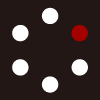
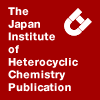
HETEROCYCLES
An International Journal for Reviews and Communications in Heterocyclic ChemistryWeb Edition ISSN: 1881-0942
Published online by The Japan Institute of Heterocyclic Chemistry
e-Journal
Full Text HTML
Received, 25th December, 2015, Accepted, 22nd February, 2016, Published online, 26th February, 2016.
DOI: 10.3987/REV-15-836
■ Syntheses of Heterocycles via Alkyne-Carbonyl Metathesis of Unactivated Alkynes
Akio Saito* and Keiichiro Tateishi
Division of Applied Chemistry, Graduate School of Engineering, Tokyo University of Agriculture and Technology, 2-24-16 Naka-cho, Koganei, Tokyo 184-8588, Japan
Abstract
The formation of α,β-unsaturated carbonyl compounds by a catalytic alkyne-carbonyl metathesis, which can be catalyzed by both π- and σ-acids, has received attention as an atom economical process alternative to the Wittig reaction. During the last decade, the catalytic alkyne-carbonyl metathesis has provided attractive methods for the construction of various cyclic compounds. This review summarizes synthetic methods of heterocycles with a focus on recent advances in the catalytic alkyne-carbonyl metathesis of the unactivated alkynes.CONTENTS
1. Introduction
2. Syntheses of heterocycles via intramolecular alkyne-carbonyl metathesis
2.1. Ring-closing metathesis and the related reaction of alkynals or alkynones
2.2. Ring-closing metathesis of alkynyl acetals
2.3. Domino reactions based on intramolecular alkyne-carbonyl metathesis
3. Syntheses of heterocycles via intermolecular alkyne-carbonyl metathesis
4. Conclusion
1. introduction
A metathesis reaction between a carbon–carbon triple bond and a carbonyl group, which is called “alkyne-carbonyl metathesis”, “hetero-enyne metathesis”, and so on, provides an attractive method for the construction of α,β-unsaturated carbonyl compounds (Scheme 1).1 The alkyne-carbonyl metathesis was discovered from a study on UV-irradiated [2+2] cycloaddition reactions of unactivated alkynes with aromatic aldehydes or ketones in 1956.2 Although the photoirradiation promoted the intramolecular alkyne-carbonyl metathesis of alkynes with aromatic aldehydes, ketones and quinones,3 the photoreaction brought about low yields, low regioselectivities and/or low stereoselectivities of products.2,3 In 1959, Arens et al. first reported the Lewis acid-mediated formation of α,β-unsaturated esters from alkynyl ethers with aldehydes or ketones (Scheme 1a).4 Since then, Lewis acid such as BF3•OEt2 and TiCl4 have been reported to be effective on the alkyne-carbonyl metathesis of heteroatom-substituted alkynes with various carbonyl compounds including acetals and esters.5,6 These reactions have been considered to proceed via oxetene intermediates because oxetenes were isolated in some cases.7 On the basis of ab initio studies on alkyne-carbonyl metathesis of methoxyacetylene and formaldehyde, Pons et al. proposed that Lewis acid promotes asynchronous formation of the oxetene intermediates and further electrocyclic ring-opening into methyl acrylate (Scheme 1b).8
Lithium ynolates can react with various carbonyl compounds in the absence of Lewis acid to afford β-lactones (path a)9 or α,β-unsaturated carboxylic acids (path b),10 which are formed from common oxetene intermediates (Scheme 2). In particular, the formation of α,β-unsaturated carboxylic acids from aldehydes,10 acylsilanes,10c α-oxy- and α-amino ketones,10e alkynyl ketones,10g and esters10f,h proceeded with excellent stereoselectivity. Shindo et al. explicated that this high torquoselectivity11 would be attributed to orbital interactions in the electrocyclic ring-opening reactions of oxetene intermediates.10 Thus, the strong orbital interaction between the nonbonding orbital of the oxygen on the oxetene as well as the cleaving C-O σ-bond with antibonding orbitals such as the Si-C σ*-orbitals, C-Z (Z = O, N) σ*-orbitals makes the electron accepting substituents A at C-4 position of oxetene rotate inward. On the other hand, electron donating substituents D rotates outward. When such electronic properties of the substituents are not significantly different, steric effects of C-3 and C-4 substituents tend to influence on the torquoselectivity of the ring-opening reactions.
Lewis acids can also promote the intermolecular alkyne-carbonyl metathesis of unactivated alkynes and aldehydes (Scheme 1). Although BF3•OEt2-mediated reactions of aromatic alkynes were exemplified in the early reports of alkyne-carbonyl metathesis,12 Yamaguchi et al. demonstrated a stoichiometric amount of SbF5 could be applied not only to aromatic alkynes but also to less reactive aliphatic alkynes (Scheme 3).13 The regioselectivity of the formation of oxetene intermediates tend to depend on steric effects of substituents of unactivated alkynes (RL > RS), the case of unsymmetrical aliphatic alkyne bearing no significantly different substituents (RL = nBu, RS = Me) even proceeds with high regioselectivity.
