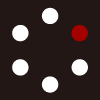
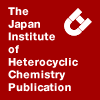
HETEROCYCLES
An International Journal for Reviews and Communications in Heterocyclic ChemistryWeb Edition ISSN: 1881-0942
Published online by The Japan Institute of Heterocyclic Chemistry
e-Journal
Full Text HTML
Received, 14th January, 2015, Accepted, 24th February, 2015, Published online, 6th March, 2015.
DOI: 10.3987/COM-15-13176
■ Environmental Friendly Synthesis of Bis-Perfluoropyridine and Pyrimidine in Water
Reza Ranjbar-Karimi* and Aliyeh Khaje-Khezri
Department of Chemistry, Vali-Asr University, Islamic Republic of Iran, 7713936417, Iran
Abstract
An easy, efficient and simple approach for the synthesis of some perfluoropyridine and pyrimidine derivatives by reaction of pentafluoropyridine and tetrafluoropyrimidine with mono and bidentate N-nucleophiles in the presence of potassium carbonate in water as a green solvent, at room temperature is reported. The structures of some products were unambiguously confirmed by X-ray crystallography.INTRODUCTION
Organic reactions in aqueous media have attracted considerable attention in recent years, since they offer a powerful tool for minimizing waste production and harmful organic solvent dispersal.1 Some particular properties of water make this solvent very attractive (e.g., non-toxicity, non-inflammability, high heat capacity, possibility of controlling pH, isolating insoluble solid products by filtration and recycling inorganic catalysts and itself), allowing organic processes in aqueous medium to be safer, very efficient, and highly selective.2 Because water is natural, abundant, cheap, and readily available, it is thought to be an ideal solvent for both laboratory and industrial chemical processes.3 It is not only abundant, inexpensive, and environmentally benign but it also shows novel reactivity and selectivity for simple synthesis of biologically active compounds in the pharmaceutical or agrochemical industries.4-7 In fact, as clearly stated by Sheldon, it is generally recognized that “the best solvent is no solvent and if a solvent is needed it should preferably be water”.8 Traditionally, organic reactions are carried out in organic solvents (e.g., THF, DMF) and oftentimes at temperatures above ambient,9 although use of (potentially toxic) organic solvents is always the norm, which by definition fails to meet the "12 Principles of Green Chemistry".10
Syntheses of polyfunctional heterocyclic fused ring systems with low molecular weight are important in life science industries.11,12 Pentafluoropyridine has attracted considerable interest due to its synthetic utility. Various multi-functional pyridine derivatives and construction of new heterocyclic and macrocyclic systems could be accessed from simple reaction conditions.13-20 These were including reaction of various bifunctional nucleophiles with pentafluoropyridine. All five fluorine atoms in pentafluoropyridine may be substituted by an appropriate nucleophile due to its highly electron efficient aromatic ring system. The site-reactivity order of pentafluoropyridine is well known.21-24
RESULTS AND DISCUSSION
In our earlier study, we have reported reaction of pentafluoropyridine derivatives with some unequal bidentate nucleophiles in acetonitrile under reflux condition.25 Further, we also successfully utilized ultrasound for the preparation of some pentafluoropyridine derivatives via the reactions of different nucleophiles (mono and bidentate nucleophiles) with pentafluoropyridine in acetonitrile.26 Herein, we wish to report a facile, green and efficient method for preparation of some tetrafluoropyridine and trifluoropyrimidine derivatives via reaction of mono and bidentate N-nucleophiles with pentafluoro- pyridine and tetrafluoropyrimidine respectively in water as a green solvent at room temperature.
The reaction of pentafluoropyridine 1 (X = CF) with 2-aminomethylpyridine in the presence of potassium carbonate in water as a solvent gave 2,3,5,6-tetrafluoro-N-(pyridin-2-ylmethyl)pyridin-4-amine 3a in 80% yields (Table 1, entry 1).
