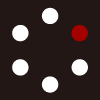
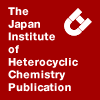
HETEROCYCLES
An International Journal for Reviews and Communications in Heterocyclic ChemistryWeb Edition ISSN: 1881-0942
Published online by The Japan Institute of Heterocyclic Chemistry
e-Journal
Full Text HTML
Received, 4th February, 2015, Accepted, 24th March, 2015, Published online, 2nd April, 2015.
DOI: 10.3987/COM-15-13205
■ Organocatalytic Regioselective Three-Component, One-Pot Allylic Substitution of Morita-Baylis-Hillman Carbonates
Lin Jiang,* Jiang-Feng Zhou, Fei Li, Yong-Gen Li, Hong-Li Li, Yong-Ming Chuan, and Ming-Long Yuan*
Key Laboratory of Chemistry in Ethnic Medicinal Resources, State Ethnic Affairs Commission & Ministry of Education, Engineering Research Center of Biopolymer Functional Materials, Yunnan Minzu University, 134 Yi Er Yi Avenue, Kunming, Yunnan, 650031, China
Abstract
A Lewis base mediated three-component, one-pot allylic substitution reaction of Morita-Baylis-Hillman (MBH) carbonates with water and 1-phenyl-5-methylsulfonyltetrazole (PTSO2Me) has been developed. In the presence of 1,4-diazabicyclo[2.2.2]octane (DABCO), a series of 1-phenyl-5-functionalized tetrazoles are prepared in moderate to excellent yield (40-97%). The key reaction mechanism is rationalized by the ipso-substitution of PTSO2Me with hydroxide which derives from deprotonation of water.Morita-Baylis-Hillman (MBH) adducts or their derivatives are valuable synthetic intermediates since they provide versatile functionalities for further synthetic transformations.1 Recently, the allylic substitution reactions of MBH acetates or carbonates as electrophiles, through a SN2’-SN2’ cascade, by metal-free catalysis of nucleophilic tertiary amines or phosphines have emerged as a very powerful strategy for the construction of densely functionalized compounds in a rapid and atom-economic way and under mild reaction conditions.2 Since the pioneering work conducted by Lu and co-workers,3 the high synthetic potential of MBH carbonates has been actively investigated with recognition of the much superior reactivity of tert-butoxycarbonyl (Boc) group, and thus allowing the application of a variety of nucleophiles.4 In 2002, Kim and co-workers reported the use of cinchona alkaloid derivatives for the hydrolysis of MBH acetate with sodium bicarbonate as a water surrogate.5 This reaction followed the kinetic resolution route and afforded MBH alcohols in low yield (generally less than 50%). By contrast, Jiang and co-workers developed a similar reaction by using MBH carbonates and water as reactants in the presence of a Lewis basic catalyst, which greatly improved the yield of MBH alcohols (up to 99%).6 The latter represented the only example of organocatalytic transformation of MBH derivatives with water as a nucleophile (Scheme 1, a).7
Tetrazoles are known to be popular functionalities with a broad range of synthetic and medicinal chemistry applications,8 therefore, the direct introduction of tetrazole segments into multi-functionalized molecules is highly desirable.9 Koldobskii and co-workers have presented the synthesis of 1-aryl-5-functionally substituted tetrazoles upon reactions of 1-aryl-5-methylsulfonyltetrazoles with diverse C-, N-, and O-nucleophiles (Scheme 1, b).10 In consideration of the mechanisms of ipso-substitution reaction of alkyl sulfonyl-substituted heteroaromatic compounds with nucleophiles11 and allylic substitution of MBH carbonates with water,6 we envisage that, under the catalysis of a Lewis base, presumably, the tert-butoxide released from the decarboxylation of leaving group would deprotonate water firstly, and then the hydroxide serves as a nucleophile to react with 1-phenyl-5-methylsulfonyltetrazole (PTSO2Me). Subsequently, the newly generated anion of 1-phenyl-1H-tetrazol-5-ol would attack the intermediate to deliver allylic substitution products (Scheme 1, c). Herein, we would like to describe the first Lewis base catalyzed three-component, one-pot allylic substitution of MBH carbonates with water and PTSO2Me.
