 7.39 (1H, t, J = 8.0, 1.5 Hz, H-6 or H-5), 7.61 (1H, d, J = 8 Hz, H-7 or H-4); 13C NMR (DMSO-d6) δ: 168.80, 140.58, 131.85, 129.16, 125.13, 124.70, 124.14, 109.08, 26.98; CAS registry number [114380-33-5].
2,3-Diiodo-1-methylindole (6c).33 Mp 76-77 °C (lit.,33 76-78 °C); 1H NMR (CDCl3) δ: 3.89 (3H, s, CH3), 7.1-7.42 (2H, m); 13C NMR (DMSO-d6) δ: 138.11, 131.15, 122.71, 120.80, 120.50, 111.06, 99.78, 71.72, 36.09; HRMS (EI) m/z: Calcd for C9H7NI2: 382.8668. Found: 382.8671; CAS registry number [180623-97-6].
Preparation of bromo-1-(phenylsulfonyl)indole-2,3-dicarboxylic acids: To a solution of dimethyl bromo-1-(phenylsulfonyl)indole-2,3-dicarboxylate derivative (1 mmol) in toluene (10 mL) was added 1M boron tribromide in a CH2Cl2 solution (3 mL). The mixture was then stirred at room temperature overnight. Water was added to reaction mixture and the precipitate was collected by filtration and washed with water, then with n-hexane. The bromo-1-(phenylsulfonyl)indole-2,3-dicarboxylic acid was used without further purification.
5-Bromo-1-(phenylsulfonyl)indole-2,3-dicarboxylic acid (19). 1H NMR (CDCl3) δ: 7.45-7.62 (5H, m, arom), 7.86 (1H, s, H-6), 7.87 (1H, d, J = 8.0 Hz, H-4), 8.17 (1H, d, J = 9.2 Hz, H-7).
6-Bromo-1-(phenylsulfonyl)indole-2,3-dicarboxylic acid (21). 1H NMR (CDCl3) δ: 7.30-7.64 (5H, m, arom), 7.86 (1H, s, H-6), 7.91 (2H, d, J = 7.6 Hz, H-4, 5), 8.50 (1H, s, H-7).
Typical Procedure for the decarboxylative bromination of bromoindole-2,3-dicarboxylic acid with PhI(OAc)2 in the presence of potassium bromide: To a mixture of PhI(OAc)2 and potassium bromide in THF (10 mL) was added bromoindole-2,3-dicarboxylic acids (1 mmol) at 0 °C. After 0.5 h of stirring, the solvent was exchanged to TFE (10 mL) and the reaction mixture was stirred for 2.5 h at room temperature. Water was added to the reaction mixture and the mixture was extracted with CH2Cl2. The combined extracts were washed with 2-3% sodium thiosulfate solution, then water, and dried over Na2SO4. The extracts were concentrated under reduced pressure to give a solid, which was purified by column chromatography on silica gel to afford the bromoindole derivatives.
2,3,5-Tribromo-1-(phenylsulfonyl)indole. 1H NMR (CDCl3) δ: 7.45-7.62 (5H, m, arom), 7.86 (1H, s, H-6), 7.87 (1H, d, J = 8.0 Hz, H-4), 8.17 (1H, d, J = 9.2 Hz, H-7).
2,3,6-Tribromo-1-(phenylsulfonyl)indole: 1H NMR (CDCl3) δ: 7.30-7.64 (5H, m, arom), 7.86 (1H, s, H-6), 7.91 (2H, d, J = 7.6 Hz, H-4, 5), 8.50 (1H, s, H-7).
Syntheses of polybromoindoles: A 1M solution of tetrabutylammonium fluoride in THF (0.04 mL) was added to a mixture of polybromo-1-(phenylsulfonyl)indole in THF (1 mL) at -20 °C under argon and the reaction mixture was stirred for 1 h at the same temperature. Hydrochloric acid (2 %) was added to the mixture and then extracted with CHCl3. The extracts were washed with water, dried over Na2SO4, then concentrated under reduced pressure to afford a residue, which was purified by column chromatography (n-hexane : AcOEt = 3:1) to give the polybromoindole.
2,3,5-Tribromoindole.8 Mp 150-151 °C from EtOAc/hexane (lit.,8 mp 150-151 °C); IR(KBr) ν: 2918, 2850, 1635, 1456, 1434 cm-1 ; 1H NMR (CDCl3) δ: 7.13 (1H, d, J = 8.8 Hz, H-7), 7.29 (1H, dd, J = 8.8, 1.8, H-6), 7.61 (1H, d, J = 1.1 Hz, H-4), 8.34 (1H, br s, N-H); CAS Registry Number [918529-97-2].