The 19F NMR spectrum of 3a had a chemical shift at -97.1 ppm, in which the resonance attributed to fluorine located ortho to ring nitrogen, similar to the shift observed for the analogous system. The corresponding resonance for fluorine located meta to ring nitrogen occurred at –164.0 ppm, similar to the shifts observed for the analogous systems.15,16
The present methodology has been found successful in the nucleophilic substitution of aliphatic diamine with pentafluoropyridine to achieve bis-tetrafluoropyridine derivatives (Table 1, entries 2-4, compound 3b-d). These reactions were also carried out in the presence of potassium carbonate in water solvent and identification of product was done by 1H, 13C, 19F NMR analyses, mass spectra and elemental analyses.
The 19F NMR spectra of 3b, 3c and 3d had a chemical shift at -95.42, -94.22 and -94.58 ppm, in which the resonance attributed to fluorine located ortho to ring nitrogen, similar to the shift observed for the analogous system. The corresponding resonance for fluorine which was located meta to ring nitrogen occurred at -154.23, -164.71 and -164.85 ppm, similar to the shifts observed for the analogous systems.
Similarly, the reaction of aliphatic diamine with tetrafluoropyrimidine (Table 1, X = N) in the presence of potassium carbonate in water solvent gave bis-trifluoropyrimidine derivatives from regiospecific substitution of fluorine attached to the 4-position of tetrafluoropyrimidine similar to reported earlier work27 (Table 1, entries 5-9, compound 3e-i). The structures of 3e-i could be determined by 19F NMR spectroscopy which showed three resonances in the range -47.66 - -48.75, -80.66 - -89.74 and -180.12 - -181.16 ppm for F-5, F-2 and F-6 respectively in a 1:1:1 ratio. A consideration of the 19F NMR substituent chemical shifts from literature data27 and this work, confirmed the structure of 3e-i.
The structures of compounds 3a and 3b were also confirmed by X-ray crystallography. Molecular structures and ORTEP plots of compounds 3a and 3b are shown in Figures 1 and 2, respectively.
The asymmetric unit of the compound 3b comprised half of the molecule in which the center of symmetry was located in the middle of pipyrazine ring. In the crystal packing of 3b, molecules were connected together by the intermolecular C–H…F and C–F…π interactions, forming a3-D network. The interesting feature of the crystal structure of 3b is the weak intermolecular F(2)…F(4) [2.858(2) A° ] interaction which is shorter than the sum of the van der Waals radius [2.94 A°] of F atoms.28 The asymmetric unit of the compound 3a comprised a molecule of compound 3a. In the crystal packing of 3a, molecules were connected together by the intermolecular C–H…F interaction into a 1-D chain along the b-axis of the unit cell (Table 2). The crystal packing also showed intermolecular π…π interaction with centroid to centroid distance of 3.7008(16) Ǻ [Cg(1)…Cg(2)i = 3.7008(16) Ǻ; Cg1 = N1/C7–C11; Cg2 = N3/C1–C5 (i) 3/2- x,-1/2+ y,3/2- z].29
In conclusion, as has been shown in this paper, water can be used as a green solvent for preparation of some perfluoropyridine and pyrimidine derivatives in high yield at room temperature.
EXPERIMENTAL
Melting points were determined in open capillary tubes by an Electrothermal IA 9000 melting point apparatus. FT-IR spectra of all the final products were recorded on a Bruker instrument by using the KBr self-supported pellet technique. The 13C NMR, 1H NMR spectra were recorded on a Bruker Avance-300 75, and 125 MHz for 13C NMR, 300, 400, and 500 MHz for 1H NMR. NMR spectra were obtained in solution of DMSO-d6 and CDCl3 using tetramethylsilane (TMS) as internal standard. The 19F NMR spectra were recorded at 470 MHz. In the 19F NMR spectra, upfield shifts were quoted as negative and referenced to CFCl3. Mass spectra were taken by a Micromass Platform II: EI mode (70 eV). Elemental analyses were obtained on an Exeter Analytical CE-440 elemental analyser. Medium pressure (‘flash’) column chromatography was performed using silica (Merck #60). Silica plates (Merck) were used for TLC analysis.
