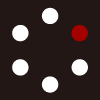
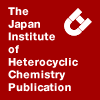
HETEROCYCLES
An International Journal for Reviews and Communications in Heterocyclic ChemistryWeb Edition ISSN: 1881-0942
Published online by The Japan Institute of Heterocyclic Chemistry
e-Journal
Full Text HTML
Received, 19th October, 2015, Accepted, 6th January, 2016, Published online, 18th January, 2016.
■ Preparation of Nicotinoyl Amino Acid Derivatives by Fmoc-Solid Phase Synthesis and Preliminary Studies on the DNA-Binding Properties of Nicotinoyl Derivatives
Dongxin Zhao* and Kui Lu*
School of Chemistry and Chemical Engineering, Henan University of Technology, Zhengzhou 450001, China
Abstract
Three types of nicotinoyl amino acids, i.e., nicotinoyl leucine (NA-Leu), NA-Leu-His, and NA-Tyr-Tyr, were synthesized by Fmoc solid-phase peptide synthesis, purified by reversed-phase HPLC, and characterized by 1H, 13C NMR and ESI-MS. The interactions of nicotinic acid and nicotinoyl derivatives with ctDNA were investigated by fluorescence spectroscopy. NA-Leu-His and NA-Tyr-Tyr exhibited higher affinity for ctDNA compared with free NA, indicating that the imidazolyl of histidine and the phenol group of tyrosine can enhance the embedding interaction of nicotinoyl derivatives into ctDNA. In addition, leucine in the derivatives helped form a special surrounding and spatial structure to interact with ctDNA. The stronger interaction of NA-Tyr-Tyr and NA-Leu-His with ctDNA suggested that the modified nicotinic acid probably erhaps had significant practical value and should be further studied.introduction
Nicotinic acid (NA) is one of thirteen necessary vitamins, and also is known as vitamin PP (pellegra preventive factor) because it has been identified as the cause of pellegra disease. Niacinamide cannot be directly converted from NA in the body, but both compounds are important components of the co-factors NAD and NADP.1 At present, NA and niacinamide are mainly used as peripheral vasodilators in the treatment of migraine, tinnitus, aural vertigo, cardiovascular diseases, and Alzheimer diseases.2 A myriad of studies examined the efficacy and safety of nicotinic acid and niacinamide. However, several studies have reported the adverse effects of nicotinic acid and niacinamide, such as diarrhea, hyperglycemia and hepatotoxicity.3 Several studies have recently also focused on improving the stability, absorption, and potency of drug by peptidyl modification based on the peptide transporter system of mammalians, which can mediate the absorption of peptidyl drugs with amide bonds.4 Tyrosine and histidine have special functions for phenol and imidazole sidechains in medicines, metalloproteins, and specific enzymes by common coordinating ligands in vivo, aside from being protein frame. Leucine is usually used as a component of proteins to form a specific hydrophobic environment.5 Nicotinoyl derivatives can be modified by amino acids, whose stability, side effect, and degrade can be improved because of amide bond and sidechain of amino acid residues.6 Nicotinic acid derivatives, i.e., nicotinoyl leucine (NA-L-Leu, 1), NA-L-Leu-L-His (2), and NA-L-Tyr-L-Tyr (3) were efficiently synthesized in this study through solid-phase peptide synthesis and characterized by IR, 1H NMR, 13C NMR, and ESI-MS. Preliminary studies on DNA-binding properties of nicotinoyl derivatives through UV absorption spectroscopy and fluorescence emission spectroscopy were described.
RESULTS AND DISCUSSION
Synthesis. Nicotinoyl derivatives were prepared according to a modification of the literature report.7 The synthetic process is shown in detail in Scheme 1. NA peptides were synthesized by solid phase peptide synthesis on Wang resin with good yield, and then analyzed by RP-HPLC thorugh the peak area normalization method, 1 (82.0%), 2 (88.2%), and 3 (88.0%). The final products of nicotinoyl derivatives were isolated in high purity (95%) and characterized by NMR and ESI-MS. Thus, the synthesized derivatives can be used to investigate their properties.
Ultraviolet absorption spectra. The contraction and extension of the DNA molecule usually induced hypochromicity in the ultraviolet absorption spectra of the drug-DNA interaction system, and the explosion of DNA base pair led hyperchromicity. Ultraviolet absorption at 260 nm of ctDNA showed a hypochromic effect with increasing concentration of NA and NA derivatives, but the maximum absorption wavelength was not changed.