2,3,6-Tribromoindole.8 Mp 74-75 °C from EtOAc/hexane (lit.,8 mp 74-75 °C); IR(KBr) ν: 2918, 2850, 1635, 1456, 1434 cm-1; 1H NMR (CDCl3) δ: 7.61 (1H, s, H-4 or H-7), 7.76 (1H, s, H-7 or H-4), 8.38 (1H, br s, NH); CAS Registry Number [918530-08-2].
ACKNOWLEDGEMENTS
This work was partially supported by Grant-in-Aid for Scientific Research (C) from the Japan Society for the Promotion of Science (JSPS) (25460027), Kinki University Research Grant Project, the Research Institute of Meijo University, and also in part “High-Tech Research Center Project” for Private Universities and matching fund subsidy.
References
1. B. Borodin, Liebigs Ann., 1861, 119, 121; CrossRef H. Hunsdiecker and C. Hunsdiecker, Chem. Ber., 1942, 75, 291; CrossRef R. G. Johnson and R. K. Ingham, Chem. Rev., 1956, 56, 219; CrossRef C. V. Wilson, Org. React., 1957, 9, 332; R. A. Sheldon and J. K. Kochi, Org. React., 1972, 19, 279.
2. S. J. Cristol and W. C. Firth, Jr., J. Org. Chem., 1961, 26, 280; CrossRef J. K. Kochi, J. Am. Chem. Soc., 1965, 87, 2500; CrossRef D. H. R. Barton, H. P. Faro, E. P. Serebryakov, and N. F. Woolsey, J. Chem. Soc., 1965, 2438; CrossRef A. McKillop, D. Bromley, and E. C. Taylor, J. Org. Chem., 1969, 34, 1172; CrossRef J. Cason and D. M. Walba, J. Org. Chem., 1972, 37, 669; CrossRef R. C. Cambie, R. C. Hayward, J. L. Jurlina, P. S. Rutledge, and P. D. Woodgate, J. Chem. Soc., Perkin Trans. I, 1981, 2608; CrossRef D. H. R. Barton, D. Crich, and W. B. Motherwell, Tetrahedron, 1985, 41, 3901; CrossRef J. Sinha, S. Layek, G. C. Mandal, and M. Bhattacharjee, Chem. Commun., 2001, 19, 1916; CrossRef B.-S. Koo, E.-H. Kim, and K.-J. Lee, Synth. Commun., 2002, 32, 2275; CrossRef J. P. Das, P. Sinha, and S. Roy, Org. Lett., 2002, 4, 3055. CrossRef
3. J. I. Concepcion, C. G. Francisco, R. Freire, R. Hernandez, J. A. Salazar, and E. Suárez, J. Org. Chem., 1986, 51, 402. CrossRef
4. T. Wirth, 'Hypervalent Iodine Chemistry,' Springer Berlin/Heidelberg, 2003 (Top. Curr. Chem. Vol. 224). CrossRef
5. A. Varvoglis, Tetrahedron, 1997, 53, 1179; CrossRef H. Togo and M. Katohgi, Synlett, 2001, 12, 565; V. V. Zhdankin and P. J. Stang, Chem. Rev., 2002, 102, 2523; CrossRef A. Minatti, Synlett, 2003, 14, 140; R. M. Moriarty, J. Org. Chem., 2005, 70, 2893; CrossRef T. Wirth, Angew. Chem. Int. Ed., 2005, 44, 3656; CrossRef V. V. Zhdankin and P. J. Stang, Chem. Rev., 2008, 108, 5299; CrossRef T. Dohi and Y. Kita, Chem. Commun., 2009, 2073; CrossRef M. Uyanik and K. Ishihara, Chem. Commun., 2009, 2086; CrossRef M. Brown, U. Farid, and T. Wirth, Synlett, 2013, 24, 424. CrossRef
6. R. Singh and G. Just, Synth. Commun., 1988, 18, 1327; CrossRef A. Graven, K. A. Joergensen, S. Dahl, and A. Stanczak, J. Org. Chem., 1994, 59, 3543; CrossRef P. Camps, A. E. Lukach, X. Pujol, and S. Vazquez, Tetrahedron, 2000, 56, 2703; CrossRef V. N. Telvekar, N. D. Arote, and O. P. Herlekar, Synlett, 2005, 16, 2495. CrossRef
7. G. W. Gribble, Environ. Sci. Pollut. Res., 2000, 7, 37; CrossRef G. W. Gribble, Chem. Soc. Rev., 1999, 28, 335; CrossRef G. W. Gribble, Acc. Chem. Res., 1998, 31, 141; CrossRef M. Alvarez, M. Salas, and J. A. Joule, Heterocycles, 1991, 32, 1391. CrossRef