As early studies on the intramolecular alkyne-carbonyl metathesis, Hanack, Weiler and others reported that alkynyl ketones underwent both Brønsted and Lewis acid-promoted formation of cyclic enones (Scheme 4a, b).14 Notably, oxygen-18 labeling experiments by Harding et al. showed the complete incorporation of carbonyl oxygen of these starting materials into the final products.14e Although previous mechanism for the formation of endo-cyclic enones have been considered to involve a highly strained intermediate such as an anti-Bredt oxetene (Scheme 4a, b), Wempe and Grunwell suggested new mechanism via a tricyclic oxetane intermediate (Scheme 4c) on the basis of NMR studies and ab initio calculations.15
In the last decade, the catalytic alkyne-carbonyl metathesis, which provides an atom-economical manner alternative to the Wittig reaction, of unactivated alkynes has been achieved by the use of Lewis acid such as Yb(OTf)3,16 BF3•OEt2, AgSbF6,17 AuCl3-AgSbF6,18 Fe(III) halides,19 SbF5-alcohol20 and others,21 or Brønsted acid.17,22,23 For example, Krische et al. reported the “intermolecular” reaction of aromatic alkynes and aldehydes under Ag(I), BF3 or HBF4 catalysis, in which the activation of C-C triple bonds by AgSbF6 was confirmed by 13C-NMR analysis (Scheme 5a).17 In contrast to AgSbF6, BF3,8 SbF5-alcohol20 or In(OTf)3-alcohol21b catalytic system activates carbonyl group of aldehydes in the reaction of aromatic alkyne and aldehydes. In other words, both π- and σ-acids can catalyze the alkyne-carbonyl metathesis reactions.24
Jin and Yamamoto have succeeded in developing the “intramolecular” reaction of carbon-tethered 6-alkynyl ketones catalyzed by Au(III)18 and that of 5-alkynyl ketones catalyzed by TfOH (Scheme 6a).22a Notably, TfOH catalyst worked well in MeOH solvent, which would be attributed to the formation of reactive oxonium intermediates for alkyne-carbonyl metathesis.22a In connection with these reactions, a similar formation of carbocyclic enones from 5-, 6-, and 7-alkynynals in trifluoroacetic acid (TFA) media has been reported by Saá group (Scheme 6b).25 Interestingly, terminal 5-alkynynals were converted to 6-membered ring products, whereas internal ones were converted to 5-membered ring products. The difference in these cyclization modes would indicate aldol mechanism via the regioselective formation of vinyl trifluoroacetate intermediates as proposed by the authors.
Although these intramolecular reactions have been shown as the synthetic method of carbocylic compounds, the catalytic alkyne-carbonyl metathesis can be applied to heterocyclic syntheses like the reactions of heteroatom-tethered 1,5- and 1,6-ynals by Krische’s methods (Scheme 5b).17 Since these reports, there has been a significant increase in research efforts directed towards the development of novel methods for the construction of heterocycles based on the alkyne-carbonyl metathesis. In this review, we describe an overview of the field, concentrating on recent advances in the catalytic alkyne-carbonyl metathesis of the unactivated alkynes.
2. Syntheses of heterocycles via intramolecular alkyne-carbonyl metathesis
2.1. Ring-closing metathesis and the related reaction of alkynals or alkynones
A ring-closing alkyne-carbonyl metathesis of acyclic heteroatom-tethered precursors provides powerful tools for constructing medium ring-size heterocyclic compounds. Among them, easily available O-alkynyl salicylaldehyde derivatives have been relatively well studied as the precursors. As stated above, the ring-closing alkyne-carbonyl metathesis of heteroatom-tethered 1,5- and 1,6-ynals, which is a first reported case of the heterocyclic synthesis by the catalytic alkyne-carbonyl metathesis, has been reported by Krische et al. in 2005. In this literature, the authors demonstrated AgSbF6 efficiently catalyzed the reactions of the O-propargyl salicylaldehydes 1a, b compared to BF3 or HBF4 (Scheme 7).17 Later on, Hsung et al. have been found out a catalytic ring-closing ynamide-carbonyl metathesis of O-alkynyl salicylaldehydes (Scheme 8).26 Interestingly, the ring-closing metathesis of 1d, which would be more reactive than the corresponding unactivated alkynes, occurred concomitantly during the amidation of 1c.
After these reports, Jana et al. reported the environmentally friendly and inexpensive FeCl3 catalyst was effective on the formation of various functionalized 2H-chromenes 2 (Scheme 9, upper).27a Furthermore, FeCl3 catalyst can be employed for the synthesis of oxepines 4 from the homopropargyl derivatives 3 and for the synthesis of nitrogen-containing heterocycles 6 from the tosylamide-tethered 5 (Scheme 10). 27b,c As well as the reaction of methoxyacetylene (Scheme 1),8 these reactions were suggested to procced through stepwise [2+2] cycloaddition reactions (Scheme 9, upper).27a Thus, it is crucial to generate vinylic carbocation intermediate A efficiently and therefore aryl-substituted alkynes (R = aryl) brought about superior results to alkyl-substituted ones (R = alkyl). Similar observations were shown in Krische’s report, in which the reaction of aromatic alkyne 1a proceeded at lower temperature than that of aliphatic alkynes 1b (Scheme 7). Unfortunately, in cases of terminal alkynes (R or R2 = H), FeCl3 do not catalyze these reactions along with the recovery of starting materials (Scheme 9 and 10).27 Very recently, Kim et al. applied the ring-closing alkyne-carbonyl metathesis of 1a [X = 4-MeO, R = 3,4-(MeO)2C6H3] to construction of brazilin core skeleton, in which In(OTf)3 showed superior result to FeCl3 (Scheme 9, lower).28
As the ring-closing reaction of the terminal propargyl compounds 1, CuI/(NH4)2HPO4-catalyzed formation reaction of chromenes 7 has been published (Scheme 11, upper).29,30 In contrast to FeCl3, the Cu(I)-catalytic systems have been described to bring about the yield of metathesis products 2, which as intermediates would lead to 7 by the subsequent Knoevenagel condensation in the presence of active methylene compounds under the similar conditions, although the mechanism analysis for the formation of 2 has been not carried out in detail. On the other hand, chromenone-tethered 6-alkynals 8 can be converted to endo-cyclic enones 9 by CuI/I2 catalytic systems (Scheme 11, lower).31 Bandyopadhyay et al. proposed transannulation-type [2+2] cycloaddition would involve in this reaction, and I2 would act as a σ-acid to coordinate carbonyl groups of aldehydes as well as CuI would activate the terminal alkyne as a π-acid. However, this procedure is limited to the reaction of terminal alkynes.
An approach to endo-cyclic enones, benzoxepinones 10 from the terminal propargyl compounds 1 has been achieved by Au(I)-catalyzed domino reactions, which proceed via heterocyclization of the in situ generated hemi-acetals followed by Petasis–Ferrier rearrangement of the resulting intermediates (Scheme 12).32 Although requiring an additional step of β-elimination of BnOH, this procedure can be applied not only to terminal alkynes (R2 = H) but also to internal alkynes (R2 = alkyl). In addition to this protocol, Au(I)-catalyzed ring-closing reactions of alkynyl ketones via difference route in the alkyne-carbonyl metathesis have been known.33
TFA can be used for the synthesis of polycyclic heterocycles via ring-closing reactions of heterocycle-tethered 1,6-ynals (Scheme 13).34 According to the report by Kim et al., pyrroles 11 were exposed to TFA media affording pyrroloquinolines 12 through the metathesis-type reaction between triple bonds and carbonyl groups.34a In addition to aldol mechanism indicated by Saá (Scheme 6b),25 [2+2] cycloaddition mechanism was suggested to take part in this reaction (Scheme 13, upper). In cases of indolizines 13, however, benzopyridoindoles 14 are formed prior to the metathesis products 15.34b The formation of 14 was explained to consist of hydroarylation by an attack of the C3 position of indolizine ring to triple bonds, followed by a deformylative aromatization of the intermediate C.
2.2. Ring-closing metathesis of alkynyl acetals
As shown in Scheme 6a, oxocarbenium ions consisting of carbonyl group and alcohol show high reactivity for alkyne-carbonyl metathesis22a so that the ring-closing metathesis reactions of alkynyl acetals through the generation of oxocarbenium ions can be realized. Yu and Li group reported a Fe(III)-catalyzed metathesis of alkynyl acetals 16 for the construction of five- to eight-membered heterocycles 17 (Scheme 14, upper).19a Computational studies on this reaction mechanism indicated the involvement of the stepwise [2+2] cycloaddition reactions of oxocarbenium D followed by the cycloreversion of intermediates F. The formation of F must overcome a barrier of 17.2 kcal/mol from E to transition state TS-1, and thus E is easily trapped by chloride anion to form product 18 in the presence of AcCl at a relatively low temperature (Scheme 14, lower).19a In similar to the formation of 18, Prins-type cyclization of alkynyl acetals has been known.19b,35
Taylor et al. demonstrated formic acid media was effective on the ring-closing reaction of alkynyl acetals.36 As shown in Scheme 15, 16 were rapidly converted to five- or six-membered cyclic enones 17 in formic acid at 100 ºC.36a The reaction of 16 at rt within 30 min led to a potential intermediate 19, which would be formed by a Prins-type process through an addition of HCO2H to intermediate E. In similar to the formation of 17, the exposure of 20 in formic acid brought about the formation of indolizidine 21 in excellent yield, and then five-step conversion from 21 gave grandisine D.36b 21 has been also used as a synthetic intermediate of elaeokanidine A.36c Furthermore, this procedure could be applied to the ring-closing reaction of 22 resulting in not only the yield of chromene 23a and dihydroquinoline 23b from internal alkynes (R = Pr) but also the yield of benzoxepins 24 and 25 from terminal alkynes (R = H, X = O).36a Very recently, ring-closing reactions of 22c, d were used for the synthetic approach to rotenoid natural products by Kim group (Scheme 16).37 In contrast to Taylor’s examples, formic acid media did not promote the formation of 23c, which is known as a synthetic intermediate of (±)-deguelin.38 On the other hand, In(OTf)3 in THF-H2O (4:1) brought about the high yield of 23c and 26 (with the deprotection of MOM group from 23d). Furthermore, the obtained 26 could be easily converted to (±)-munduserone.
2.3. Domino reactions based on intramolecular alkyne-carbonyl metathesis
Since α,β-enones are widely used as useful synthons in various conversion reactions catalyzed by Lewis acid and Brønsted acid, domino reactions via an alkyne-carbonyl metathesis has been found.39 As early examples based on the catalytic alkyne-carbonyl metathesis of unactivated alkynes, domino reactions with a Nazarov cyclization has been known (Scheme 17).20,40 In 2008, Saito and Hanzawa group reported a highly stereoselective synthesis of trans-2,3-disubstituted indanones 29 through the intermolecular metathesis of phenylalkynes 27 and aldehydes, in which SbF5-alcohol complexes activates the aldehydes and intermediate 28 as a σ-acid catalyst (Scheme 17a).20 In the same year, Jin and Yamamoto group reported the similar domino reactions via the intramolecular metathesis of 6-alkynyl ketones 30 (n = 2) caused by both σ- and π-acidity of cationic gold (III) catalyst (Scheme 17b).40 Notable, the formation of polycyclic compounds 31 (n = 1) were efficiently catalyzed by TfOH in MeOH, which was effective for the ring-closing metathesis of 5-alkynyl ketones as shown in Scheme 6a.22a
The present domino reaction can be used not only for the formation of carbocycles21c but also for the formation of heterocycles (Scheme 18).41 Thus, Saá et al. have succeeded in the developing the synthetic method of bicyclic azepane 33 by means of HBF4-mediated domino reactions of nitrogen-tethered alkynyl acetals 32, in which the formation of each product isomer depends on the proton (Ha, Hb, or Hc) removing in the Nazarov intermediate G. In cases of β-substituted 33a (R1 = H, R2 = Me), regardless of the geometry of 32a, the elimination of the proton proceeded preferably in order to Ha and Hb. In cases of α-substituted 33b (R1 = tBu, R2 = H), the elimination of Hb proceeded preferably along with the elimination of Hc. Such an isomeric formation of product was observed in the preparation of 31 (m = 3, 4, Scheme 17b).22a,40
Domino alkyne-carbonyl metathesis approaches to polycyclic carbocycles or heterocycles triggered by an incorporation of carbonyl equivalents into alkyne compounds have been achieved by some groups (Scheme 19, 20).42,43
In the synthesis of naphthalene derivatives 36 by Manojveer and Balamurugan, alkynyl acetal intermediates 35 were generated by TfOH-catalyzed aldol-type reactions of o-alkynylacetophenone 34 with orthoformate (Scheme 19, upper).42a In the ring-closing reaction of intermediates 35, Prins-type process of oxonium H was considered to be involved along with [2+2] cycloaddition pathway. The authors extended the synthetic method of naphthalene derivatives 36 to the formation of bicyclic or tricyclic benzenes 39 from alkynal 37 and ketone, in which the ring-closing reaction of intermediates 38 was also considered to consist of [2+2] cycloaddition and/or Prins-type pathway (Scheme 19, lower).42b Siddiqui et al. reported In(III)-catalyzed synthesis of spiro dihydrofuran oxindoles 43 by ring-closing metathesis of alkynyl ketone intermediate 42, which formed by the three-component reaction of isatins 40, terminal alkynes 27 and phenacyl bromides 41 (Scheme 20).43
Yeh et al. have succeeded the development of domino reactions based on a semipinacol rearrangement, which led to conversion of alkynyl epoxides to alkynyl ketone intermediates (Scheme 21).44 For example, alkynyl epoxides 44 were treated with TfOH catalysts to afford spiropiperidines 46, in which ring-opening of oxirane of 44 brought about the ring contraction of cyclohexanol parts followed by the ring-closing metathesis of alkynals 45 (Scheme 21, upper).44a Due to the isolation of the alkynal 45a (R = Ph) in the model reaction, 45 would take part into these reactions as a key intermediate. Since spiropiperidines 46 were obtained as a diastereomer mixture, 46 were subject to IBX oxidation and characterized as ketone 47. In cases of alkynyl epoxides 48, the C-O bond at the quaternary α-carbon was cleaved in the presence of Tf2NH to form 6-alkynyl ketones 49, which were converted to fused piperidines 50, via H-1,2-shift (Scheme 21, middle).44b On the other hand, alkynyl epoxides 51 bearing quaternary carbons at both α- and β-position underwent the cleavage of C-O bond at β-position in the presence of a catalytic amount of TMSOTf (10 mol%) giving rise to the yield of 5-alkynyl ketones 52.44b Since the ring-closing metathesis of 52 requires a small excess amount of Lewis acid, 1.2 equiv of TMSOTf was used in the domino reaction for the formation of metathesis products 53, which were isolated as pyrroles 54 after the basic workup (Scheme 21, lower). In these reactions, substituent effects of alkyne were observed and thus terminal, aliphatic and electron-deficient aromatic alkynes showed little or no conversion to desired product (Scheme 21).44
3. Syntheses of heterocycles via intErmolecular alkyne-carbonyl metathesis
For the extension of intermolecular alkyne-carbonyl metathesis to synthetic methods of cyclic compounds, the development of domino reactions with cyclization of metathesis product is required.45 As shown in Scheme 17a, Saito and Hanzawa group developed the domino approach to indanones from phenylalkynes and aldehydes catalyzed by SbF5-alcohol complexes.20 And then the authors applied the domino reaction to the synthesis of heterocycles from o-alkynylaniline (Scheme 22).46 In the synthesis of dihydroquinolinones 57, SbF5•5MeOH, which can be prepared from a 1:5 mixture of SbF5 and MeOH, efficiently catalyzed the intermolecular metathesis of o-alkynylanilines 55 with aldehydes and the subsequent cyclization of enone intermediates 56.46a The key intermediates 56 can be isolated under similar conditions at lower temperature. The σ-acidity of the catalyst is crucial for these reactions because π-acid catalysts including AuCl3 have promoted the cyclization of 55 to indole compounds prior to the intermolecular reaction between 55 and aldehydes. SbF5•5MeOH can be employed for the synthesis of 4-chromanone 59 from the oxygen analogue 58 and aldehydes.47 Since iminoquinolines is generally difficult to be synthesized by the imination of low electrophilic dihydroquinolinones, three-component synthesis of iminoquinolines 62 have been found on the basis of synthetic method of dihydroquinolinones 57.46b These three-component reactions proceed smoothly by hexafluoroisopropanol (HFIP, pKa = 9.3) media without any catalyst rather than SbF5•5MeOH to give 62 with complete trans-selectivities. The present mechanism was proposed to consist of (1) formation of imine from aldehyde and aromatic amine, (2) metathesis of alkyne 61 and imine, and (3) cyclization of α,β-unsaturated imine intermediate.
Recently, two groups independently published domino reactions of o-alkynylphenols or -anilines with aldehydes (Scheme 23). In the analogue manner by Saito and Hanzawa group,20 Lu and Wang group developed the BF3-mediated synthesis of 4-chromanone 59 from o-alkynylphenols 58 having the electron-withdrawing group.48 In this report, the domino reaction has been extended to a one-pot synthesis of 4-chromenone 63 with DDQ oxidation of 59, although 4-chromenone 63 having electron-rich aromatic rings (Ar = 4-MeC6H4, 2-furyl) were converted to products 64 by the removal of aromatic rings. On the other hand, the synthesis of fused dihydroquinolines 66 by Ma et al. was considered to proceed through a Prins-type cyclization of iminium intermediates derived from 65 and aldehydes, which can be regarded as an alternative reaction pathway from o-alkynylanilines and aldehydes.49 For these reactions, Sc(OTf)3 catalytic systems were effective, and particularly the dual catalysts of Sc(OTf)3 and PhCO2H showed good yields of products from electron-deficient aldehydes (R = 4-NCC6H4, 4-MeOCOC6H4, etc). Such a dual catalyst showed its efficiency in the intermolecular alkyne-carbonyl metathesis.21c
In connection with alkyne-carbonyl metathesis/cyclization sequence of domino reactions, Balamurugan et al. found the TfOH-catalyzed domino approach to benzofluorenes 67 from o-alkynylbenzaldehydes 37 with aromatic alkynes 27 (Scheme 24).50 The addition of orthoformates is essential for the formation of 67, whereas the use of TfOH only afforded naphthalene 68. The isolated acetals 69 were treated with TfOH to form 67 regardless of the presence of orthoformates, and thus 69 would be initially formed as an intermediate. From results of some control experiments, the authors suggested metathesis intermediates J derived from 69 and 27 would undergo consecutive electrophilic cyclization reactions to yield 67.
Recently, as an efficient method for the construction of medium and large ring lactones, which does not require high-dilution conditions and a slow addition of substrates, ring-expansion metathesis of cyclic acetals 70 and silyloxy alkynes 7151 was found out by Sun et al. (Scheme 25).52 Interestingly, this reaction would involve an unprecedented ring conjunction mode of oxetene such as intermediates K, which led to seven- to ten-, and eighteen-membered lactones 72. In addition to BF3•Et2O as a promoter, collidine worked well as an additive for the reversible formation of pyridinium L with the highly unstable cyclic oxocarbenium intermediate derived from 70. Thus, L would be considered as a reservoir of the oxocarbenium intermediate to prevent its decomposition before reacting with 71. Furthermore, this lactone formation reaction could be applied to iterative ring expansions with hydrogenation and reductive acylation of the first obtained lactone 72a. Therefore, this protocol is in principle capable of assembling various medium and large lactones from a small ring acetal.
4. Conclusion
We have described a variety of procedures for the synthesis of heterocycles based on the catalytic alkyne-carbonyl metathesis of the unactivated alkynes. The progress of the alkyne-carbonyl metathesis chemistry for the last decades has brought about not only various ring-closing metathesis reactions but also domino approaches with the cyclization of metathesis intermediates and ring-expansion metathesis reactions, which are limited to the examples of silyloxy alkynes. These strategies have been successfully applied to the total syntheses of some important molecules. However, there is still a room for the improvement of the intermolecular metathesis of unactivated alkynes with simple ketones and other low electrophilic carbonyl compounds,12 although some groups reported [2+2] reactions of unactivated alkynes with trifluoroketones53 or electron-deficient alkynes with simple ketones.54 In the future, the alkyne-carbonyl metathesis chemistry would be further improved and a brilliant success would be brought to organic syntheses.
ACKNOWLEDGEMENTS
This work was partially supported by JSPS Grants-in-Aid for Scientific Research (C) Grant No 15K07852 and by The Naito Foundation.
References
1. Review for gold-catalyzed alkyne-carbonyl metathesis reaction has been recently reported. See, L. Liu, B. Xu, and G. B. Hammond, Beilstein J. Org. Chem., 2011, 7, 606.
2. G. Büchi, J. T. Kofron, E. Koller, and D. Rosenthal, J. Am. Chem. Soc., 1956, 78, 876.
3. Early examples: (a) D. Bryce-Smith, A. Gilbert, and M. G. Johnson, Tetrahedron Lett., 1968, 2863; (b) S. Farid, W. Kothe, and G. Pfundt, Tetrahedron Lett., 1968, 4147; (c) S. P. Pappas and N. A. Portnoy, J. Org. Chem., 1968, 33, 2200; (d) H. J. T. Bos and J. S. M. Boleij, Recl. Trav. Chim. Pays-Bas, 1969, 88, 465; (e) T. Miyamoto, Y. Shigemitsu, and Y. Odaira, Chem. Commun., 1969, 1410; (f) H. J. T. Bos, H. T. Van Der Bend, J. S. M. Boleij, C. J. A. Everaars, and H. Polman, Recl. Trar. Chim. Pays-Bas, 1972, 91, 65; (g) H. Polman, J. S. M. Boleij, and H. J. T. Bos, Recl. Trav. Chim. Pays-Bas, 1972, 91, 1088; (h) A. Mosterd, H. J. Matser, and H. J. T. Bos, Tetrahedron Lett., 1974, 15, 4179.
4. H. Vieregge, H. J. T. Bos, and J. F. Arens, Recl. Trav. Chim. Pays-Bas, 1959, 78, 664. CrossRef
5. (a) L. B. Bos and J. F. Arens, Recl. Trav. Chim. Pays-Bas, 1963, 82, 157; CrossRef (b) H. Vieregge, H. M. Schmidt, J. Renema, H. J. T. Bos, and J. F. Arens, Recl. Trav. Chim. Pays-Bas, 1966, 85, 929; CrossRef (c) R. Fuk and H. G. Viehe, Chem. Ber., 1970, 103, 564; CrossRef (d) Z. A. Krasnaya and V. F. Kucherov, Izv. Akad. Nauk SSSR, Ser. Khim., 1965, 110; (e) S. M. Makin, I. E. Mikerin, O. A. Shavrygina, G. A. Ermakova, and B. M. Arshava, Zh. Org. Khim., 1984, 20, 2317; (f) J. Pornet, B. Khouz, and L. Miginiac, Tetrahedron Lett., 1985, 26, 1861; CrossRef (g) J. Pornet, A. Rayadh, and L. Miginiac, Tetrahedron Lett., 1986, 27, 5479; CrossRef (h) C. J. Kowalski and S. Sakdarat, J. Org. Chem., 1990, 55, 1977; CrossRef (i) D. Zakarya, A. Rayadh, M. Samih, and T. Lakhlifi, T. Tetrahedron Lett., 1994, 35, 405; CrossRef (j) Uncatalyzed reaction: G. van den Bosch, H. J. T. Bos, and J. F. Arens, Recl. Trav. Chim. Pays-Bas, 1966, 85, 567.
6. Examples for synthetic applications: (a) D. Crich and J. Z. Crich, Tetrahedron Lett., 1994, 35, 2469; (b) P. Kraft, C. Denis, and W. Eichenberger, Eur. J. Org. Chem., 2001, 2363; CrossRef (c) A. S. Golubev, N. N. Sergeeva, L. Hennig, A. F. Kolomiets, and K. Burger, Tetrahedron, 2003, 59, 1389; CrossRef (d) C. Ran, W. Zhang, and L. M. Sayre, Tetrahedron Lett., 2004, 45, 7851. CrossRef
7. (a) W. J. Middleton, J. Org. Chem., 1965, 30, 1307; CrossRef (b) L. E. Friedrich and J. D. Bower, J. Am. Chem. Soc., 1973, 95, 6869; CrossRef (c) P. C. Martino and P. B. Shevlin, J. Am. Chem. Soc., 1980, 102, 5429; CrossRef (d) L. E. Friedrich and P. Y.-S. Lam, J. Org. Chem., 1981, 46, 306; CrossRef (e) First experimental evidence of oxetene intermediate in the Lewis acid-promoted process: M. Oblin, J.-M. Pons, J.-L. Parrain, and M. Rajzmann, Chem. Commun., 1998, 1619. CrossRef
8. M. Oblin, M. Rajzmann, and J.-M. Pons, Tetrahedron, 2001, 57, 3099. CrossRef
9. (a) C. J. Kowalski and K. W. Fields, J. Am. Chem. Soc., 1982, 104, 321; CrossRef (b) M. Shindo, Y. Sato, and K. Shishido, J. Am. Chem. Soc., 1999, 121, 6507. CrossRef
10. (a) M. Shindo, Y. Sato, and K. Shishido, Tetrahedron Lett., 1998, 39, 4857; CrossRef (b) M. Shindo, Y. Sato, and K. Shishido, J. Org. Chem., 2000, 65, 5443; CrossRef (c) M. Shindo, K. Matsumoto, S. Mori, and K. Shishido, J. Am. Chem. Soc., 2002, 124, 6840; CrossRef (d) M. Shindo, Y. Sato, T. Yoshikawa, R. Koretsune, and K. Shishido, J. Org. Chem., 2004, 69, 3912; CrossRef (e) M. Shindo, T. Yoshikawa, Y. Itou, S. Mori, T. Nishii, and K. Shishido, Chem. Eur. J., 2006, 12, 524; CrossRef (f) M. Shindo, T. Kita, T. Kumagai, K. Matsumoto, and K. Shishido, J. Am. Chem. Soc., 2006, 128, 1062; CrossRef (g) T. Yoshikawa, S. Mori, and M. Shindo, J. Am. Chem. Soc., 2009, 131, 2092; CrossRef (h) S. Umezu and M. Shindo, Tetrahedron Lett., 2013, 54, 6871; Reviews; CrossRef (i) M. Shindo and S. Mori, J. Synth. Org. Chem. Jpn., 2008, 66, 28; CrossRef (j) M. Shindo and S. Mori, Synlett, 2008, 2231. CrossRef
11. In an electrocyclic ring-opening reactions of cyclobutene analogues to give 1,3-dienyl compounds, the stereochemical process of geometrical isomers is referred to as “torquoselectivity”. See, (a) W. R. Dolbier, Jr., H. Koroniak, K. N. Houk, and C. Sheu, Acc. Chem. Res., 1996, 29, 471; (b) W. R. Dolbier, Jr., H. Koroniak, D. J. Burton, A. R. Bailey, G. S. Shaw, and S. W. Hansen, J. Am. Chem. Soc., 1984, 106, 1871; CrossRef (c) W. Kirmse, N. G. Rondan, K. N. Houk, J. Am. Chem. Soc., 1984, 106, 7989; CrossRef (d) E. A. Kallel, Y. Wang, D. C. Spellmeyer, and K. N. Houk, J. Am. Chem. Soc., 1990, 112, 6759. CrossRef
12. H. J. T. Bos and J. F. Arens, Recl. Trav. Chim. Pays-Bas, 1963, 82, 157. CrossRef
13. A. Hayashi, M. Yamaguchi, and M. Hirama, Synlett, 1995, 195.
14. (a) C. E. Harding and M. Hanack, Tetrahedron Lett., 1971, 12, 1253; (b) R. J. Balf, B. Rao, and L. Weiler, Can. J. Chem., 1971, 49, 3135; CrossRef (c) M. Hanack, C. E. Harding, and J.-L. Derocque, Chem. Ber., 1972, 105, 421; CrossRef (d) M. J. Chandy and M. Hanack, Arch. Pharm., 1975, 308, 578; CrossRef (e) C. E. Harding and G. R. Stanford, Jr., J. Org. Chem., 1989, 54, 3054; CrossRef (f) C. E. Harding and S. L. King, J. Org. Chem., 1992, 57, 883; CrossRef (g) J. R. Grunwell, M. F. Wempe, and J. Mitchell, Tetrahedron Lett., 1993, 34, 7163; CrossRef See, (h) A. Balog and D. P. Curran, J. Org. Chem., 1995, 60, 337; (i) A. Balog, S. J. Geib, and D. P. Curran, J. Org. Chem., 1995, 60, 345. CrossRef
15. M. F. Wempe and J. R. Grunwell, Tetrahedron Lett., 2000, 41, 6709. CrossRef
16. M. Curini, F. Epifano, F. Maltese, and O. Rosati, Synlett, 2003, 552. CrossRef
17. J. U. Rhee and M. J. Krische, Org. Lett., 2005, 7, 2493. CrossRef
18. T. Jin and Y. Yamamoto, Org. Lett., 2007, 9, 5259. CrossRef
19. (a) T.-Y. Xu, Q. Yang, D.-P. Li, J.-H. Dong, Z.-K. Yu, and Y.-X. Li, Chem. Eur. J., 2010, 16, 9264; CrossRef (b) Stoichiometric reaction: P. O. Miranda, D. D. Diaz, J. I. Padron, M. A. Ramirez, and V. S. Martin, J. Org. Chem., 2005, 70, 57. CrossRef
20. A. Saito, M. Umakoshi, M. Yagyu, and Y. Hanzawa, Org. Lett., 2008, 10, 1783. CrossRef
21. (a) [Rh(P(OPh)3)2]BF4: K. Tanaka, K. Sasaki, K. Takeishi, and K. Sugishima, Chem. Commun., 2005, 4711; (b) In(OTf)3-alcohol: K. Miura, K. Yamamoto, A. Yamanobe, K. Ito, H. Kinoshita, J. Ichikawa, and A. Hosomi, Chem. Lett., 2010, 39, 766; CrossRef (c) In(OTf)3-PhCO2H: L. Zhu, Z.-G. Xi, J. Lv, and S. Luo, Org. Lett., 2013, 15, 4496; CrossRef (d) Substoichiometric reaction using GaCl3: G. S. Viswanathan and C.-J. Li, Tetrahedron Lett., 2002, 43, 1613; (e) In some cases of Pt(II)-catalyzed hydrometalation/cyclization sequence of 1,5- and 1,6-alkynals, cyclic enones were obtained as byproducts. See, J.-T. Hong, M.-J. Kang, and H.-Y. Jang, Bull. Korean Chem. Soc., 2010, 31, 2085.
22. (a) T. Jin, F. Yang, C. Liu, and Y. Yamamoto, Chem. Commun., 2009, 3533; CrossRef (b) L. Liu, L. Wei, and J. Zhang, Adv. Synth. Catal., 2010, 352, 1920. CrossRef
23. Difluoro-λ3-bromane-induced formation of α,β-enones from alkynes and ethanol has been reported. In this reaction, in situ generated acetaldehyde undergo HBF4-promoted alkyne-carbonyl metathesis. See, M. Ochiai, A. Yoshimura, T. Mori, Y. Nishi, and M. Hirobe, J. Am. Chem. Soc., 2008, 130, 3742.
24. Although catalyzing the similar reactions of terminal alkynals, CpRu(II) complex promotes decarbonylation reactions of products. See, J. A. Varela, C. González-Rodríguez, S. G. Rubín, L. Castedo, and C. Saá, J. Am. Chem. Soc., 2006, 128, 9576.
25. C. González-Rodríguez, L. Escalante, A. L. Varela, and C. Saá, Org. Lett., 2009, 11, 1531. CrossRef
26. This procedure can be employed for the construction of prequinolizidine and pyrrolizidinone. See, K. C. M. Kurtz, R. P. