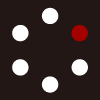
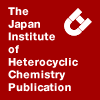
HETEROCYCLES
An International Journal for Reviews and Communications in Heterocyclic ChemistryWeb Edition ISSN: 1881-0942
Published online by The Japan Institute of Heterocyclic Chemistry
e-Journal
Full Text HTML
Received, 19th February, 2015, Accepted, 19th June, 2015, Published online, 6th July, 2015.
DOI: 10.3987/COM-15-13197
■ NIR-Fluorescent Ethyl 4,7-Bis(5-arylthiophen-2-yl)-1,2,5-oxadiazolo[3,4-c]pyridine-6-carboxylate
Kentaro Nishi,* Nobuyuki Seto, Wataru Iwasaki, Yohei Matsuoka, Yuki Kashiwa, Youichi Sano, Tadashi Kawaharada, Takashi Yazumi, Keiji Mizuki, and Shin-ichiro Isobe
Faculty of Engineering, Kyushu Sangyo University , 3-1 Matsukadai, 2-Chome, Higashiku, Fukuoka 813-8503, Japan
Abstract
Ethyl 4,7-bis(5-bromothiophen-2-yl)-1,2,5-oxadiazolo[3,4-c]pyridine-6-carboxylate 2 reacted with phenyl-, 4-methylphenyl-, 4-methoxyphenyl-, 3,4-dimethoxyphenyl-, 1-naphthyl-, and 2-naphthyl-boronic acid to give the corresponding π-extended 4,7-bis(5-arylthiophen-2-yl) derivatives 3a–f, which emitted fluorescence at around 700 nm in DMSO solution. The 4-methoxy and 2-naphthyl derivatives (3c and 3f) emitted fluorescence at 729 nm (13717 cm-1, Φ = 0.02) and 714 nm (14005 cm-1 and Φ = 0.11), respectively, with a large Stokes shift (184 and 182 nm for 3c; 4631 cm-1 and 4791 cm-1 for 3f).INTRODUCTION
A wide variety of fluorescent dyes have been developed for biological applications; for example, Cy3 and Cy5 are used in DNA microarray studies,1 while Alexa fluor dyes2 and FITC dyes are used in immunohistochemistry. Near-infrared fluorescent (NIRF) dyes, in particular, have gained importance for the visualization of abnormal tissues in vivo. NIR fluorescence is unaffected by the autofluorescence arising from red blood cells or organelles, at wavelengths of 650 nm or shorter, and is hence clearly observed in in vivo systems. The NIR wavelength region is called as optical window in biological tissue.3,4
Introduction of aryl groups at the 5-position of the two thiophen rings of the orange fluorescent 4,7-di(thiophen-2-yl)-1,2,5-oxadiazolo[3,4-c]pyridine 1 (Figure 1) causes a long-wavelength shift of the fluorescence by 46–78 nm, because of extension of the π-chromophore in the thiophen rings; the 5-arylthiophen-2-yl derivatives emit fluorescence in the 546–647 nm region.5
The present article discusses the effect of π-chromophore extension on the absorption and emission spectra of ethyl 4,7-bis(5-arylthiophen-2-yl)-1,2,5-oxadiazolo[3,4-c]pyridine-6-carboxylates 3, which were prepared by Suzuki coupling6 of bis(5-bromothiophen-2-yl) derivatives 2 with the corresponding arylboronic acids 4.
RESULTS AND DISCUSSION
1. Preparation of 4,7-bis(5-arylthiophen-2-yl)-1,2,5-oxadiazolo[3,4-c]pyridines 3
The desired π-extended 5-arylthiophen-2-yl derivatives 3a–f were prepared in high yields by the Suzuki coupling of bromothienyl compound 25 with arylboronic acids 4a–f (Scheme 1).
The yield of 4,7-diphenyl derivative 3a was improved (from 67%5 to 91%) when the reaction was performed using a smaller amount of aq. 2 M sodium carbonate and a greater amount of benzene, with effective stirring of the reaction mixture. This procedure was successfully applied for the preparation of substituted-phenyl derivatives 3b–d (82–94% yields) and naphthyl derivatives 3e–f (83–90% yields).
2. Absorption and fluorescence spectra
The spectral properties of 3 were studied in CHCl3 and DMSO (Tables 1, 2 and Figures 2, 3). The absorption spectra of the derivatives with electron-donating methyl and methoxy groups 3b–d and the derivative with a π-extended 2-naphthyl compound 3f showed long-wavelength shift (10–41 nm) as compared with the spectrum of the phenyl derivative 3a.
Compounds 3b–d emitted fluorescence in the 688–729 nm region. The methoxy group was effective in increasing the emission wavelength, but the quantum yield in this case was low. 4-Methoxy derivative 3c emitted fluorescence at 712 nm (14045 cm-1, Φ = 0.13) in CHCl3 and at 729 nm (13717 cm-1, Φ = 0.02) in DMSO. Dimethoxy derivative 3d was weakly fluorescent (723 nm, 13831 cm-1 and Φ = 0.08) in CHCl3 and non-fluorescent in DMSO. Naphthyl compounds 3e–f emitted fluorescence in the 676–714 nm regions, in CHCl3 and DMSO. The emission properties of 2-naphthyl derivative 3f were favorable. Due to steric reasons, it showed longer fluorescence emission spectra and larger quantum yield than 1-naphthyl isomer (3e). 2-Naphthyl derivative 3f emitted fluorescence at 692 nm (14450 cm-1 and Φ = 0.28) in CHCl3 and at 714 nm (14005 cm-1 and Φ = 0.11) in DMSO, while 1-isomer 3e emitted fluorescence at 676 nm (14792 cm-1, Φ = 0.29) in CHCl3 and showed weak fluorescence at 709 nm (14104 cm-1, Φ = 0.05) in DMSO. Compounds 3 had characteristic large Stokes shifts (Tables 1 and 2),5 indicative of a donor-acceptor-donor (D-A-D) structure.7, 8 The solvent effect, which was more significant for emission than for absorption,7, 8 suggested that the structure of 3 in the excited state was more polar than that in the ground state.7-12 The solvent-chromophore interaction of D-A-D compounds 3 may be favored in the polar DMSO than in, CHCl3, thus leading to reduced quantum yields in DMSO.
CONCLUSION
Suzuki coupling of bromothienyl compound 2 with various arylboronic acids 4a–f gave π-extended 5-arylthiophen-2-yl derivatives 3a–f in excellent yields (82–94%). In CHCl3 and DMSO, compounds 3a–f showed NIR fluorescence (673–729 nm) and large Stokes shifts. These properties of compounds 3 will be advantageous for application as fluorescent labeling reagents.
EXPERIMENTAL
General
Melting points were determined on a Yanaco micromelting point apparatus (MP 500P) and are reported as uncorrected values. 1H NMR spectra were obtained on a JEOL JMS-70 (400 MHz) in CDCl3 solution. MS spectra were obtained on a JEOL JMS-70 mass spectrometer. Elemental analyses were performed at the Elemental Analytical Center, Kyushu University. UV-VIS spectra were obtained on a JASCO V560-DS spectrometer. Fluorescence spectra were recorded on a HITACHI F-4500 spectrometer. Fluorescence quantum yields were measured on a HAMAMATSU C10027-02 photoluminescence spectrometer. Column chromatography was carried out on a silica gel column (KANTO C-60).
Typical procedure for the preparation of 3
To a mixture of bis(5-bromothiophen-2-yl) derivative 2 (200 mg, 0.388 mmol) in benzene (8 mL) and phenylboronic acid 4a (118 mg, 0.971 mmol) in EtOH (5 mL) were added aq. 2 M sodium carbonate (2 mL) and tetrakis(tripenylphosphine)palladium(0) (13.5 mg, 11.7 μmol). Then the mixture was heated under reflux for 2 h, cooled to rt, poured into water, extracted with CHCl3, dried over magnesium sulfate, and evaporated in vacuo. The residue was column chromatographed (KANTO C-60, CHCl3/hexane = 1:1, (v/v)) to give crude 3a, which, on recrystallization from MeCN, afforded ethyl 4,7-bis(5’-phenylthiophen-2-yl)-1,2,5-oxadiazolo[3,4-c]pyridine-6-carboxylate 3a (180 mg, 91% yield) as dark red needles; mp 188–190 ºC (Lit.,5 188–190 ºC).
