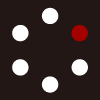
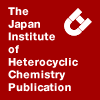
HETEROCYCLES
An International Journal for Reviews and Communications in Heterocyclic ChemistryWeb Edition ISSN: 1881-0942
Published online by The Japan Institute of Heterocyclic Chemistry
e-Journal
Full Text HTML
Received, 11th February, 2015, Accepted, 20th April, 2015, Published online, 11th May, 2015.
DOI: 10.3987/COM-15-13191
■ An Efficient Construction of 4-Oxo-4H-chromene-2-carboxylate Derivatives via One-Pot Cascade Reaction Under Solvent-Free Conditions
Chao Huang,* Jia-Hui Guo, Huang-Mei Fu, Ming-Long Yuan, and Li-Juan Yang
Key Laboratory of Chemistry in Ethnic Medicinal Resources, State Ethnic Affairs Commission & Ministry of Education, Engineering Research Center of Biopolymer Functional Materials, Yunnan Minzu University, 134 Yi Er Yi Avenue, Kunming, Yunnan, 650031, China
Abstract
An efficient synthetic strategy to chromone derivatives from commercially available diethyl acetylenedicarboxylate and phenols via a one-pot cascade reaction has been developed. Performing the reaction using pyridine and polyphosphoric acid as the catalyst at room temperature and 90 oC without any solvent gave the chromone derivatives in good to high yields at one time. A possible reaction pathway was also proposed and supported by the experiment. This protocol is environmentally friendly and metal-free, with advantages including short reaction times, convenient operation, and mild reaction conditions.As oxygen-containing heterocyclic compounds, chromones can be found in a large number of natural products.1 Chromone scaffolds (Figure 1), for example flavones and isoflavones, have a broad range of biological activities such as anti-inflammatory, antiplatelet, anticancer, antiviral, and antimicrobial properties.2 Due to their pharmaceutical applications, recently, relevant reports, which focused on the construction of simple chromones and fused chromones as well as the derivatization and total synthesis, have gradually increased.3 Among these, considerable efforts have been paid for exploring ideal synthetic routes to 4-oxo-4H-chromene-2-carboxylates (Figure 1).
In modern organic chemistry, the ideal synthesis should be a combination of a number of environmental, health, safety, and economic targets, so that meets the principles of green chemistry.4 Accordingly, synthetic methodologies including bicyclic construction, chromanones transformation, and chromones derivatization have been designed.1a Actually, few study has been reported on chromanones transformation, while as for the chromones derivatization has been often used for the formation of functional compounds and fused heterocyclic compounds.5 Compared with the previous reports, bicyclic construction has been frequently employed and could be considered as the most efficient strategy for the construction of simple chromones.6 However, most of the reported methodologies had with limitations, i.e., they are multi-step and typically carried out with metal catalysts, strong acids, or unconventional reagents. Furthermore, it often requires high temperature or pressure that makes the synthesis and isolation of desired products difficult and the yields are low to moderate. Thus, it is highly urgent to develop some facile alternatives with easily available starting materials and under mild reaction conditions for fulfilling the principle of efficient and green synthesis.
In the past few decades, cascade reaction has aroused much attention because of the advantages, viz., atom economy, step-saving, and efficiency in chemical processes by which avoiding the isolation of intermediates and change of reaction conditions.7 It has also been regarded as a powerful tool for total synthesis of natural products and construction of heterocyclic compounds. Stimulated by our prior work,8 we are pleased to introduce the reaction for the construction of chromones. Herein, we design a protocol for the construction of 4-oxo-4H-chromene-2-carboxylate ring via a cascade reaction between commercially available phenols 1 and the diethyl acetylenedicarboxylate 2 (Scheme 1).