According to the equation:8 ΔA-1 = (ΔεCD)-1 + (ΔεKCD)-1CN-1, CN is the analysis concentration of NA and the derivatives, CD is the analysis concentration of ctDNA, Δε is a constant value in a certain measurement condition, and K is the apparent binding constant. In the experiment, fixed CD and changed CN, then ΔA-1 and CN-1 showed a linear relationship, so the K of NA and NA derivatives with DNA obtained from the regression line were 5.81 × 102 mol-1·L-1 (NA), 1.88 × 103 mol-1·L-1 (1), 8.2 × 103 mol-1·L-1 (2), and 7.25 × 102 mol-1·L-1 (3), respectively. This result indicates that the modification of nicotinic acid by amino acids promotes interaction strength between NA derivatives and ctDNA, so the fluorescence spectra study was performed to investigate the interaction mode of NA or NA derivatives with ctDNA.
Fluorescence spectra. Fluorescence quenching is a simple, powerful and effective probe to determine the nature of interactions between organic particles and natural organic macromolecules. Ethidium bromide (EB) is a commonly used fluorescent dye for DNA binding studies because its fluorescence is extremely weak, and can specifically embed in base pairs of DNA double-helical, enhancing the fluorescence of a system. When another molecule intercalates with the stacked base pairs of DNA, it competes with the action site of the DNA base pairs with EB, and EB dissociates from the DNA, which finally leads to fluorescence quenching. According to the Long’s theory, the fluorescence quenching extent of EB-DNA can be used to confirm the binding extent between the molecule and DNA based on the fluorescence quenching of the EB-DNA system at 608 nm by increasing the niacin and NA peptide concentrations.9 Thus, the intercalative mechanism of NA and NA peptides was further confirmed by fluorescence quenching after interaction with ctDNA.
Figure 1 shows that the Stern-Volmer plots of 1, 2 and 3 are linear, whereas that of niacin is non-linear. The linear process should follow the Stern-Volmer equation:10 F0/F = 1 + Kq0[Q], where F0 and F are the fluorescence intensities of the system in the absence and presence of quenching agents, [Q] is the concentration of the quencher, Kq is the quenching constant, and 0 is the average life of fluorescent molecules without a quenching agent at approximately 10-8 s.11 The quenching constants (Kq) of derivatives with the DNA-EB system were 7.89×109 L·mol-1·s-1 (1), 2.44×1011 L·mol-1·s-1 (2), and 3.13×1010 L·mol-1·s-1 (2) based on the Stern-Volmer equation.
Fluorescence quenching is divided into dynamic quenching and static quenching. Static quenching is caused by the non-fluorescent compound produced by the interaction between the quenching agent and the fluorescent substance in the ground state. Dynamic quenching refers to fluorescent substance molecules in the excited state colliding with the quenching agent molecules, thereby resulting in fluorescence intensity quenching. The Stern-Volmer plots of NA is non-linear, indicating a complex quenching behavior between NA with ctDNA. This quenching most likely involves both static and dynamic quenching mechanisms because of the embedding interaction from the pyridyl of NA and the electrostatic interaction from the carboxyl of NA.12 The Kq of 1 is smaller than the maximum diffusion controlled collision rate constant (2×1010 L·mol-1·s-1) of small molecules with biological macromolecules, indicating that the fluorescence quenching action of 1 on the DNA-EB system is dynamic quenching caused by collisions because static quenching is weakened through the hydrophobic force of leucine residue. The Kq of 3 is slightly higher than the maximum diffusion controlled collision rate constant (2×1010 L·mol-1·s-1) of small molecules with biological macromolecules, indicating that the fluorescence quenching actions of 3 on DNA-EB system are not dynamic quenching but static quenching because the phenol groups of tyrosine in 3 can embed into the groove structure of ctDNA. Meanwhile, the Kq of 2 is higher than the Kq of 3, indicating that the spatial structure of 2 is more suitable for embedding into the DNA groove for the leucine between nicotinic acid and histidine, and the imidazolyl of histidine and the pyridyl of nicotinic acid can interact with the ctDNA base pair by π-π stacking interaction.13
Thus, this scenario indicates that nicotinic acid modified with tyrosine and histidine is easier to interact with DNA by embedding into the DNA groove, but still need an appropriate spatial structure to enhance the interaction strength. The interaction of 3 and 2 with DNA is stronger than free nicotinic acid, which suggests tyrosine and histidine modified nicotinic acid probably had significant practical value. The results provide valuable information in understanding the interaction mode of modified small molecules with DNA and in exploring the development of novel and highly effective peptidyl drugs.