Starting Materials
All starting materials were commercially available.
General procedure for preparation of compound 3a-i
Potassium carbonate (0.2 mmol) was added to the mixture of nucleophile (0.1 mmol) in water (3 mL). Then pentafluoropyridine or tetrafluoropyrimidine (0.1 mmol for 3a and 0.2 mmol for other compounds) was added and the resulting solution was stirred at room temperature for a few hours. After completion of the reaction as indicated by TLC, CHCl3 (20 mL) and brine (20 mL) were added then the organic layer collected. The CHCl3 was evaporated and column chromatography on silica gel using EtOAc-hexane (1:5) as eluent (for compound 3f and 3h) or recrystallization from EtOAc (for all compounds except 3f and 3h) gave the product.
2,3,5,6-Tetrafluoro-N-(pyridin-2-ylmethyl)pyridin-4-amine 3a
(0.06 g, 80%) as a white solid; mp 112-115 oC; Anal. Calcd for C11H7F4N3: C, 51.4; H, 2.7; N, 16.4%. Found: C, 51.3; H, 2.5; N, 16.3; δF (470 MHz, DMSO-d6); -97.0 (2F, m, F-2), -164.0 (2F, m, F-3); δH (500 MHz, DMSO-d6); 4.71 (d, 2H, 3JHH = 6.2 Hz, CH2), 7.30 (1H, m, Ar-H), 7.32 (1H, d, 3JHH = 7.9 Hz, Ar-H), 7.83-8.20 (1H, m, Ar-H), 7.91 (s, NH), 8.53 (1H, d, 3JHH = 4.4 Hz, Ar-H); δC (125 MHz, DMSO-d6); 48.3 (t, 4JCF = 3.6 Hz, CH2), 120.6 (s, Ar-C), 122.3 (s, Ar-C), 130.8 (ddd, 1JCF = 242.3, 2JCF = 35.7, 3JCF = 18.6 Hz, C-3), 136.9 (s, Ar-C), 138.5 (m, C-4), 143.6 (ddd, 1JCF = 233.8, 2JCF = 18.6, 3JCF = 9.5 Hz, C-2); 149.0 (s, Ar-C), 158.1 (s, Ar-C); MS (EI+), m/z (%)= 237.06 (M-20, 2), 191 (65), 71 (43), 55 (100).
1,4-Bis(perfluoropyridin-4-yl)piperazine 3b
(80%), white solid, mp 198-200 oC; Anal. Calcd for C14H8F8N4: C, 43.76; H, 2.10; N, 14.58% Found: C, 43.63; H, 1.95; N, 14.36%; δF (470 MHz, DMSO-d6); -95.42 (4F, m, F-2), -154.23 (4F, m, F-3); δH (500 MHz, DMSO-d6); 3.59 (8H, s, CH2); δC (125 MHz, CDCl3); 46.24 (s, CH2), 137.61 (dd, 1JCF = 245.0, 2JCF = 32.3 Hz, C-3), 138.36 (m, C-4), 145.00 (ddd, 1JCF = 230.0, 2JCF = 29.1, 3JCF =7.8 Hz, C-2).
N,N'-Bis-(2,3,5,6-tetrafluoro-pyridin-4-yl)-butane-1,4-diamine 3c
(0.04 g, 90%), white solid, mp 67-68 oC; Anal. Calcd for C14H10F8N4: C, 43.53; H, 2.61; N, 14.51% Found: C, 43.45; H, 2.55; N, 14.46%; δF (475 MHz, CDCl3); -94.22 (4F, m, F-2), -164.71(4F, m, F-3); δH (500 MHz, CDCl3); 1.73 (4H, m, CH2), 3.57 (4H, t, 3JHH = 5.2 Hz, CH2), 4.62 (2H, bs, NH); δC (125 MHz, CDCl3); 22.79 (s, CH2), 39.15 (t, 4JCF = 4.1 Hz, CH2), 125.97 (ddd, 1JCF = 246.2, 2JCF = 34.5, 3JCF = 2.8 Hz, C-3), 133.31 (m, C-4), 139.43 (ddd, 1JCF = 225.5, 2JCF = 19.4, 3JCF = 15.2 Hz, C-2); MS (EI+), m/z (%) = 386 (M+, 10), 191 (30), 178 (100), 131 (24), 81 (14).