At first, we treated 1a with 2 in solvent-free conditions at room temperature and the reaction might proceed by the addition of pyridine as a base; however, the target molecule could not be found by 1H NMR analysis. Then some catalysts were added to the reaction mixture, which were expected to get the 4-oxo-4H-chromene-2-carboxylates in one-pot, and we finally used the concentrated sulfuric acid at last. Next, phenol 1a and diethyl acetylenedicarboxylate 2 were treated to optimize the reaction conditions (Table 1). The reaction could also proceed in different solvents at room temperature (Table 1, entries 2–5), but we could not obtain the products 3a in better yields. In the elevated temperature treatment (at 90 oC), the target product 3 was obtained in the yield of more than 50% under solvent-free conditions (Table 1, entries 6–7). A study on different catalyst such as concentrated sulfuric acid and diphenyl ether was examined, and we could find polyphosphoric acid (PPA) as the best catalyst (Table 1, entries 7–9). Among the basic catalysts, pyridine affords the best result (Table 1, entries 9–11). Thus, we defined the reaction of the phenol 1a with 1.25 equiv of diethyl acetylenedicarboxylate 2, as catalysts by pyridine and PPA, under solvent-free conditions from room temperature to 90 oC as the standard reaction conditions (Table 1, entry 9).
Under the optimal conditions established, the scope of the one-pot cascade reaction without solvent was developed using a range of substituted phenols 1 (Scheme 2). They provided the chromone compounds 3 in good to high yields, and the results are summarized in Scheme 2 and the experimental section. All of the products 3a–3k were characterized by IR, 1H NMR, 13C NMR and the high resolution mass spectrometry (HRMS). The reaction of substituted phenols 1 and 2 proceeded smoothly, however, the phenols with active functional group and β-naphthol were not good for this reaction. The presence of either electron-withdrawing or electron-donating groups on the aromatic ring of phenols 1 played a significant role in the formation of chromones 3. For example, the reactions of 2 and phenols 1b and 1c having the electron-donating substituents proceeded in short time (3 h) and afforded 3b and 3c in high yields (76% and 88%), respectively. However, reversely the reactions of 2 and phenols 1d–1g having the electron-withdrawing substituted proceeded slowly (5 h) and afforded 3d–3g in moderate yields (from 51% to 56%). Moreover, the positional isomers (ortho-, meta-, and para- Me) having same methyl group on phenol ring showed no effect on the yields (from 73% to 76%) of the chromones 3. However, the regioselectivity of intramolecular Friedel-Crafts reaction was observed in the reaction of 2 and meta-substituted phenol such as 1j and 1k, and the yields of major product were above 50% yield.
Under the optimal conditions established, the scope of the one-pot cascade reaction without solvent was developed using a range of substituted phenols 1 (Scheme 2). They provided the chromone compounds 3 in good to high yields, and the results are summarized in Scheme 2 and the experimental section. All of the products 3a–3k were characterized by IR, 1H NMR, 13C NMR and the high resolution mass spectrometry (HRMS). The reaction of substituted phenols 1 and 2 proceeded smoothly, however, the phenols with active functional group and β-naphthol were not good for this reaction. The presence of either electron-withdrawing or electron-donating groups on the aromatic ring of phenols 1 played a significant role in the formation of chromones 3. For example, the reactions of 2 and phenols 1b and 1c having the electron-donating substituents proceeded in short time (3 h) and afforded 3b and 3c in high yields (76% and 88%), respectively. However, reversely the reactions of 2 and phenols 1d–1g having the electron-withdrawing substituted proceeded slowly (5 h) and afforded 3d–3g in moderate yields (from 51% to 56%). Moreover, the positional isomers (ortho-, meta-, and para- Me) having same methyl group on phenol ring showed no effect on the yields (from 73% to 76%) of the chromones 3. However, the regioselectivity of intramolecular Friedel-Crafts reaction was observed in the reaction of 2 and meta-substituted phenol such as 1j and 1k, and the yields of major product were above 50% yield.
In conclusion, we have developed an efficient and facile strategy for the formation of chromone derivatives. The approach used pyridine and PPA as catalysts, easily accessed diethyl acetylenedicarboxylate 2 and simple phenols 1 as the starting materials, and the corresponding chromones 3 were obtained in reasonable yields at one time. This method did not need the aid of any metals and classical solvents. Generally, the advantages of this environmentally benign procedure over earlier reported approaches include its simplicity, fast and clean reactions and high yields. Our further investigation into the application of the strategy for the formation of chromone heterocyclic compounds and chromone drug are currently on-going.
Experimental Section
All compounds were fully characterized by spectroscopic techniques. The NMR spectra were recorded on a Bruker Avance 400 spectrometer at 400 MHz spectrometer (1H: 400 MHz, 13C: 100 MHz) with tetramethylsilane (TMS) as the internal standard (δ 0.0 ppm), chemical shifts (δ) are expressed in ppm, and J values are given in Hz. Deuterated chloroform (CDCl3) was used as a solvent. IR spectra were recorded on a FT-IR Thermo Nicolet Avatar 360 using a KBr pellet. The melting points were determined on an XT-4A melting point apparatus and are uncorrected. Alumina N-neutral (100–200 & 200–300 mesh) for column chromatography and silica GF254 for TLC were produced by Qingdao Marine Chemical Company (China).
Typical Procedure for the Preparation of the Chromone Derivatives 3.
Ethyl 4-oxo-4H-chromene-2-carboxylate (3a). Pyridine (0.50 mmol) was added to a mixture of diethyl acetylenedicarboxylate 2 (1.00 mmol) and phenol 1a (1.25 mmol). The resulting mixture was stirred at room temperature for 10 min. Then, PPA (6.00 mmol) was added and the mixture was heated up to 90 oC for the appropriate amount of time. The process of the reaction was monitored by TLC. And the heating will be stopped when the intermediate from the reaction of 1a and 2 transformed to the new product completely, then the mixture was cooled to room temperature. The residue was purified by column chromatography on silica gel to give 3a (82%); White solid: mp 68–69 C (lit,9 mp 63 °C); IR (KBr) (νmax, cm-1) 3090, 1739, 1651, 1451, 1251, 1119, 1063, 859; 1H NMR (400 MHz, CDCl3): δ 1.20 (t, J = 7.2 Hz, 3H), 4.11 (q, J = 7.2 Hz, 2H), 5.48 (d, J = 12.6 Hz, 1H), 6.98 (m, 1H), 7.09 (t, J = 7.43 Hz, 1H), 7.28 (t, J = 8.0 Hz, 1H), 7.73 (m, 1H); 13C NMR (100 MHz, CDCl3): δ 14.3, 60.0, 102.2, 118.1, 118.1, 125.0, 130.0, 130.0, 155.9, 159.0, 159.0, 167.2; HRMS (ESI-TOF) m/z Calcd for C12H10O4 [M+Na]+ 241.0477, found 241.0471.
Ethyl 6-methyl-4-oxo-4H-chromene-2-carboxylate (3b): Yield 76%; White solid: mp 133–134 C (lit,10 mp 137–139 °C); IR (KBr) (νmax, cm-1) 3080, 1748, 1651, 1616, 1478, 1287, 1238, 1122, 1045, 867, 833, 737; 1H NMR (400 MHz, CDCl3): δ 1.42 (t, J = 7.1 Hz, 3H), 2.45 (s, 3H), 4.45 (q, J = 7.1 Hz, 2H), 7.09 (s, 1H), 7.52 (m, 2H), 7.96 (m, 1H); 13C NMR (100 MHz, CDCl3): δ 14.1, 21.0, 62.9, 114.6, 118.5, 124.0, 125.0, 136.0, 136.1, 152.1, 154.3, 160.6, 178.5; HRMS (ESI-TOF) m/z Calcd for C13H12O4 [M+Na]+ 255.0633, found 240.0627.
Ethyl 6-methoxy-4-oxo-4H-chromene-2-carboxylate (3c): Yield 88%; White solid: mp 102–103 C (lit,9 mp 98 °C); IR (KBr) (νmax, cm-1) 3085, 1741, 1653, 1451, 1249, 1117, 1064, 862; 1H NMR (400 MHz, CDCl3): δ 1.44 (t, J = 7.2 Hz, 3H), 3.9 (s, 1H), 4.47 (q, J = 7.2 Hz, 2H), 7.1 (s, 1H), 7.33 (dd, J = 9.2, 3.2 Hz, 1H), 7.55 (s, 1H); 13C NMR (100 MHz, CDCl3): δ 14.1, 56.0, 63.0, 104.6, 113.9, 115.5, 120.3, 125.0, 150.9, 152.0, 157.5, 160.6, 178.3; HRMS (ESI-TOF) m/z Calcd for C13H12O5 [M+Na]+ 271.0582, found 271.0576.