8. K. A. Maruya, Chemosphere, 2003, 52, 409; CrossRef O. R. Suarez-Castillo, L. Beiza-Granados, M. Melendez-Rodriguez, A. Alvarez-Hernandez, M. S. Morales-Rios, and P. Joseph-Nathan, J. Nat. Prod., 2006, 69, 1596. CrossRef
9. C. S. Vairappan, T. Kawamoto, H. Miwa, and M. Suzuki, Planta Med., 2004, 70, 1087; CrossRef G. T. Carter, K. L. Rinehart Jr., L. H. Li, S. L. Kuentzel, and J. L. Connor, Tetrahedron Lett., 1978, 19, 4479. CrossRef
10. A. R. Hodder and R. J. Capon, J. Nat. Prod., 1991, 54, 1661; CrossRef R. S. Norton and R. J. Wells, J. Am. Chem. Soc., 1982, 104, 3628. CrossRef
11. M. R. Brennan and K. L. Erickson, Tetrahedron Lett., 1978, 19, 1637. CrossRef
12. Y. Miki, H. Shirokoshi, and K. Matsushita, Tetrahedron Lett., 1999, 40, 4347. CrossRef
13. Y. Miki and H. Hachiken, Synlett, 1993, 4, 333. CrossRef
14. Y. Miki, Y. Tada, N. Yanase, H. Hachiken, and K. Matsushita, Tetrahedron Lett., 1996, 37, 7753. CrossRef
15. Y. Miki, Y. Tada, and K. Matsushita, Heterocycles, 1998, 48, 1593. CrossRef
16. Y. Miki, Y. Aoki, Y. Tsuzaki, M. Umemoto, and H. Hibino, Heterocycles, 2005, 65, 2693. CrossRef
17. Y. Miki, Y. Tsuzaki, H. Hibino, and Y. Aoki, Synlett, 2004, 15, 2206. CrossRef
18. Y. Miki, Y. Aoki, H. Miyatake, T. Minematsu, and H. Hibino, Tetrahedron Lett., 2006, 47, 5215. CrossRef
19. Y. Miki, M. Kuromatsu, H. Miyatake, and H. Hamamoto, Tetrahedron Lett., 2007, 48, 9093. CrossRef
20. A. Putey, F. Popowycz, and B. Joseph, Synlett, 2007, 18, 419.
21. H. Umemoto, M. Umemoto, C. Ohta, M. Dohshita, H. Tanaka, S. Hattori, H. Hamamoto, and Y. Miki, Heterocycles, 2009, 78, 2845. CrossRef
22. H. Hamamoto, H. Umemoto, M. Umemoto, C. Ohta, M. Dohshita, and Y. Miki, Synlett, 2010, 21, 2593.
23. H. Hamamoto, S. Hattori, K. Takemaru, and Y. Miki, Synlett, 2011, 22, 1563.
24. Y. Miki, H. Umemoto, M. Dohshita, and H. Hamamoto, Tetrahedron Lett., 2012, 53, 1924. CrossRef
25. Y. Miki, H. Hachiken, and I. Yoshikawa, Heterocycles, 1997, 45, 1143. CrossRef
26. D. C. Braddock, G. Cansell, and S. A. Hermitage, Synlett, 2004, 15, 461. CrossRef
27. S. C. Conway and G. W. Gribble, Heterocycles, 1992, 34, 2095. CrossRef
28. T. Dohi, A. Maruyama,
M. Yoshimura, K. Morimoto, H. Tohma, and Y. Kita, Angew. Chem. Int. Ed., 2005, 44, 6193. CrossRef
29. M. G. Saulnier and G. W. Gribble, J. Org. Chem., 1982, 47, 757. CrossRef
30. Y. Liu and G. W. Gribble, Tetrahedron Lett. 2001, 42, 2949; CrossRef L. Baiocchi and M. J. Giannangeli, Heterocycl. Chem., 1988, 25, 1905. CrossRef
31. M. Janda, J. Srogl, and P. Holy, Coll. Czech. Commun., 1981, 46, 3278; CrossRef E. J. Moriconi and J. J. Murray, J. Org. Chem., 1964, 29, 3577. CrossRef
32. A. E. Kellie, D. G. O'Sullivan, and P. W. Sadler, J. Chem. Soc., 1956, 3809; CrossRef A. Hantzsch, Ber., 1921, 54, 1221. CrossRef
33. J. Ezquerra, C. Pedregal, C. Lamas, J. Barluenga, M. Pérez, M. A. G.-Martín, and J. M. González, J. Org. Chem., 1996, 61, 5804. CrossRef
34. Y. Miki, M. Umemoto, M. Nakamura, H. Hibino, N. Ohkita, A. Kato, and Y. Aoki, Heterocycles, 2006, 68, 1893 CrossRef