Ethyl 4,7-bis(5’-p-methylphenylthiophen-2-yl)-1,2,5-oxadiazolo[3,4-c]pyridine-6-carboxylate 3b: Yield 94%, dark green needles (EtOAc), mp 229–231 ºC; 1H-NMR (CDCl3) δ: 1.37 (t, 3H, J = 7.1 Hz), 2.39 (s, 3H), 2.40 (s, 3H), 4.50 (q, 2H, J = 7.1 Hz), 7.23 (dd, 4H, J = 7.8, 6.1 Hz), 7.36 (d, 1H, J = 3.9 Hz), 7.45 (d, 1H, J = 4.0 Hz), 7.56 (d, 2H, J = 8.2 Hz), 7.63 (d, 2H, J = 8.2 Hz), 7.72 (d, 1H, J = 3.9 Hz), 8.51 (d, 1H, J = 4.0 Hz); FAB-MS (3-nitrobenzyl alcohol): m/z 537 (M+); Anal. Calcd for C30H23N3O3S2: C, 67.02; H, 4.31; N, 7.82. Found; C, 67.18; H, 4.22; N, 7.99.
Ethyl 4,7-bis(5’-(4”-methoxyphenyl)thiophen-2-yl)-1,2,5-oxadiazolo[3,4-c]pyridine-6-carboxylate 3c: Yield 94%, black needles (EtOAc, mp 226–228 ºC; 1H-NMR (CDCl3) δ: 1.38 (t, 3H, J = 7.1 Hz), 3.86 (s, 3H), 3.87 (s, 3H), 4.50 (q, 2H, J = 7.1 Hz), 6.96 (dd, 4H, J = 8.8, 4.7 Hz), 7.29 (d, 1H, J = 3.9 Hz), 7.39 (d, 1H, J = 4.0 Hz), 7.60 (d, 2H, J = 8.8 Hz), 7.68 (d, 2H, J = 8.9 Hz), 7.71 (d, 1H, J = 3.9 Hz), 8.50 (d, 1H, J = 4.0 Hz); FAB-MS (3-nitrobenzyl alcohol): m/z 569 (M+); Anal. Calcd for C30H23N3O5S2: C, 63.25; H, 4.07; N, 7.38. Found; C, 63.01; H, 4.27; N, 7.55.
Ethyl 4,7-bis(5’-(3”,4”-dimethoxyphenyl)thiophen-2-yl)-1,2,5-oxadiazolo[3,4-c]pyridine-6- carboxylate 3d: Yield 82%, dark violet needles (EtOAc), mp 186–188 ºC; 1H-NMR (CDCl3) δ: 1.38 (t, 3H, J = 7.1 Hz), 3.93 (s, 3H), 3.94 (s, 3H), 3.97 (s, 3H), 3.99 (s, 3H), 4.50 (q, 2H, J = 7.2 Hz), 6.91 (d, 1H, J = 4.3 Hz ), 6.93 (d, 1H, J = 4.2 Hz), 7.15 (s, 1H), 7.21 (s, 1H), 7.24 (d, 1H, J = 8.3 Hz), 7.30 (d, 1H, J = 3.9 Hz), 7.33 (d, 1H, J = 8.4 Hz), 7.40 (d, 1H, J = 4.0 Hz), 7.70 (d, 1H, J = 3.9 Hz), 8.50 (d, 1H, J = 4.0 Hz); FAB-MS (3-nitrobenzyl alcohol): m/z 629 (M+); Anal. Calcd for C32H27N3O7S2: C, 61.04; H, 4.32; N, 6.67. Found; C, 60.76; H, 4.56; N, 6.88.