Ethyl 6-bromo-4-oxo-4H-chromene-2-carboxylate (3d): Yield 53%; White solid: mp 136–137 C (lit,11 mp 143–144 °C); IR (KBr) (νmax, cm-1) 3095, 1739, 1652, 1451, 1250, 1119, 1064, 860; 1H NMR (400 MHz, CDCl3): δ 1.44 (t, J = 7.1 Hz, 3H), 4.49 (q, J = 7.1 Hz, 2H), 7.26 (s, 1H), 7.38 (d, J = 8.0 Hz, 1H), 7.74 (d, J = 8.0 Hz, 1H), 8.48 (s, 1H); 13C NMR (100 MHz, CDCl3): δ 14.2, 63.7, 74.8, 111.9, 119.9, 129.1, 136.4, 136.8, 152.0, 162.8; HRMS (ESI-TOF) m/z Calcd for C12H9BrO4 [M+Na]+ 318.9582, found 318.9576.
Ethyl 6-fluoro-4-oxo-4H-chromene-2-carboxylate (3e): Yield 56%; White solid: mp 128–129 C; IR (KBr) (νmax, cm-1) 3075, 1740, 1652, 1450, 1250, 1118, 1064, 860; 1H NMR (400 MHz, CDCl3): δ 1.37 (t, J = 7.2 Hz, 3H), 4.40 (q, J = 7.2 Hz, 2H), 7.04 (s, 1H), 7.41 (m, 1H), 7.57 (m, 1H), 7.76 (dd, J = 8.0, 3.1 Hz, 1H); 13C NMR (100 MHz, CDCl3): δ 13.0, 62.1, 109.5, 109.7, 112.9, 120.0, 120.1, 121.9, 122.2, 151.4, 159.3, 176.6; HRMS (ESI-TOF) m/z Calcd for C12H9FO4 [M+Na]+ 259.0383, found 259.0377.
Ethyl 4-oxo-6-trifluoromethyl-4H-chromene-2-carboxylate (3f): Yield 53%; White solid: mp 123–124 C; IR (KBr) (νmax, cm-1) 3080, 1740, 1652, 1450, 1250, 1119, 1064, 859; 1H NMR (400 MHz, CDCl3): δ 1.43 (t, J = 7.1 Hz, 3H), 4.45 (d, J = 7.1 Hz, 2H), 7.15 (s, 1H), 7.26 (s, 1H), 7.67 (d, J = 8.9 Hz, 1H), 8.40 (q, J = 4.0 Hz, 1H); 13C NMR (100 MHz, CDCl3): δ 13.1, 60.7, 114.1, 118.2, 123.0, 127.1, 130.8, 134.3, 151.5, 157.3, 159.2, 164.0, 176.9; HRMS (ESI-TOF) m/z Calcd for C13H9F3O4 [M+Na]+ 309.0351, found 309.0345.
Ethyl 6-nitro-4-oxo-4H-chromene-2-carboxylate (3g): Yield 51%; White solid: mp 172–173 C (lit,11 mp 178–179 °C); IR (KBr) (νmax, cm-1) 3075, 1739, 1651, 1450, 1250, 1118, 1064, 858; 1H NMR (400 MHz, CDCl3): δ 1.44 (t, J = 7.0 Hz, 3H), 4.49 (q, J = 7.0 Hz, 2H), 7.17 (s, 1H), 7.78 (d, J = 9.9 Hz, 1H), 8.56 (m, 1H), 9.04 (d, J = 8.0 Hz, 1H); 13C NMR (100 MHz, CDCl3): δ 14.1, 63.5, 115.0, 120.7, 122.5, 124.4, 128.9, 145.2, 152.8, 158.7, 159.8, 176.8; HRMS (ESI-TOF) m/z Calcd for C12H9NO6 [M+Na]+ 286.0328, found 286.0322.
Ethyl 8-methyl-4-oxo-4H-chromene-2-carboxylate (3h): Yield 73%; White solid: mp 101–103 C (lit,11 mp 100–101 °C); IR (KBr) (νmax, cm-1) 3085, 1741, 1653, 1454, 1249, 1115, 1065, 863; 1H NMR (400 MHz, CDCl3): δ 1.35 (t, J = 7.2 Hz, 3H), 2.43 (s, 3H), 4.36 (q, J = 7.2 Hz, 2H), 6.97 (s, 1H), 7.21 (t, J = 7.7 Hz, 1H), 7.45 (d, J = 7.4 Hz, 1H), 7.89 (d, J = 7.9 Hz, 1H); 13C NMR (100 MHz, CDCl3): δ 14.1, 15.5, 62.9, 114.4, 123.2, 124.3, 125.4, 128.4, 135.8, 152.0, 154.4, 160.6, 178.8;HRMS (ESI-TOF) m/z Calcd for C13H12O4 [M+Na]+ 255.0633, found 240.0627.
Ethyl 8-bromo-4-oxo-4H-chromene-2-carboxylate (3i): Yield 69%; White solid: mp 124–125 C (lit,12 mp 125–126 °C); IR (KBr) (νmax, cm-1) 3085, 1740, 1653, 1450, 1250, 1118, 1064, 861; 1H NMR (400 MHz, CDCl3): δ 1.38 (t, J = 7.1 Hz, 3H), 4.41 (q, J = 8.0 Hz, 2H), 7.05 (s, 1H), 7.24 (t, J = 8.0 Hz, 1H), 7.88 (m, 1H), 8.05 (m, 1H); 13C NMR (100 MHz, CDCl3): δ 13.0, 62.1, 111.4, 113.6, 124.0, 124.6, 125.4, 137.1, 151.5, 151.6, 159.0, 176.8; HRMS (ESI-TOF) m/z Calcd for C12H9BrO4 [M+Na]+ 318.9582, found 318.9576.
Ethyl 7-methyl-4-oxo-4H-chromene-2-carboxylate (3j): Yield 76%; White solid: mp 57–58 C (lit,11 mp 57–58 °C); IR (KBr) (νmax, cm-1) 2968, 1754, 1690, 1625, 1440, 1361, 1318, 1222, 1167, 1055, 1013, 927, 854, 792, 736; 1H NMR (400 MHz, CDCl3): δ 1.37 (m, 3H), 2.43 (s, 3H), 4.39 (q, J = 7.1 Hz, 2H), 7.02 (s, 1H), 7.19 (d, J = 8.1 Hz, 1H), 7.35 (s, 1H), 8.00 (d, J = 8.1 Hz, 1H); 13C NMR (100 MHz, CDCl3): δ 14.1, 21.9, 62.9, 114.7, 118.4, 122.1, 125.4, 127.4, 146.3, 151.9, 156.1, 160.6, 178.3; HRMS (ESI-TOF) m/z Calcd for C13H12O4 [M+Na]+ 255.0633, found 240.0627.
Ethyl 7-formyl-4-oxo-4H-chromene-2-carboxylate (3k): Yield 55%; White solid: mp 103–104 C; IR (KBr) (νmax, cm-1) 3075, 1739, 1651, 1450, 1250, 1119, 1064, 857; 1H NMR (400 MHz, CDCl3): δ 1.44 (t, J = 7.1 Hz, 3H), 4.47 (q, J = 7.1 Hz, 2H), 7.82 (s, 1H), 7.83 (d, J = 5.3 Hz, 1H), 7.13 (s, 1H), 7.86 (s, 1H), 11.08 (s, 1H); 13C NMR (100 MHz, CDCl3): δ 13.1, 62.2, 114.6, 122.7, 122.8, 124.5, 133.3, 136.6, 151.0, 155.3, 159.0, 178.5, 191.5; HRMS (ESI-TOF) m/z Calcd for C13H10O5 [M+Na]+ 269.0426, found 269.0420.
Acknowledgements
This work was supported by the National Natural Science Foundation of China (No. 21202142), and the Key Project of Chinese Ministry of Education (No. 212161), Start-up funds of Yunnan Minzu University.