EXPERIMENTAL
The NA derivatives were analyzed and purified on an Agilent C18 column (150 mm × 4.6 mm) with 5 μm silica as a stationary phase. A gradient elution with eluent A (0.5% TFA) and eluent B (acetonitrile) was used at a flow rate of 1.0 mL·min-1. Peaks were detected at λ = 246 nm. 1H NMR and 13C NMR experiments were performed using a Bruker DPX-400 MHz instrument with TMS as the internal standard. Mass spectra were analyzed on an Agilent Esquire 3000 mass spectrometer fitted with an ion spray source working in positive ion mode with methanol as the solvent.
Starting Materials. All chemicals used in this study were commercially available.
Synthesis. The synthetic process of 2 is detailed as follows. Fmoc-L-His(Trt)-Wang Resin (571.8 mg, 0.51 mmol/g) was placed in a peptide synthesis vessel and treated with DMF (5 mL, 30 min), 20% piperidine in DMF (5 mL, 3 min), 20% piperidine in DMF (5 mL, 30 min), DMF (5×3 mL, 2 min each), and MeOH (5×3 mL, 2 min each). The Kaiser test was performed at this point to identify the presence of free amine available for coupling. A coupling solution was prepared by adding DIEA (0.135 mL, 0.8169 mmol) to a DMF (5 mL) solution containing Fmoc-L-Leu-OH (571.5 mg, 0.2172 mmol), HOBT (108.5 mg, 0.8000 mmol) and HBTU (303.8 mg, 0.8000 mmol) in an ice bath. The coupling solution was added to the peptide synthesis vessel containing the resin and reacted for 4 h. The resin was washed with DMF (5 × 3 mL, 2 min each) and MeOH (5 × 3 mL, 2 min each). A coupling solution was prepared by adding DIEA (0.135 mL, 0.8169 mmol) to a DMF (5 mL) solution containing nicotinic acid (98.5 mg, 0.8000 mmol), HOBT (108.3 mg, 0.8000 mmol) and HBTU (303.4 mg, 0.8000 mmol) in an ice bath after the Kaiser test showed the full coupling. This solution was added to the peptide synthesis vessel and reacted for 4 h. After the Kaiser test showed the full coupling, the resin was then washed with DMF (2 × 10 ml, 2 min each), CH2Cl2 (2 × 5 mL, 2 min each), MeOH (2 × 5 mL, 2 min each), and DMF (2 × 10 mL, 2 min each). The cleavage of NA-L-Leu-L-His from the resin was accomplished using a solution of 95% TFA, 2.5% TIS, and 2.5% water for 3 h. The crude product of NA-L-Leu-L-His was obtained by precipitation in cold diethyl ether.
NA-L-Leu-L-His (2). IR (cm-1, KBr Disc): 3360-2800 (O-H), 1680 (C=O), 1520 (N-H), 1460(C-H), 1200 (C-N), and 840-720 (C-H). 1H NMR (D2O): δ 9.09 (s, 1H, pyridyl-H), 8.85 (d, J = 2.05 Hz, 2H, pyridyl-H), 8.53 (t, J = 0.87 Hz, 1H, pyridyl-H), 8.11 (d, J = 1.00 Hz, 1H, imidazole-H), 7.23 (s, 1H, imidazole-H), 4.42 (t, J = 1.04 Hz, 1H, HNCHCONH), 3.29 (t, J = 1.06 Hz, 1H, CHCH2-imidazolyl), 3.13 (d, J = 1.14 Hz, 2H, CHCH2-imidazolyl), 1.66-1.48 (m, 3H, CHCH2CH(CH3)2), and 0.82 (d, J = 6.14 Hz, 6H, CHCH3). 13C NMR (D2O): δ 24.28, 51.46, 53.49 (CH); 26.00, 39.57 (CH2); 20.80, 21.81 (CH3); 164.28, 173.01, 174.17 (C=O); 127.60, 128.67, 141.81, 143.64, 145.18 (pyridyl); 117.23, 117.78, 133.30 (imidazolyl). Mass spectrometry calcd for C18H23N5O4: [M+H]+ = 373.4. Found: 373.8.
NA-L-Leu (1).14 1H NMR (D2O): δ 1.601-1.730 (m, 2H, -CH2-), 1.31-1.54 (m, 2H, -CH-), and 0.74-0.85 (m, 3H, -CH3). Mass spectrometry calcd for C12H16N2O3: [M+H]+ = 236.3. Found: 237.1.