Ethyl 4,7-bis(5’-(1”-naphthyl)thiophen-2-yl)-1,2,5-oxadiazolo[3,4-c]pyridine-6-carboxylate 3e: Yield 90%, Red needles (MeCN), mp 204–207 ºC; 1H-NMR (CDCl3) δ: 1.39 (t, 3H, J = 7.1 Hz), 4.50 (q, 2H, J = 7.2 Hz), 7.38 (d, 2H, J = 3.8 Hz), 7.46 (d, 2H, J = 3.9 Hz), 7.52–7.58 (m, 6H), 7.65–7.71 (m, 2H), 7.89 (d, 1H, J = 3.8 Hz), 7.91–7.96 (m, 4H), 8.29–8.33 (m, 2H), 8.65 (d, 1H, J = 3.9 Hz); FAB-MS (3-nitrobenzyl alcohol): m/z 609 (M+); Anal. Calcd for C36H23N3O3S2: C, 70.91; H, 3.80; N, 6.89. Found; C, 70.93; H, 3.81; N, 6.89.
Ethyl 4,7-bis(5’-(2”-naphthyl)thiophen-2-yl)-1,2,5-oxadiazolo[3,4-c]pyridine-6-carboxylate 3f: Yield 83%, dark green needles (EtOAc), mp 232–235 ºC; 1H-NMR (CDCl3) δ: 1.41 (t, 3H, J = 7.1 Hz), 4.54 (q, 2H, J = 7.2 Hz), 7.50–7.55 (m, 5H), 7.64 (d, 2H, J = 4.2 Hz), 7.78–7.92 (m, 9H), 8.14 (s, 1H), 8.23 (s, 1H), 8.57 (d, 1H, J = 4.0 Hz); FAB-MS (3-nitrobenzyl alcohol): m/z 609 (M+); Anal. Calcd for C36H23N3O3S2: C, 70.91; H, 3.80; N, 6.89. Found; C, 70.88; H, 3.83; N, 6.84.
References
1. J. L. DeRisi, V. R. Iyer, and P. O. Brown, Science, 1997, 278, 680. CrossRef
2. R. Haugland, ‘The Molecular Probes® Handbook, A Guide to Fluorescent Probes and Labeling Technologies’, 10th, ed. by life technologies, Inc., California, 2005.
3. H. Kojima, Yakugaku Zasshi, 2008, 128, 1653. CrossRef
4. R. Weissleder, Nat. Biotech., 2001, 19, 316. CrossRef
5. H. Gorohmaru, T. Thiemann, T. Sawada, K. Takahashi, K. Nishi-i, N. Ochi, Y. Kosugi, and S. Mataka, Heterocycles, 2002, 56, 421. CrossRef
6. N. Miyaura and A. Suzuki, Chem. Rev., 1995, 95, 2457. CrossRef
7. H. Hiyoshi, H. Kumagai, H. Ooi, T. Sonoda, and S. Mataka, Heterocycles, 2007, 72, 231. CrossRef
8. R. Nandy and S. Sankararaman, Beilstein J. Org. Chem., 2010, 6, 992. CrossRef
9. J. A. Marsden, J. J. Miller, L. D. Shirtcliff, and M. M. Haley, J. Am. Chem. Soc., 2005, 127, 2464. CrossRef
10. A. B. J. Parusel, K. Rechthaler, D. Piorun, A. Danel, K. Khatchatryan, K. Rotkiewicz, and G. Kohler1, J. Fluoresc., 1998, 8, 375. CrossRef
11. R. Letrun, M. Koch, M. L. Dekhtyar, V. V. Kurdyukov, A. I. Tolmachev, W Rettig, and E. Vauthey, J. Phys. Chem. A, 2013, 117, 13112. CrossRef
12. C. Cao, X. Liu, Q. Qiao, M. Zhao, W. Yin, D. Mao, H. Zhang, and Z. Xu, Chem. Comm., 2014, 50, 15811. CrossRef