References
1. (a) A. Gaspar, M. J. Matos, J. Garrido, E. Uriarte, and F. Borges, Chem. Rev., 2014, 114, 4960; CrossRef (b) A. M. Edwards and J. B. L. Howell, Clin. Exp. Allergy, 2000, 30, 756; CrossRef (b) A. M. Edwards and J. B. L. Howell, Clin. Exp. Allergy, 2000, 30, 756. CrossRef
2. (a) S. Hua, Front. Pharmacol., 2013, 4, 127; (b) A. Alama, A. M. Orengo, S. Ferrini, and R. Gangemi, Drug Discovery Today, 2012, 17, 435; CrossRef (c) C. Conti, P. Mastromarino, P. Goldoni, G. Portalone, and N. Desideri, Antiviral Chem. Chemother., 2005, 16, 267; CrossRef (d) J. Rai, G. K. Randhawa, and M. Kaur, Int. J. Appl. Basic Med. Res., 2013, 3, 3. CrossRef
3. (a) X. S. Wang, G. L. Cheng, and X. L. Cui, Chem. Commun., 2014, 50, 652; CrossRef (b) V. Eschenbrenner-Lux, P. Kuchler, S. Ziegler, K. Kumar, and H. Waldmann, Angew. Chem. Int. Ed., 2014, 53, 2134; CrossRef (c) S. Vedachalam, Q. L. Wong, B. Maji, J. Zeng, J. M. Ma, and X. W. Liu, Adv. Synth. Catal., 2011, 353, 219; CrossRef (d) N. J. Zhong, L. Liu, D. Wang, and Y. J. Chen, Chem. Commun., 2013, 49, 3697. CrossRef
4. (a) P. A. Wender, Nat. Prod. Rep., 2014, 31, 433; CrossRef (b) P. Anastas and N. Eghbali, Chem. Soc. Rev., 2010, 39, 301; CrossRef (c) M. B. Gawande, V. D. B. Bonifacio, R. Luque, P. S. Branco, and R. S. Varma, Chem. Soc. Rev., 2013, 42, 5522. CrossRef
5. (a) T. Ankner, M. Friden-Saxin, N. Pemberton, T. Seifert, M. Grotli, K. Luthman, and G. Hilmersson, Org. Lett., 2010, 12, 2210; CrossRef (b) M. R. Zanwar, M. J. Raihan, S. D. Gawande, V. Kayala, D. Janreddy, C. W. Kuo, R. Ambre, and C. F. Yao, J. Org. Chem., 2012, 77, 6495. CrossRef
6. (a) J. Zhao, Y. F. Zhao, and H. Fu, Angew. Chem. Int. Ed., 2011, 50, 3769; CrossRef (b) J. P. Lin and Y. Q. Long, Chem. Commun., 2013, 49, 5313. CrossRef
7. J. Barluenga, F. Rodriguez, and F. J. Fananas, Chem. Asian J., 2009, 4, 1036. CrossRef
8. (a) C. Huang, Y. Yin, J. Guo, J. Wang, B. Fan, and L. Yang, RSC Adv., 2014, 4, 10188; CrossRef (b) S. J. Yan, C. Huang, C. X. Su, Y. F. Ni, and J. Lin, J. Comb. Chem., 2010, 12, 91; CrossRef (c) C. Huang, S. J. Yan, Y. M. Li, R. Huang, and J. Lin, Bioorg. Med. Chem. Lett., 2010, 20, 4665; CrossRef (d) S. J. Yan, C. Huang, X. H. Zeng, R. Huang, and J. Lin, Bioorg. Med. Chem. Lett., 2010, 20, 48. CrossRef
9. T. Walenzyk, C. Carola, H. Buchholz, and B. König, Tetrahedron, 2005, 61, 7366. CrossRef
10. W. Huang, Y. Ding, Y. Miao, M. Z. Liu, Y. Li, and G. F. Yang, Eur. J. Med. Chem., 2009, 44, 3687. CrossRef
11. G. P. E. D. Shaw, J. Med. Chem., 1972, 15, 865. CrossRef
12. S. G. D. B. E. Mobbs, J. Chem. Soc., Perkin Trans 1, 1987, 2597.