2,3,4,5,6-Pentafluoro-N-(2-methyl-5-(perfluorophenylamino)pentyl)benzenamine 3d
(0.053 g, 89%), white solid, mp 59-61 oC; Anal. Calcd for C16H14F8N4: C, 46.38, H, 3.41, N, 13.52% Found; C, 46.43; H, 3.25; N, 13.39%; δF (375 MHz, CDCl3); -94.58 (m, 4F, F-2), -164.85 (m, 4F, F-3); δH (400 MHz, CDCl3); 1.06 (3H, d, 3JHH = 6.8 Hz, CH3), 1.28 (2H, m, CH2), 1.51 (2H, m, CH2), 1.76 (1H, m, CH) 3.32 (2H, m, CH2), 3.52 (2H, m, CH2), 4.6 (2H, bs, NH). δC (100 MHz, CDCl3); 17.41 (s, CH3), 25.83, 28.46, 31.15 (s, CH2), 34.48 (s, CH), 45.15 (t, 4JCF = 4.2 Hz, NCH2), 50.88 (t, 4JCF = 9.8 Hz, NCH2), 131.27 (dd, 1JCF = 242.3, 2JCF = 34.0, C-3), 137.81 (m, C-4), 144.47 (dm, 1JCF = 248.2 Hz, C-2); MS (EI+), m/z (%) = 414 (M+, 50), 395 (100), 324 (70), 305 (26), 276 (23).
N-(2-(2,5,6-Trifluoropyrimidin-4-ylamino)ethyl)-2,5,6-trifluoropyrimidin-4-amine 3e
(0.029 g, 92%), white solid, mp 188-190 oC; Anal. Calcd for C10H6F6N6: C, 37.05; H, 1.87; N, 25.92% Found: C, 37.01; H, 1.81; N, 25.82%; δF (188 MHz, CDCl3); -48.75 (2F, d, 3JFF = 25.5 Hz, F-5), -89.74 (2F, d, 4JFF = 16.3 Hz, F-2), -180.12 (2F, dd, 3JFF = 25.5, 4JFF = 16.3 Hz, F-6); δH (400 MHz, CDCl3); 3.56 (4H, s, CH2), 7.99 (2H, bs, NH); δC (100 MHz, CDCl3); 60.29 (s, CH2), 128.16 (ddd, 1JCF = 253.8, 2JCF = 23.6, 3JCF = 9.1 Hz, C-5), 155.01 (ddd, 1JCF = 214.1, 2JCF = 21.8, 3JCF = 4.0 Hz, C-6), 155.32 (ddd, 1JCF = 230.2, 2JCF = 20.5, 3JCF = 8.8 Hz, C-2), 156.97(m, C-4); MS (EI+), m/z (%) = 324 (M+, 2), 175 (42), 163 (37), 161 (100), 114 (15).
N-(3-(2,5,6-Trifluoropyrimidin-4-ylamino)propyl)-2,5,6-trifluoropyrimidin-4-amine 3f
(0.031 g, 85%), Oily; Anal. Calcd for C11H8F6N6: C, 39.06; H, 2.38; N, 24.85% Found: C, 38.92; H, 2.16; N, 24.71%; δF (376 MHz, CDCl3); -47.73 (2F, d, 3JFF = 25.5 Hz, F-5), -87.16 (2F, d, 4JFF = 16.7 Hz, F-2), -180.31 (2F, dd, 3JFF = 25.5, 4JFF = 16.7 Hz, F-6); δH (400 MHz, CDCl3); 1.92 (2H, pent, 3JHH = 6.5 Hz, CH2), 3.61 (4H, t, 3JHH = 6.5 Hz, CH2), 6.40 (2H, bs, NH); δC (100 MHz, CDCl3); 29.45, 38.17 (s, CH2), 128.08 (ddd, 1JCF = 250.6, 2JCF = 23.0, 3JCF = 9.2 Hz, C-5), 153.37 (ddd, 1JCF = 294.8, 2JCF = 19.8, 3JCF = 12.7 Hz, C-6), 155.19 (ddd, 1JCF = 218.7 , 2JCF = 21.8, 3JCF = 4.0 Hz, C-2), 157.30 (m, C-4); MS (EI+), m/z (%) = 338 (M+, 2), 175 (40), 161 (100), 114 (36).
N-(3-(2,5,6-Trifluoropyrimidin-4-ylamino)propyl)-2,5,6-trifluoropyrimidin-4-amine 3g
(0.032 g, 90%), white solid, mp 118-121 oC; Anal. Calcd for C12H10F6N6: C, 40.92; H, 2.86; N, 23.86% Found: C, 40.80; H, 2.72; N, 23.80%; δF (188 MHz, CDCl3); -48.10 (2F, d, 3JFF = 25.1 Hz, F-5), -80.66 (2F, d, 4JFF = 16.3 Hz, F-2), -180.45 (2F, dd, 3JFF = 25.1, 4JFF = 16.3 Hz, F-6); δH (400 MHz, CDCl3); 1.56 (4H, m, CH2), 3.46 (4H, t, 3JHH = 7.2 Hz, CH2), 8.37 (2H, bs, NH); δC (100 MHz, CDCl3); 26.28, 31.21 (s, CH2), 127.95 (ddd, 1JCF = 250.4, 2JCF = 22.6, 3JCF = 7.5 Hz, C-5), 155.10 (ddd, 1JCF = 214.7, 2JCF = 23.3, 3JCF = 3.3 Hz, C-6), 155.80 (ddd, 1JCF = 236.7, 2JCF = 20.6, 3JCF = 10.9 Hz, C-2), 156.9 (m, C-4); MS (EI+), m/z (%) = 352 (M+, 2), 161 (100).
N-(3-(2,5,6-Trifluoropyrimidin-4-ylamino)propyl)-2,5,6-trifluoropyrimidin-4-amine 3h
(0.034 g, 90%), Oily; Anal. Calcd for C15H16F6N6 requires C, 45.69; H, 4.09; N, 21.31% Found: C, 45.64; H, 4.02; N, 21.30%; δF (376 MHz, CDCl3); -47.66 (2F, d, 3JFF = 25.1 Hz, F-5), -87.87 (2F, d, 4JFF = 17.1 Hz, F-2), -181.05 (2F, dd, 3JFF = 25.1, 4JFF = 17.1 Hz, F-6); δH (400 MHz, CDCl3); 1.36 (6H, m, CH2), 1.62 (4H, m, CH2), 3.50 (4H, t, 3JHH = 7.2 Hz, CH2), 5.63 (2H, bs, NH); δC (100 MHz, CDCl3); 26.74, 28.97, 29.34, 29.41, 41.72 (s, CH2), 128.01 (ddd, 1JCF = 248.4, 2JCF = 22.9, 3JCF = 8.8 Hz, C-5), 155.26 (ddd, 1JCF = 215.6 , 2JCF = 21.3, 3JCF = 3.4 Hz, C-6), 156.10 (ddd, 1JCF = 251.8, 2JCF = 10.4, 3JCF = 10.7 Hz, C-2), 156.87 (m, C-4); MS (EI+), m/z (%) = 352 (M+, 2), 161 (100).
N-(3-(2,5,6-Trifluoropyrimidin-4-ylamino)propyl)-2,5,6-trifluoropyrimidin-4-amine 3i
(0.036 g, 88%), white solid; mp 79-83 oC; Anal. Calcd for C18H22F6N6 requires C, 51.09; H, 4.71; N, 11.72% Found: C, 50.97; H, 4.96; N, 11.64%; δF (188 MHz, CDCl3); -47.57 (2F, d, 3JFF = 25.5 Hz, F-5), -87.67 (2F, d, 4JFF = 16.6 Hz, F-2), -181.16 (2F, dd, 3JFF = 25.5, 4JFF = 16.6 Hz, F-6); δH (500 MHz, CDCl3); 1.33 (12H, m, CH2), 1.64 (4H, pent, 3JHH = 7.3 Hz, CH2), 3.51 (4H, t, 3JHH = 7.2 Hz, CH2), 5.53 (2H, bs, NH); δC (125 MHz, CDCl3); 26.87, 29.35, 29.50, 29.57, 29.58, 41.83 (s, CH2), 128.02 (ddd, 1JCF = 250.6, 2JCF = 23.1, 3JCF = 9.4 Hz, C-5), 155.32 (ddd, 1JCF = 216.67, 2JCF = 21.0, 3JCF = 3.9 Hz, C-6), 156.50 (ddd, 1JCF = 238.0, 2JCF = 21.3, 3JCF = 8.8 Hz, C-2), 157.31 (m, C-4); MS (EI+), m/z (%) = 436 (M+, 2), 175 (55), 161 (100), 148 (34).
ACKNOWLEDGEMENTS
The author wish to thank Vali-e-Asr University of Rafsanjan for partially funding this work.
References
1. For reviews see: (a) P. Tundo and P. T. Anastas, Green Chemistry: Challenging Perspectives, Oxford University Press, Oxford, 1999; (b) J. M. DeSimone, Science, 2002, 297, 799. CrossRef
3. For reviews see: (a) B. L. Mojet, S. D. Ebbesen, and L. Lefferts, Chem. Soc. Rev., 2010, 39, 4643; (b) C.-J. Li, Acc. J. Chem. Res., 2010, 43, 581; CrossRef (c) M. Lamblin, L. Nassar-Hardy, J. C. Hierso, E. Fouquet, and F. X. Felpin, Adv. Synth. Catal., 2010, 352, 33; CrossRef (d) A. Chanda and V. V. Fokin, Chem. Rev., 2009, 109, 725; CrossRef (e) D. Burtscher and K. Grela, Angew. Chem. Int. Ed., 2009, 48, 442. CrossRef
4. C.-J. Li, Chem. Rev., 2005, 105, 3095. CrossRef
5. K. Manabe, S. Limura, X. M. Sun, and S. Kobayashi, J. Am. Chem. Soc., 2002, 124, 11971. CrossRef
6. S. V. Chankeshwara and A. K. Chakraborti, Org. Lett., 2006, 8, 3259. CrossRef
7. G. L. Khatik, R. Kumar, and A. K. Chakraborti, Org. Lett., 2006, 8, 2433. CrossRef
8. R. A. Sheldon, J. Mol. Catal. A, 1996, 107, 75. CrossRef
9. For reviews see: (a) A. F. Littke and G. C. Fu, Angew. Chem. Int. Ed., 1999, 38, 2411; (b) S. P. H. Mee, V. Lee, and J. E. Baldwin, Chem. Eur. J., 2005, 11, 3294; CrossRef (c) J.-H. Li, Y. Liang, D.-P. Wang, W.-J. Liu, Y.-X. Xie, and D.-L. Yin, J. Org. Chem., 2005, 70, 2832. CrossRef
10. For reviews see: (a) P. T. Anastas and J. C. Warner, Green Chemistry: Theory and Practice, Oxford University Press, Oxford, 1998; (b) P. T. Anastas and T. Williamson, Green Chemistry, Frontiers in Benign Chemical Synthesis and Process, Oxford University of Press, Oxford, 1998; (c) C. Capello, U. Fischer, and K. Hungerbuhler, Green Chem., 2007, 9, 927. CrossRef
