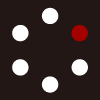
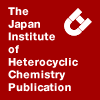
HETEROCYCLES
An International Journal for Reviews and Communications in Heterocyclic ChemistryWeb Edition ISSN: 1881-0942
Published online by The Japan Institute of Heterocyclic Chemistry
e-Journal
Full Text HTML
Received, 17th January, 2015, Accepted, 15th April, 2015, Published online, 11th May, 2015.
DOI: 10.3987/COM-15-13175
■ Triterpene Saponins from the Aerial Parts of Ilex cornuta and Their Cytotoxic Activity
Seung Young Lee, Won Se Suh, Ho Kyung Kim, Il Kyun Lee, Sang Un Choi, Ki Hyun Kim, and Kang Ro Lee*
Natural Products Laboratory, School of Pharmacy, Sungkyunkwan University, 300 Chunchun-dong, Jangan-ku, Suwon 440-746, Korea
Abstract
Four new triterpene saponins (1-4), together with 13 known triterpenoids (5-17), were isolated from the MeOH extract of Ilex cornuta Lindley (Aquifoliaceae). Their structures were elucidated on the basis of chemical and spectroscopic methods. The isolated compounds were tested for their cytotoxicities against four human tumor cell lines (A549, SK-OV-3, SK-MEL-2, and HCT15) in vitro using the sulforhodamine B (SRB) assay.Ilex cornuta Lindley (the Chinese holly or horned holly) belongs to the family Aquifoliaceae, and this herb is native to eastern China and Korea.1 This species have been used in traditional Chinese medicine for the treatment of intertrigo, headache, dizziness, and hypertension, and for purifying the blood.2 The water extract from its leaves has been used as contraceptive and anti-bacterial agents.3 Previous phytochemical studies on this plant reported the isolation of various triterpene saponins as main constituents such as ilexside, zigu-glucoside, cornutaside, and cornutaoside.3-7 Some of these isolates have been reported to exhibit increase in coronary blood flow and anti-hematoblastic coagulation activity.5
We have recently reported the isolation of triterpene derivatives from the MeOH extract of I. cornuta.8 In continuing research on this source, four new triterpene saponins (1-4), together with 13 known triterpenoids (5-17) were isolated and identified (Figure. 1). The structures were determined using spectroscopic methods including 1D and 2D NMR (1H, 13C NMR, COSY, HMQC, HMBC, and ROESY) and HR-MS data as well as chemical methods. Herein, we report the isolation, structural elucidation of the new compounds, and cytotoxic effects of these isolated compounds (1-17).
Compound 1, a colorless gum, has the molecular formula C35H56O9 as determined by the positive ion HRFAB-MS m/z 643.3825 [M + Na]+ (calcd. for C35H56NaO9 643.3822). The 13C NMR and DEPT spectrum (Table 1) exhibited 35 carbon signals composed of one carboxylic carbon at δC 180.8, six methyl carbons at δC 29.9, 24.5, 17.4, 16.2, 16.1, and 13.7, two olefinic carbons at δC 139.6, and 127.3, one oxygenated methine carbons at δC 82.1, one oxygenated methylene carbon at δC 64.5, four methine carbons at δC 48.0, 47.7, 47.5, and 42.2, nine methylene carbons at δC 38.9, 33.3, 32.5, 29.3, 27.1, 26.2, 25.0, 24.1 and 18.4, six quaternary carbons at δC 73.2, 47.9, 43.6, 42.2 40.3 and 37.0, and five remaining signals at δC 106.7, 74.6, 73.5, 69.7, and 67.0 assignable to arabinose moiety. The 1H NMR spectrum of 1 (Table 2) displayed the signals of an olefinic proton at δH 5.57 (1H, br t, H-12), one oxygenated methine proton at δH 4.26 (1H, m, H-3), a couple of oxymethylene protons at δH 4.28 and 3.70 (each 1H, d, J = 11.2 Hz, H-23), one methine proton at δH 3.29 (1H, s, H-18), five tertiary methyl protons at δH 1.70 (3H, s, H-27), 1.44 (3H, s, H-29), 1.11 (3H, s, H-26), 0.99 (3H, s, H-25) and 0.94 (3H, s, H-24), one secondary methyl proton at δH 1.14 (3H, d, J = 6.0 Hz, H-30), and one sugar anomeric protons at δH 4.99 (1H, d, J = 7.0 Hz, H-1′). The NMR spectral data were very similar to those of mateside (10),9 except for stereochemistry of methyl group at C-30.10 The β-orientation of the methyl group at C-30 was determined from correlations of the methyl group CH3-30 (H 1.14)/CH3-29 (H 1.44) and H-18 (H 3.29) in the ROESY spectrum (Figure. 