Initially, 3.0 equiv. of MBH carbonate 1a was reacted with 1.0 equiv. of water and 1.0 equiv. of PTSO2Me 2 in the presence of a Lewis base (30 mol%) in 1,2-dichloroethane (DCE) at 40 oC. Among the Lewis bases tested, 1,4-diazabicyclo[2.2.2]octane (DABCO) performed as the most favourable catalyst, furnishing allylic substitution product 3a in 83% isolated yield (Table 1, entry 1), whereas all of 4-dimethylaminopyridine (DMAP), 1,8-diazabicyclo[5.4.0]undec-7-ene (DBU) and triethylamine showed inferior catalytic activity (Table 1, entries 2-4). As was expected, no desired product was afforded when MBH acetate 1b was employed (Table 1, entry 5). In order to further optimize the reaction, more parameters were screened. Several other solvents were examined under the catalysis of 30 mol% of DABCO (Table 1, entries 6-11), and DCE was still the best choice with regard to both reaction rate and yield. Furthermore, the product yield could be improved to 94% on condition that 2.0 equiv. of water was used (Table 1, entry 12). However, the yield was dramatically ruined when adding less than 3.0 equiv. of MBH carbonate (Table 1, entry 13). In addition, decreasing the catalytic loading to 10 mol% seemed to exhibit no obvious influence on reaction yield, except for longer reaction time (Table 1, entry 14). Pleasingly, when the reaction was catalyzed by 10 mol% of DABCO at room temperature, the product yield was slightly improved, though the reaction time became a little more sluggish (Table 1, entry 15).
After screening of reaction conditions, it can be concluded that the optimized reaction should be carried out by using 1.0 equiv. of PTSO2Me 2, 2.0 equiv. of water, 3.0 equiv. of MBH carbonate 1 and 10 mol% of DABCO in 1 mL of DCE at room temperature. With the optimized reaction conditions in hand, we subsequently investigated the scope and limit of the DABCO-mediated three-component, one-pot allylic substitution of MBH carbonates. As is explicated in Table 2, in general, the allylic substitution proceeded well and tetrazole-containing products 3 were generated in moderate to excellent yield (40-97%) (Table 2, entries 1-11). Although the variation in the substitution pattern on the phenyl unit was generally tolerated, those substrates equipped with electron-rich phenyl rings (1g, 1h) provided higher product yield as compared with their electron-deficient counterparts (1c-1f) (80-90% vs 40-68%), nevertheless, longer reaction time was required for complete conversion (Table 2, entries 6-7 vs entries 2-5). MBH carbonate possessing a 1-naphthyl group (1i) was also smoothly converted, rendering product 3i in 80% yield (Table 2, entry 8). In addition, heteroaromatic MBH carbonates 1j and 1k showed relatively lower reactivity, providing good product yield (71% and 73%, respectively) after a longer period of reaction time (Table 2, entries 9-10). It was noteworthy that the MBH carbonate 1l, which was prepared from aliphatic aldehyde, could be successfully utilized in this transformation, albeit giving modest yield due to some side reactions (Table 2, entry 11). Unfortunately, we observed that unwanted side reactions became dominant when MBH carbonate deriving from acrylonitrile or methyl vinyl ketone was applied. Meanwhile, in an attempt to extend the scope of tetrazole-containing substrate, it was found that 1-methyl-5-methylsulfonyltetrazole was not susceptible to the nucleophilic attack by hydroxide under our reaction conditions, and only corresponding MBH alcohols were detected.
In conclusion, we have developed a three-component, one-pot allylic substitution of MBH carbonates with water and PTSO2Me promoted by DABCO. This protocol enables the straightforward and mild synthesis of various 1-phenyl-5-functionalized tetrazole derivatives. Besides, this study has provided one of the rare examples of water-involved transformations of MBH derivatives, and to the best of our knowledge, it is the first report on organocatalytic allylic substitution of MBH carbonates in a three-component, one-pot manner. Currently, an investigation of expanding the substrate scope is in progress and the results will be reported in due course.