11. J. A. Joule and K. Mills, Heterocyclic Chemistry, Blackwell, Oxford, 2000.
12. I. Collins, J. Chem. Soc., Perkin Trans. 1, 2002, 1921.
13. H. Benmansour, R. D. Chambers, P. R. Hoskin, and G. Sandford, J. Fluorine Chem., 2001, 112, 133. CrossRef
14. A. Barone, G. Sandford, R. Slater, D. S. Yufit, J. A. K. Howard, and A. Vong, J. Org. Chem., 2005, 70, 9377. CrossRef
15. G. Sandford, R. Slater, D. S. Yufit, J. A. K. Howard, and A. Vong, J. Org. Chem., 2005, 70, 7208. CrossRef
16. C. A. Hargreaves, G. SandfordR. Slater, D. S. Yufit, J. A. K. Howard, and A. Vong, Tetrahedron, 2007, 63, 5204. CrossRef
17. R. D. Chambers, P. R. Hoskin, G. Sandford, D. S. Yufit, and J. A. K. Howard, J. Chem. Soc., Perkin Trans.1, 2001, 2788. CrossRef
18. R. D. Chambers, P. R. Hoskin, A. R. Kenwright, A. Khalil, P. Richmond, G. Sandford, R. Slater, D. S. Yufit, and J. A. K. Howard, Org. Biomol. Chem., 2003, 1, 2137. CrossRef
19. R. D. Chamber, A. Khalil, C. B. Murray, G. Sandford, A. S. Batsanov, and J. A. K. Howard, J. Fluorine Chem., 2005, 126, 1002. CrossRef
20. R. D. Chambers, P. R. Hoskin, A. Khalil, P. Richmond, G. Sandford, D. S. Yufit, and J. A. K. Howard, J. Fluorine Chem., 2002, 116, 19. CrossRef
21. R. D. Chambers and C. R. Sargent, Adv. Heterocycl. Chem., 1981, 28, 1. CrossRef
22. G. M. Brooke, J. Fluorine Chem., 1997, 86, 1. CrossRef
23. W. J. Guilford, J. Dallas, and D. Arnaiz, J. Labelled Compd. Radiopharm., 2001, 44, 247. CrossRef
24. H. Amii and K. Uneyama, Chem. Rev., 2009, 109, 2119. CrossRef
25. R. Ranjbar-Karimi and M. Mousavi, J. Fluorine Chem., 2010, 131, 587. CrossRef
26. R. Ranjbar-karimi, M. Mashak-Shoshtari, and A. Darehkordi, Ultrason. Sonochem., 2011, 18, 258. CrossRef
27. E. L. Parks, G. Sandford, D. S. Yufit, J. A. K. Howard, J. A. Christopher, and D. D. Miller, Tetrahedron, 2010, 66, 6195. CrossRef
28. A. Bondi, J. Phys. Chem., 1964, 68, 441. CrossRef
29. The X-ray single crystal data for 3a and 3b were collected at 296 (1) K on STOE IPDS II diffractometers (Mo Ka = 0.71073 Ǻ). Cell parameters were retrieved using X-AREA software and refined using X-AREA on all observed reflections. Data reduction and correction for Lp (Lorentz-polarization) and decay were performed using X-AREA software. Absorption corrections were applied using MULABS in PLATON. All structures were solved by direct methods and refined by full-matrix least squares on F2 for all data using SHELXTL software. All calculations were performed by PLATON. All non-hydrogen atoms were refined anisotropically. Hydrogen atoms were positioned geometrically and refined with ariding model approximation with their parameters constrainedto the parent atom with Uiso (H) = 1.2 Ueq (C). N-bound hydrogen atoms were located from the difference Fourier map and refined to their parent atoms.