2). And, upfield shift of C-18 (C 47.5 in 1; C 54.7 in 10) and C-22 (C 32.5 in 1; C 38.6 in 10) can be explained by γ-effect for the 30β-methyl group.11,12 The above data suggested that the absolute configuration of C-20 was to be 20S.11,12 Acid hydrolysis of compound 1 with HCl gave aglycone, 20(S)-rotundic acid, and L-arabinose, which were identified by GC analysis with authentic samples. Therefore, compound 1 was identified as 3β,19α,23-trihydroxy-20(S)-urs-12-en-28-oic acid 3β-O-α-L-arabinopyranoside.
Compound 2 was obtained as a colorless gum. Its molecular formula C43H68O14 was inferred from the positive ion HRESI-MS m/z 831.4500 [M + Na]+ (calcd. for C43H68NaO14 831.4507). The NMR spectra of 2 were very similar to those of brevicuspisaponin 3 (13).13 The major differences were the presence of additional acetyl group signals (H 2.05, C 170.2 and 21.3).5 The position of acetyl group was confirmed by HMBC experiment, which showed correlations between the H-2' (H 5.88) of α-arabinose and a carbonyl carbon (C 170.2).5 The stereochemistry of 2 was established based on the ROESY correlation between CH3-30/CH3-29 and H-18, suggested that the methyl group at C-30 was of β-orientation (Figure 2). This demonstrated the axial position of methyl group at C-30, indicating the 20S-configuration.11,12 Acid hydrolysis of 2 with 1N HCl gave two sugars, D-glucose and L-arabinose, which were identified by GC analysis and TLC comparison with authentic samples.7,14 Thus, the structure of 2 was established as 3-O-α-L-2'-acetoxyl-arabinopyranosyl-3β,19α-dihydroxy-20(S)-urs-12-en-28-oic acid28-O-β-D-glucopy-ranoside.
Compound 3 was obtained as a colorless gum whose molecular formula was determined to be C35H56O9 from the [M + Na]+ peak at m/z 643.3820 (calcd. for C35H56NaO9 643.3822) in the HRESI-MS. The 1H NMR spectrum of 3 displayed signals for seven methyl groups at H 0.83 (3H, s, H-25), 0.94 (3H, s, H-24), 1.02 (3H, d, J = 7.0 Hz, H-30), 1.25 (3H, s, H-27), 1.29 (3H, s, H-23), 1.30 (3H, s, H-26), and 1.34 (3H, d, J = 7.0 Hz, H-29), one olefinic proton at H 6.16 (1H, br s, H-18), and four oxygenated methine protons at H 3.38 (1H, dd, J = 11.5, 4.5 Hz, H-3), 4.02 (1H, m, H-19), 4.63 (1H, m, H-12), and 5.08 (1H, m, H-21) (Table 2). The 13C NMR spectrum of 3 exhibited 30 carbon signals, including seven methyl carbons at δ 10.6, 17.0, 17.2, 19.3, 21.9, 22.6, and 28.6, two olefinic carbons at δ 120.4 and 150.9, four oxygenated methines at δc 68.6, 69.6, 78.1, and 88.8, one carbonyl carbon at δc 179.7, three methines δc 56.4, 50.2, and 46.0, together with one sugar signals at δc 107.9, 73.3, 75.0, 69.9, and 67.2 (Table 1). These data implied that the aglycone part of 3 could be a 3,18,19,21-terthydroxy-18,19-seco-ursan derivative.7 Additionally, The 1H–1H COSY correlations of H-21 to H-22, H-20, and H-20 to H-30, H-19 and the HMBC cross-peaks of H-18 with C-17, C-22 and H-22 with C-28, and H-21 with C-22, C-20 provided the information of connection from γ-lactone ring moiety through the spiro carbon at C-17. Consequently, the structure of E ring was elucidated as spiro-γ-lactone at C-17 by the signals of C 179.7 (C-28), 78.1 (C-21), 46.1 (C-17), 43.7 (C-22) in the 13C NMR spectrum.
The sugar unit was identified as L-arabinopyranose by the signals of H 4.72 (d, J = 7.0 Hz), 4.45 (m, H-2'), 4.33 (m, H-4'), 4.32 (m, Hb-5'), 4.18 (m, H-3'), and 3.83 (m, Ha-5') in the 1H NMR spectrum and C 107.9, 75.0, 73.3, 69.9, and 67.2 in the 13C NMR spectrum.15,16 The coupling constant (J = 7.0 Hz) of the anomeric proton signal at H 4.72 indicated to be α-form.15,16 The sugar position was determined by HMBC experiment, in which the long-range correlation was observed between the H-1' (δ 4.72) of L-arabinopyranose and the C-3 (δ 88.8) of aglycone (Figure. 2). The 1H and 13C NMR, HMQC, 1H-1H COSY, HMBC and ROESY spectra of 3 were almost same with those of dunnianaolactone B17, which was isolated from Ilex dunniana, except the signals correlated to a sugar unit, arabinose. The relative configurations of 3 were identified by analysis of ROESY data and comparison of the previous reported data, indicating that the H-3, H-5, H-9, H-12, Me-23, and Me-27 were α-orientation and Me-24, Me-25, and Me-26 were β-orientation. (Figure. 2). The ROESY cross-peaks of H-22α (δ 2.28) /H-21 (δ 5.08), and H-19 (δ 4.02) /H-21 (δ 5.08) showed the same orientation for H19, H-21, and H-22α. The sugar, α-L-arabinopyranose was confirmed by GC analysis and optical rotation after acid hydrolysis.14,18 Thus, the structure of 3 was established as (3β,12β)-3-O-α-L-arabinopyranosyl-12,19-dihydroxy-18,19- secours-13(18)-ene-28,21-lactone.
Compound 4 was obtained as a colorless gum, and its molecular formula C41H66O15 was inferred from the positive ion HRFAB-MS m/z 803.4190 [M+ Na]+ (calcd. for C41H64NaO14 803.4194). The 1H and 13C NMR spectra of 4 were almost same with those of compound 3, except for the presence of a ketone group at C-19. The position of ketone group was determined at C-19 on the basis of the HMBC correlations between a H-30 (δ 1.02) of methyl group to C-19 (δ 209.0). The 1H–1H COSY correlations of H-21 to H-22, H-20, and H-20 to H-30 and the HMBC cross-peaks of H-18 with C-17, C-22 and H-22 with C-28, and H-21 with C-22, C-20 provided the information of connection from γ-lactone ring through the spiro carbon at C-17. Consequently, the structure of E ring was elucidated as spiro-γ-lactone at C-17 by the signals of C 178.7 (C-28), 77.3 (C-21), 45.3 (C-17), 43.3 (C-22) in the 13C NMR spectrum. Also, the analysis of 1H and 13C NMR spectra of 4 revealed the presence of arabinose and glucose units (Table 1 and 2). The configurations of the sugars were determined through the coupling constants of the anomeric protons to be α-arabinopyranose (δH 4.99, d, J = 5.5 Hz), and β-glucopyranose (δH 5.19, d, J = 7.5 Hz). The positions of two sugars were confirmed by the HMBC correlations between H-1' (δ 4.99) of arabinopyranose to C-3 (δ 88.7) of aglycone, and between H-1'' (δ 5.19) of glucopyranose to C-2' (δ 81.0) of arabinopyranose. The relative configurations of 4 were identified by the ROESY data and a comparison with previously reported data, indicating that the H-3, H-5, H-9, H-12, Me-23 and Me-27 were α-oriented and Me-24, Me-25, and Me-26 were β-oriented (Figure. 2). The ROESY cross-peaks of H-22α (δ 2.24) /H-21 (δ 4.82) showed the same orientation for H-21, and H-22α. The sugar, α-L-arabinose and β-D-glucose in 4 were confirmed by GC analysis and optical rotation after acid hydrolysis.14,18 Therefore, the structure of 4 was determined as (3β,12β)-3-[β-D-glucopyranosyl- (1→2)-α-L-arabinopyranosyl]-12-hydroxy-19-oxo-18,19-secours-13(18)-en-28,21-lactone.