EXPERIMENTAL
NMR spectra were recorded with tetramethylsilane as the internal standard. TLC was performed on glass-backed silica plates. Flash column chromatography was performed using silica gel (200-300 mesh) eluting with EtOAc and petroleum ether. UV light and I2 were used to visualize products. 1H NMR spectra were recorded at 400 MHz, and 13C NMR spectra were recorded at 100 MHz (Bruker Avance). Chemical shifts are reported in ppm downfield from CDCl3 (δ = 7.27 ppm) for 1H NMR and relative to the central CDCl3 resonance (δ = 77.0 ppm) for 13C NMR spectroscopy. Coupling constants are given in Hz. In experiments requiring dry solvents, DCE, DCM, CHCl3 and toluene were distilled from CaH2. PhCF3 was stored over 4Å molecular sieves. THF was dried over sodium metal. Acetonitrile was dried over P2O5. All other chemicals were used without purification as commercially available unless otherwise noted.
Starting materials. MBH carbonates12 and 1-phenyl-5-methylsulfonyltetrazole13 were prepared by previously reported procedures.
Typical procedure for preparation of tetrazole-containing MBH derivatives 3. MBH carbonate 1 (0.3 mmol), 1-phenyl-5-methylsulfonyltetrazole 2 (22.4 mg, 0.1 mmol), water (3.6 L, 0.2 mmol) and DABCO (1.1 mg, 10 mol%) in dry DCE (1 mL) were stirred at room temperature and the reaction was monitored by TLC analysis. After completion, the reaction mixture was directly purified by flash column chromatography on silica gel (petroleum ether/EtOAc) to give the tetrazole-containing product 3.
Methyl 2-{phenyl[(1-phenyl-1H-tetrazol-5-yl)oxy]methyl}acrylate (3a). Colourless liquid; 97% yield; 1H NMR (400 MHz, CDCl3): δ = 7.97 (d, J = 8.0 Hz, 2H), 7.50-7.46 (m, 2H), 7.44-7.39 (m, 4H), 7.38-7.32 (m, 2H), 6.66 (d, J = 1.2 Hz, 1H), 6.54 (s, 1H), 5.72 (d, J = 1.6 Hz, 1H), 3.75 (s, 3H) ppm; 13C NMR (100 MHz, CDCl3): δ = 164.2, 147.3, 136.4, 134.1, 133.6, 129.3, 128.3, 127.9, 127.8, 127.2, 126.6, 118.0, 57.5, 51.4 ppm; ESI-HRMS: calcd. for C18H16N4O3+Na 359.1120, found 359.1127.
Methyl 2-{(2-chlorophenyl)[(1-phenyl-1H-tetrazol-5-yl)oxy]methyl}acrylate (3c). Colourless liquid; 61% yield; 1H NMR (400 MHz, CDCl3): δ = 7.98 (d, J = 7.6 Hz, 2H), 7.51-7.49 (m, 2H), 7.47-7.44 (m, 1H), 7.40-7.36 (m, 2H), 7.35-7.31 (m, 2H), 6.94 (s, 1H), 6.67 (s, 1H), 5.60 (d, J=1.2 Hz, 1H), 3.77 (s, 3H) ppm; 13C NMR (100 MHz, CDCl3): δ = 164.0, 147.2, 135.2, 133.7, 132.8, 132.2, 129.1, 128.4, 128.1, 126.7, 126.2, 118.0, 54.7, 51.5 ppm; ESI-HRMS: calcd. for C18H15ClN4O3+Na 393.0730, found 393.0739.
Methyl 2-{(4-chlorophenyl)[(1-phenyl-1H-tetrazol-5-yl)oxy]methyl}acrylate (3d). Colourless liquid; 68% yield; 1H NMR (400 MHz, CDCl3): δ = 7.95 (d, J = 8.8 Hz, 2H), 7.51-7.47 (m, 2H), 7.38 (m, 5H), 6.65 (s, 1H), 6.50 (s, 1H), 5.73 (s, 1H), 3.75 (s, 3H) ppm; 13C NMR (100 MHz, CDCl3): δ = 165.1, 148.3, 137.2, 135.0, 134.6, 133.8, 130.1, 129.8, 129.4, 129.2, 127.8, 119.2, 58.0, 52.5 ppm; ESI-HRMS: calcd. for C18H15ClN4O3+Na 393.0730, found 393.0735.
Methyl 2-{(3-bromophenyl)[(1-phenyl-1H-tetrazol-5-yl)oxy]methyl}acrylate (3e). Yellow liquid; 65% yield; 1H NMR (400 MHz, CDCl3): δ = 7.92 (d, J = 8.4 Hz, 2H), 7.53 (m, 1H), 7.48-7.43 (m, 3H), 7.35-7.31 (m, 2H), 7.26-7.22 (m, 1H), 6.64 (d, J = 0.4 Hz, 1H), 6.45 (s, 1H), 5.71 (d, J = 1.6 Hz, 1H), 3.72 (s, 3H) ppm; 13C NMR (100 MHz, CDCl3): δ = 165.0, 148.3, 137.5, 137.0, 134.6, 132.1, 131.4, 130.5, 129.4, 127.8, 127.0, 123.0, 119.2, 57.9, 52.6 ppm; ESI-HRMS: calcd. for C18H15BrN4O3+H 415.0406, found 415.0402.
Methyl 2-{(3,4-dichlorophenyl)[(1-phenyl-1H-tetrazol-5-yl)oxy]methyl}acrylate (3f). Yellow liquid; 40% yield; 1H NMR (400 MHz, CDCl3): δ = 7.96-7.93 (m, 2H), 7.53 (d, J = 2.4 Hz, 1H), 7.51-7.47 (m, 3H), 7.39-7.35 (m, 1H), 7.29-7.27 (m, 1H), 6.68 (d, J = 0.8 Hz, 1H), 6.47 (s, 1H), 5.77 (d, J = 1.6 Hz, 1H), 3.77 (s, 3H) ppm; 13C NMR (100 MHz, CDCl3): δ = 164.9, 148.2, 136.7, 135.4, 134.5, 133.4, 133.3, 131.0, 130.4, 130.2, 129.4, 127.9, 127.7, 119.2, 57.5, 52.6 ppm. ESI-HRMS: calcd. for C18H14Cl2N4O3+Na 427.0341, found 427.0343.
Methyl 2-{[(1-phenyl-1H-tetrazol-5-yl)oxy](m-tolyl)methyl}acrylate (3g). Colourless liquid; 90% yield; 1H NMR (400 MHz, CDCl3): δ = 7.86 (d, J = 8.0 Hz, 2H), 7.39-7.35 (m, 2H), 7.26-7.22 (m, 1H), 7.20-7.15 (m, 1H), 7.11-7.05 (m, 3H), 6.54 (s, 1H), 6.38 (s, 1H), 5.62 (s,1H), 3.64 (s, 3H), 2.25 (s, 3H) ppm; 13C NMR (100 MHz, CDCl3): δ = 164.3, 147.3, 137.7, 136.5, 134.1, 133.7, 129.3, 128.6, 128.3, 127.9, 127.8, 126.6, 124.3, 118.1, 57.6, 51.4, 20.4 ppm; ESI-HRMS: calcd. for C19H18N4O3+H 351.1457, found 351.1455.
Methyl 2-{[(1-phenyl-1H-tetrazol-5-yl)oxy](p-tolyl)methyl}acrylate (3h). Colourless liquid; 80% yield; 1H NMR (400 MHz, CDCl3): δ = 7.96 (d, J = 8.0 Hz, 2H), 7.50-7.46 (m, 2H), 7.37-7.33 (m, 1H), 7.31 (d, J = 8.0 Hz, 2H), 7.21 (d, J = 8.0 Hz, 2H), 6.64 (s, 1H), 6.49 (s, 1H), 5.72 (d, J = 1.6 Hz, 1H), 3.75 (s, 3H), 2.35 (s, 3H) ppm; 13C NMR (100 MHz, CDCl3): δ= 164.3, 147.3, 137.8, 136.5, 133.7, 131.1, 129.0, 128.6, 128.3, 127.2, 126.6, 118.1, 57.4, 51.4, 20.2 ppm; ESI-HRMS: calcd. for C19H18N4O3+H 351.1457, found 351.1451.
Methyl 2-{naphthalen-1-yl[(1-phenyl-1H-tetrazol-5-yl)oxy]methyl}acrylate (3i). Colourless liquid; 80% yield; 1H NMR (400 MHz, CDCl3): δ = 8.04 (d, J = 8.4 Hz, 1H), 7.98 (d, J = 8.0 Hz, 2H), 7.91-7.88 (m, 2H), 7.60-7.54 (m, 2H), 7.53-7.47 (m, 4H), 7.38-7.34 (m, 2H), 6.70 (s, 1H), 5.70 (d, J= 1.2 Hz, 1H), 3.76 (s, 3H) ppm; 13C NMR (100 MHz, CDCl3): δ = 164.3, 147.3, 136.0, 133.7,132.9,130.2,129.6,129.3,128.7, 128.4, 128.0, 126.7, 126.2, 125.2, 124.9, 124.1, 121.7, 118.1, 53.8, 51.5 ppm; ESI-HRMS: calcd. for C22H18N4O3+H 387.1457, found 387.1457.
Methyl 2-{[(1-phenyl-1H-tetrazol-5-yl)oxy](thiophen-2-yl)methyl}acrylate (3j). Yellow liquid; 71% yield; 1H NMR (400 MHz, CDCl3): δ = 7.96 (d, J = 8.8 Hz, 2H), 7.51-7.47 (m, 2H), 7.38-7.35 (m, 2H), 7.16 (d, J = 3.6 Hz, 1H),7.03-7.01 (m, 1H), 6.81 (s, 1H), 6.66 (s, 1H), 5.97 (s, 1H), 3.78 (s, 3H) ppm; 13C NMR (100 MHz, CDCl3): δ = 164.0, 146.9, 136.4, 133.6, 128.9, 128.4, 127.1, 126.7, 126.1, 125.9, 118.1, 99.0, 52.8, 51.5 ppm; ESI-HRMS: calcd. for C16H14N4O3S+Na 365.0684, found 365.0688.
Methyl 2-{[(1-phenyl-1H-tetrazol-5-yl)oxy](furan-2-yl)methyl}acrylate (3k). Yellow liquid; 73% yield; 1H NMR (400 MHz, CDCl3): δ = 7.96 (d, J = 8.0 Hz, 2H), 7.51-7.49 (m, 2H), 7.47-7.45 (m, 1H), 7.38-7.35 (m, 1H), 6.68 (d, J = 0.4 Hz, 1H), 6.64 (s, 1H), 6.42-6.39 (m, 2H), 5.95 (s, 1H), 3.79 (s, 3H) ppm; 13C NMR (100 MHz, CDCl3): δ = 163.9, 146.9, 142.6, 134.3, 133.6, 129.4, 128.4, 126.7, 118.2, 109.7, 109.4, 51.5, 51.4 ppm; ESI-HRMS: calcd. for C16H14N4O4+Na 349.0913, found 349.0919.
Methyl 2-methylene-3-[(1-phenyl-1H-tetrazol-5-yl)oxy]hexanoate (3l). Colourless liquid; 40% yield; 1H NMR (400 MHz, CDCl3): 7.97 (d, J = 8.4 Hz, 2H), 7.51-7.47 (m, 2H), 7.38-7.34 (m, 1H), 6.51 (s, 1H), 5.96 (d, J = 0.8 Hz, 1H), 5.36 (dd, J = 8.0 Hz, 4.8 Hz, 1H), 3.79 (s, 3H), 2.78-2.18 (m, 1H), 2.07-1.98 (m, 1H), 0.97 (t, J = 7.2 Hz, 3H), 0.88-0.84 (m, 2H) ppm; 13C NMR (100 MHz, CDCl3): 165.6, 148.7, 138.0, 134.8, 129.4, 128.0, 127.6, 119.1, 54.8, 52.4, 34.6, 19.5, 13.4 ppm; ESI-HRMS: calcd. for C15H18N4O3+H 303.1457, found 303.1450.