NA-L-Tyr-L-Tyr (3). 1H NMR (D2O): δ 7.07 (d, J = 2.12 Hz, 4H, Ar-H), 6.75 (d, J = 4.35 Hz, 4H, Ar-H), 4.58-4.66 (m, 2H, HNCHCO), 2.67-2.74 (m, 2H, CHCH2-Ar). Mass spectrometry calcd for C24H23N3O6: [M+H]+ = 449.4. Found: 449.9.
UV-vis absorption spectroscopy measurements. UV-vis absorption spectra were recorded in a quartz cell on a UV-2450 UV-vis spectrometer. The concentration of ctDNA solution was calculated based on the standard ε260 = 6600 L·mol-1·cm-1.15 3 mL of 3.75 × 10-3 mol·L-1 ctDNA solution was then added to colorimetric ware and titrated with a specific volumes of NA and NA derivatives. The volumes of NA and NA derivatives solutions (3.75 × 10-2 mol·L-1) were determined by Rt (Rt = cmodification/cDNA). The background absorption from all reagents was subtracted from the absorption spectra by using a corresponding solution without ctDNA as the reference solution.
Fluorescence emission spectroscopy measurements. Steady-state fluorescence spectra were recorded in a quartz cell (light path 10 mm) on an F 23010 fluorescence spectrometer. Different volumes of NA and niacin derivatives solutions determined by Rt (Rt = cmodification/cDNA) added to a series of 10 mL colorimetric tube at fixed concentration of ctDNA (4.1 × 10-5 mol·L-1) and EB (1.23 × 10-6 mol·L-1), respectively, that was diluted to 10 mL with a Tris-HCl buffer solution (pH = 7.4). After heating in a water bath for 1 h at a constant temperature (37 ºC), its fluorescence spectra were measured in the range of 550 nm to 700 nm at an excitation wavelength of 260 nm by using a spectrofluorometer (Varian, America). The entrance and exit slits for all fluorescence measurements were both maintained at 5 nm.
ACKNOWLEDGEMENTS
This work supported by the National Natural Science Foundation of China (21172054 and 21301050), the Innovation scientists and Technicians Troop construction projects of Zhengzhou city (10LJRC174) and the Foundation of Education Department of Henan Province (13B150947).
References
1. J. Hegyi, R. A. Schwartz, and V. Hegyi, Int. J. Dermatol., 2004, 43, 1; CrossRef M. C. Chuong, L. Palugan, T. M. Su, C. Busano, R. Lee, G. D. Pretoro, and A. Shah, AAPS PharmSciTech., 2010, 11, 1650; CrossRef P. Belenky, K. L. Bogan, and C. Brenner, Trends Biochem. Sci., 2007, 32, 12. CrossRef
2. T. Rubic, M. Trottmann, and R. L. Lorenz, Biochem. Pharmacol., 2004, 67, 411; CrossRef E. Holzhӓuser, C. Albrecht, Q. Zhou, A. Buttler, M. R. Preusch, E. Blessing, H. A. Katyss, and F. Bea, J. Cardiovasc. Pharm., 2011, 57, 447; K. N. Green, J. S. Steffan, H. Martinez-Coria, X. M. Sun, S. S. Schreiber, L. M. Thompson, and F. M. Laferla, J. Neurosci., 2008, 28, 11500. CrossRef
3. M. Knip, I. F. Douek, W. P. T. Moore, H. A. Gillmor, A. E. M. Mclean, P. J. Bingley, and E. A. M. Gale, Diabetologia, 2000, 43, 1337; CrossRef D. M. Capuzzi, J. M. Morgan, O. A. Brusco, and C. M. Intenzo, Curr. Atheroscler. Rep., 2000, 2, 64; CrossRef D. Papaliodis, W. Boucher, D. Kempuraj, M. Michaelian, A. Wolfberg, M. House, and T. C. Theoharides, J. Pharmacol. Exp. Ther., 2008, 327, 665; CrossRef M. K. Mittal, T. Florin, J. Perrone, J. H. Delgado, and K. C. Osterhoudt, Ann. Emerg. Med., 2007, 50, 587; CrossRef A. Pandit, T. Sachdeva, and P. Pafna, J. Appl. Pharm. Sci., 2012, 2, 233.
4. C. Pestourie, B. Thézé, B. Kuhnast, S. L. Helleix, K. Gombert, F. Dollé, B. Tavitian, and F. Duconqé, Eur. J. Nucl. Med. Mol. Imaging, 2010, 37, 58; CrossRef W. J. Liu and X. Lu, Asian J. Pharm. Sci., 2012, 7, 287.
5. D. Webster and J. Wildgoose, Cochrane Db. Syst. Rev., 2013, 6, 1; X. Z. Chen, A. Steel, and M. A. Hediger, Biochem. Bioph. Res. Co., 2000, 272, 726. CrossRef
6. A. Ezra, A. Hoffman, E. Breuer, I. S. Alferiev, J. Mӧnkkӧnen, N. E. Hanany-Rozen, G. Weiss, D. Stepensky, I. Gati, H. Cohen, S. Tӧrmӓlehto, G. L. Amidon, and G. Golomb, J. Med. Chem., 2000, 43, 3641; CrossRef C. Yang, G. S. Tirucheraigs, and A. K. Mitra, Expert Opin. Biol. Ther., 2001, 1, 159. CrossRef
7. M. J. Sever and J. J. Wilker, Tetrahedron, 2001, 57, 6139; CrossRef K. Lu, J. Sun, D. X. Zhao, Q. C. Zhu, and W. Lv, Int. J. Pept. Res. Ther., 2014, 20, 299; CrossRef D. X. Zhao, L. Ma, K. Lu, J. Z. Wu, and J. He, Res. Chem. Intermediat., 2015. DOI: 10.1007/s11164-014-1913-1. CrossRef
8. S. A. Tysoe, A. D. Barker, and T. C. Strekas, J. Phys. Chem., 1993, 97, 1707; CrossRef C. Y. Zhou, Y. Zhao, Y. B. Wu, C. X. Yin, and Y. Pin, J. Inorg. Biochem., 2007, 101, 10; CrossRef Z. Q. Liu, M. Jiang, Y. T. Li, Z. Y. Wu, and J. X. Yang, Inorg. Chim. Acta, 2009, 362, 1253; CrossRef W. J. Mei, Chem. J. Chinese Univ., 2002, 23, 2049.
9. C. Y. Zhou, Y. Zhao, Y. B. Wu, C. X. Yin, and Y. Pin, J. Inorg. Biochem., 2007, 101, 10; CrossRef Q. Guo, L. Z. Li, J. F. Dong, H. Y. Liu, Z. C. Xue, and T. Xu, Acta Chim. Sinica, 2012, 70, 1617; CrossRef E. J. Gao, M. C. Zhu, H. X. Yin, L. Liu, Q. Wu, and G. Sun, J. Inorg. Biochem., 2008, 102, 1958. CrossRef
10. H. L. Geng, L. Ma, K. Lu, Y. J. Ma, and X. Wu, Chin. J. Anal. Chem., 2009, 37, 548; A. Manciulea, A. Baker, and J. R. Lead, Chemosphere, 2009, 76, 1023. CrossRef
11. S. G. Geng, Y. R. Cui, Q. F. Liu, F. L. Cui, G. S. Zhang, Y. W. Chi, and H. Peng, J. Lumin., 2013, 141, 144; CrossRef J. Sartorius and H. J. Schneider, J. Chem. Soc., Perkin. Trans. 2, 1997, 2319. CrossRef
12. S. G. Geng, Q. Wu, L. Shi, and F. L. Cui, Int. J. Biol. Macromol., 2013, 60, 288; CrossRef S. Scheiner and M. M. Szczesniak, Prog. Clin. Biol. Res., 1985, 172, 53; J. Zhao and D. Nelson, J. Inorg. Biochem., 2005, 99, 383. CrossRef
13. A. Airinei, R. I. Tigoianu, E. Rusu, and D. O. Dorohoid, Dig. J. Nanomater. Bios., 2011, 6, 1265; H. L. Cheng, L. Ma, and K. Lu, Chinese J. Anal. Chem., 2009, 37, 548; CrossRef N. Shahabadin and S. Mohammadi, Bioinorg. Chem. Appl., 2012, 26, 571913; F. Zhao, H. Liang, H. Cheng, J. Wang, and W. H. Zhao, Chem. J. Chinese Univ., 2011, 32, 1277.
14. K. Kurita, T. Nishibori, and M. Harata, Biomacromolecules, 2002, 3, 705. CrossRef
15. E. J. Gao, M. C. Zhu, H. X. Yin, L. Liu, Q. Wu, and Y. G. Sun, J. Inorg. Biochem., 2008, 102, 1958 CrossRef