The known compounds were identified as cornutaoside A (5),7 ilexasprellanoside C (6),19 ilexoside G (7),16 zigu-glucoside I (8),5 ilexside II (9),4 mateside (10),9 ilexoside XXVII (11),20 ilexoside XXX (12),21 brevicuspisaponin 3 (13),13 bifinoside A (14),22 ursolic acid (15),23 oleanolic acid (16),23 and 3β-Hydroxy-24-nor-urs-4(23),12-dien-28-oic acid (17)6 by comparison their NMR data with those reported in the literature values.
The cytotoxic activities of the isolated compounds (1-17) a evaluated by determining their inhibitory effects on human tumor cell lines (A549, SK-OV-3, SK-MEL-2, and HCT15) in vitro using the sulforhodamine B (SRB) assay.24 Compounds 16 and 17 showed moderate cytotoxic activity against the four human tumor cell lines [IC50 (16): 12.57, 17.76, 12.92, 13.01 μM, IC50 (17): 12.92, 10.03, 7.82, 11.08 μM], respectively. But the other compounds were inactive (IC50: >30.0 μM).
EXPERIMENTAL
General. Melting points are determined on a Gallenkamp melting point apparatus and are uncorrected. IR spectra were recorded on a Bruker IFS-66/S FT-IR spectrometer. NMR spectra were recorded on a Varian UNITY INOVA 500 NMR spectrometer operating at 500 MHz (1H) or 125 MHz (13C), with chemical shifts given in ppm (δ). HR-ESI mass spectra were recorded on a SI-2/LCQ DecaXP Liquid chromatography (LC)-mass spectrometer. HR-FAB mass spectra were obtained on a JEOL JMS700 mass spectrometer. GC (Gas Chromatography) was carried out on using a HP-5 capillary column (30 cm × 0.25 mm × 0.25 μm); column temperature, 230 oC; injection temperature, 280 oC; carrier gas, He. Preparative HPLC was performed using a Gilson 306 pump with a Shodex refractive index detector. Chromatographic separation was performed on an Econosil RP-18 10 μ column (250 × 10 mm i.d.). Silica gel 60 (Merk Co., 70 - 230 mesh), RP-C18 silica gel (YMC GEL ODS-A, 12 nm, S-75 μm) and Sephadex LH-20 (Pharmacia) were used for column chromatography. TLC was performed using Merck pre-coated Silica gel F254 plates and RP-18 F254s plates. Low-pressure liquid chromatography was performed over a LiChroprep Lobar-A RP-18 (240 × 10 mm i.d.) column with a FMI QSY-O pump (ISCO).
Plant material. The leaves and trunks of Ilex cornuta (5.0 kg) were collected on Jeju Island, Korea in March 2011, and the plant was identified by one of the authors (K.R.Lee). A voucher specimen (SKKU-NPL 1108) has been deposited at the herbarium in the School of Pharmacy, Sungkyunkwan University, Suwon, Korea.
Extraction and isolation. The leaves and trunks of I. cornuta (5.0 kg) were extracted with 80% aqueous MeOH under reflux and filtered. The resulting MeOH extracts (550 g) were suspended in distilled water (800 mL × 3) and then successively partitioned with n-hexane, CH2Cl2, EtOAc and n-BuOH, yielding 20, 68, 28 and 100 g, respectively. The EtOAc soluble fraction (28 g) was chromatographed on a silica gel (230–400 mesh, 600 g) column eluted with CHCl3 - MeOH (40:1 – 1:1, gradient system) to yield eleven fractions (E1–E11). Fraction E1 (7 g) was loaded on a Sephadex LH-20 column (450 g, 3 × 90 cm) and eluted with CH2Cl2 – MeOH (1:1, 2 L) to yield three subfractions E11 - 13. Subfraction E12 (5 g) was purified by semi-preparative reversed-phase HPLC using a 250 mm × 10 mm i.d., 10 μm, Econosil RP-18 column (Alltech) with a solvent system of 90% MeOH (2 L, flow rate; 2 mL/min) to give 15 (2 g), 16 (20 mg), and 17 (4 mg). Fraction E6 (500 mg) was loaded on a Sephadex LH–20 column (450 g, 3 × 90 cm) and eluted with CH2Cl2 – MeOH (1 : 1) to yield four subfractions E61 – E64. Subfraction E63 (30 mg) was separated by semi-preparative reversed-phase HPLC with a solvent system of 50% MeOH (1 L, flow rate; 2 mL/min) to give 6 (10 mg). Fraction E7 (5 g) was chromatographed on an RP–C18 silica gel (230-400 mesh, 200 g) column chromatography (200 g, 3 × 30 cm) using a solvent system of 70% MeOH (3 L) to give five subfractions (E71–E75). Subfraction E73 (250 mg) was purified by semi-preparative reversed-phase HPLC with a solvent system of 50% MeCN (1 L, flow rate; 2 mL/min) to give 1 (70 mg), 2 (7 mg), and 10 (10 mg). Subfraction E74 (50 mg) was purified by preparative reverse-phase HPLC (50% MeCN) to give 14 (5 mg). Fraction E8 (5 g) was loaded on a Sephadex LH-20 column (450 g, 3 × 90 cm) and eluted with CH2Cl2- MeOH (1:1, 2 L) to yield four subfractions E81 – E84. Subfractions E82 (3 g) was chromatographed on an RP–C18 silica gel (230-400 mesh, 10 g) column chromatography (200 g, 3 × 30 cm) using a solvent system of 50% MeOH (2.5 L) to give five subfractions (E821–E825). Subfractions E825 (500 mg) was purified by semi-preparative reversed-phase HPLC with a solvent system of 20% MeCN (1 L, flow rate; 2 mL/min) to yield 8 (120 mg), 12 (50 mg), and 13 (15 mg). Fraction E9 (850 mg) was chromatographed on an LiChroprep Lobar–B RP–18 column (using 40% MeOH as eluant) and then purified by semi-preparative reversed-phase HPLC with a solvent system of 30% MeCN (1 L, flow rate; 2 mL/min) to yield 3 (7 mg), 4 (8 mg), and 5 (15 mg). Fraction E10 (1 g) was loaded on a Sephadex LH–20 column (200 g, 3 × 30 cm) and eluted with CH2Cl2 – MeOH (1:1, 2 L) to yield two subfractions E101 – 102. Subfractions E101 (70 mg) was purified by by semi-preparative normal-phase HPLC using a solvent system of CHCl3/MeOH (7:1, 2 L, flow rate: 2 mL/min) to yield 9 (12 mg) and 11 (20 mg). Subfractions E102 (50 mg) was purified by semi-preparative reversed-phase HPLC with a solvent system of 40% MeOH (1 L, flow rate; 2 mL/min) to afford 7 (7 mg).
Compound (1): a colorless gum; [α] +35.0 (c=2.0, MeOH); IR (KBr) νmax 3359, 2945, 2833, 1666, 1452, 1032, 649 cm-1; 1H-NMR data, see Table 2; 13C-NMR data, see Table 1; FABMS m/z 643 [M + Na]+; HRFABMS m/z 643.3825 [M + Na]+; (calcd. for C35H56O9Na 643.3822).
Compound (2): a colorless gum; [α] +20.5 (c=0.3, MeOH); IR (KBr) νmax 3383, 2944, 2834, 1748, 1650, 1453, 1261, 1032, 652 cm-1; 1H-NMR data, see Table 2; 13C-NMR data, see Table 1; HRESI-MS m/z 831.4500 [M + Na]+ (calcd. for C43H68NaO14, 831.4507).
Compound (3): a colorless gum; [α] -20.0 (c=0.2, MeOH); IR (KBr) νmax 3384, 2945, 2833, 1735, 1658, 1452, 1032, 655 cm-1; 1H-NMR data, see Table 2; 13C-NMR data, see Table 1; HRESI-MS m/z 643.3820 [M + Na]+ (calcd for C35H56O9Na, 643.3822).
Compound (4): a colorless gum; [α] -30.0 (c=0.4, MeOH); IR (KBr) νmax 3383, 2944, 2834, 1736, 1650, 1453, 1032, 652 cm-1; 1H-NMR data, see Table 2; 13C-NMR data, see Table 1; HRESI-MS m/z 803.4190 [M + Na]+ (calcd. for C41H64O14Na 803.4194).
Acid hydrolysis of compound 1-4 and sugar determination. Compound 1 (2mg) was dissolved in 3 mL of 1 N HCl, respectively. The solution was heat at 90 oC for 1 h. The hydrolysis, this reaction mixture was diluted with H2O and extracted with CHCl3. The H2O layer was dried in vacuo, and stirred with pyridine (0.1 mL) and L-cysteine methyl ester hydrochloride (2.0 mg). The mixture was stirred at 60 oC for 1.5 h. After the reaction mixture was dried in vacuo, the residue was trimethylsilylated with 1-trimethylsilylimidazole (0.1 mL) for 2 h. The mixture was partitioned between n-hexane and H2O (0.3 mL, each), and the organic layer (1 μL) was analyzed by GC.25,26 Compounds 2-4 (each, 2 mg) were treated using the same method. D-glucose and L-arabinose were treated in the same manner. L-arabinose (1 : 5.42 min, 3 : 5.45 min) was detected in 1, and 3. D-glucose (2 : 9.74 min, 4 : 9.77 min) and L-arabinose (2 : 5.41 min, 4 : 5.44) were detected in 2, and 4. Identification of D-glucose (9.76 min) and L-arabinose (5.48 min) were detected in each case by co-injection of the hydrolysate with standard silylated sugars.
Cytotoxicity Assay. A sulforhodamine B bioassay (SRB) was used to determine the cytotoxicity of the above 17 compounds. The cytotoxic activity of each compound against four cultured human tumor cells was examined in vitro at the Korea Research Institute of Chemical Technology. The tumor cell lines were A549 (non small cell lung adenocarcinoma), SK-OV-3 (ovarian cancer cells), SK-MEL-2 (skin melanoma) and HCT15 (colon cancer cells).24 Doxorubicin was used as the positive control. IC50 values for the cytotoxicity of etoposide were 0.25, 1.73, 0.14, and 3.36 μM to A549, SK-OV-3, SK-MEL-2, and HCT15 cells, respectively.
ACKNOWLEDGEMENTS
This research was supported by the Basic Science Research Program through the National Research Foundation of Korea (NRF) funded by the Ministry of Education, Science and Technology (2012R1A5A2A28671860). We are thankful to the Korea Basic Science Institute (KBSI) for the measurements of MS spectra.
References
1. Jiangsu New Medical College; Dictionary of Chinese Herbal Medicines; Shanghai Science and Technology Press, Shanghai, 1986, 1521.
2. S. W. Gau, C. C. Chen, Y. P. Chen, and H. Y. Hsu, J. Chin. Chem. Soc., 1983, 30, 185.
3. Y. Li, T. Wu, Z. H. Cheng, and Z. T. Wang, Chin. J. Chem., 2006, 24, 577. CrossRef
4. T. Nakanishi, H. Terai, M. Nasu, I. Miura, and K. Yoneda, Phytochemistry, 1982, 21, 1373. CrossRef
5. W. J. Qin, X. Wu, J. J. Zhao, Y. Fukuyama, T. Yamada, and K. Nakagawa, Phytochemistry, 1986, 25, 913. CrossRef
6. K. Nishimura, T. Miyase, H. Noguchi, and X. M. Chen, Nat. Med., 2000, 54, 297.
7. T. Wu, Y. Li, Q. J. Tang, and Z. T. Wang, Food Chem., 2008, 111, 78. CrossRef
8. S. Y. Lee, K. H. Kim, and K. R. Lee, Can. J. Chem., 2013, 91, 382. CrossRef
9. A. T. C. Taketa, E. Breitmaier, and E. P. Schenkel, J. Braz. Chem. Soc., 2004, 15, 205. CrossRef
10. K. Hidaka, M. Ito, Y. Matsuda, H. Kohida, K. Yamasaki, J. Yamahara, T. Chisaka, Y. Kawakami, T. Sato, and K. Kagei, Chem. Pharm. Bull., 1987, 35, 524. CrossRef
11. K. Hidaka, M. Ito, Y. Matsuda, H. Kohida, K. Yamasaki, J. Yamahara, T. Chisaka, Y. Kawakami, T. Sato, and K. Kagei, Chem. Pharm. Bull., 1987, 35, 524. CrossRef
12. A. T. C. Taketa, T. Shcmittmann-Schlager, D. Guillaume, G. Gosmann, and E. P. Schenkel, Phytochemistry, 2000, 53, 901. CrossRef
13. A. T. C. Taketa, T. Shcmittmann-Schlager, D. Guillaume, G. Gosmann, and E. P. Schenkel, Phytochemistry, 2000, 53, 901. CrossRef
14. S. Y. Lee, S. U. Choi, and K. R. Lee, Bull. Korean Chem. Soc., 2011, 32, 3813. CrossRef
15. T. Kakuno, K. Yoshikawa, and S. Arihara, Tetrahedron Lett., 1991, 32, 3535. CrossRef
16. T. Kakuno, K. Yoshikawa, S. Arihara, M. Takei, and K. Endo, Tetrahedron, 1991, 47, 7219. CrossRef
17. Y. Zhang, L. J. Li, P. Zhang, H. F. Pi, H. L. Ruan, and J. Z. Wu., Helv. Chim. Acta, 2011, 94, 2207. CrossRef
18. M. R. Cases, A. S. Cerezo, and C. A. Stortz, Carbohydr. Res., 1995, 269, 333. CrossRef
19. Y. Lei, S. P. Shi, Y. L. Song, D. Bi, and P. F. Tu, Chem. Biodivers., 2014, 11, 767. CrossRef
20. I. Yano, C. Nishizumi, K. Yoshikawa, and S. Arihara, Phytochemistry, 1993, 32, 417. CrossRef
21. K. Amimoto, K. Yoshikawa, and S. Arihara, Chem. Pharm. Bull., 1992, 40, 3138. CrossRef
22. N. H. Tung, T. H. Quang, N. T. T. Ngan, C. V. Minh, B. K. Anh, P. Q. Long, N. M. Cuong, and Y. H. Kim, Chem. Pharm. Bull., 2011, 59, 1417. CrossRef
23. I. K. Lee, D. H. Kim, S. Y. Lee, K. R. Kim, S. U. Choi, J. K. Hong, J. H. Lee, Y. H. Park, and K. R. Lee, Arch. Pharm. Res., 2008, 31, 1578. CrossRef
24. P. Skehan, R. Stroreng, D. Scudiero, A. Monks, J. Mcmahon, D. Vistica, J. T. Warren, H. Bokesch, S. Kenney, and M. R. Boyd, J. Nat. Cancer Inst., 1990, 82, 1107. CrossRef
25. G. B. Xie, J. Zheng, P. F. Tu, and G. Q. Liu, Helv. Chim. Acta, 2008, 91, 1630. CrossRef
26. L. Wang, Y. Cai, X. Q. Zhang, C. L. Fan, Q. W. Zhang, and X. P. Lai, Carbohydr. Res., 2012, 329, 39. CrossRef