ACKNOWLEDGEMENTS
This work was supported by the National Natural Science Foundation of China (21302163), Scientific Research Foundation of Yunnan Education Office of China (2013Z037), Youth Scientific Research Foundation of Yunnan Minzu University (2012QN03) and Graduate Students Innovation Foundation of Yunnan Minzu University (2014YJY68).
References
1. D. Basavaiah, B. S. Reddy, and S. S. Badsara, Chem. Rev., 2010, 110, 5447; CrossRef Y. Wei and M. Shi, Acc. Chem. Res., 2010, 43, 1005; CrossRef V. Declerck, J. Martinez, and F. Lamaty, Chem. Rev., 2009, 109, 1. CrossRef
2. T.-Y. Liu, M. Xie, and Y.-C. Chen, Chem. Soc. Rev., 2012, 41, 4101; CrossRef R. Rios, Catal. Sci. Technol., 2012, 2, 267; CrossRef Y. Wei and M. Shi, Chem. Rev., 2013, 113, 6659. CrossRef
3. Y. Du, X. Han, and X. Lu, Tetrahedron Lett., 2004, 45, 4967. CrossRef
4. G. Zhan, Q.-Q. Zhou, W. Du, and Y.-C. Chen, Synthesis, 2014, 46, 3383; CrossRef H.-B. Yang, Y.-Z. Zhao, R. Sang, and M. Shi, J. Org. Chem., 2014, 79, 3519; CrossRef H. Mao, A. Lin, Y. Shi, Z. Mao, X. Zhu, W. Li, H. Hu, Y. Cheng, and C. Zhu, Angew. Chem. Int. Ed., 2013, 52, 6288; CrossRef Q.-G. Wang, Q.-Q. Zhou, J.-G. Deng, and Y.-C. Chen, Org. Lett., 2013, 15, 4786. CrossRef
5. J. N. Kim, H. J. Lee, and J. H. Gong, Tetrahedron Lett., 2002, 43, 9141. CrossRef
6. B. Zhu, L. Yan, Y. Pan, R. Lee, H. Liu, Z. Han, K.-W. Huang, C.-H. Tan, and Z. Jiang, J. Org. Chem., 2011, 76, 6894. CrossRef
7. H. Zhu, P. Chen, and G. Liu, J. Am. Chem. Soc., 2014, 136, 1766; CrossRef J. Piera, A. Persson, X. Caldentey, and J.-E. Bäckvall, J. Am. Chem. Soc., 2007, 129, 14120. CrossRef
8. R. N. Bulter, 'Comprehensive Heterocyclic Chemistry: tetrazoles', Vol. 5, ed. by A. R. Katritzky and C. W. Rees, Pergamon, Oxford, 1984, pp. 791-838; M. Hiskey, D. E. Chavez, D. L. Naud, S. F. Son, H. L. Berghout, and C. A. Bome, Proc. Int. Pyrotech. Semin., 2000, 27, 3; T. Schelenz and W. J. Schafer, J. Prakt. Chem., 2000, 342, 91. CrossRef
9. P. Mani, C. Sharma, S. Kumar, and S. K. Awasthi, J. Mol. Catal. A: Chem., 2014, 392, 150; CrossRef A. Coca and E. Turek, Tetrahedron Lett., 2014, 55, 2718. CrossRef
10. R. V. Kharbash, L. V. Alam, A. P. Koreneva, and G. I. Koldobskii, Chem. Heterocycl. Compd., 2001, 37, 1493; CrossRef R.V. Kharbash, M. A. Gol’tsberg, T. V. Artamonova, E. Nordlander, and G. I. Koldobskii, Russ. J. Org. Chem., 2002, 38, 1356. CrossRef
11. D. R. Williams and L. Fu, Org. Lett., 2010, 12, 808; CrossRef J. Y. Park, I. A. Ryu, J. H. Park, D. C. Ha, and Y.-D. Gong, Synthesis, 2009, 913.
12. J. Feng, X. Lu, A. Kong, and X. Han, Tetrahedron, 2007, 63, 6035. CrossRef
13. M.-E. Lebrun, P. L. Marquand, and C. Berthelette, J. Org. Chem., 2006, 71, 2009. CrossRef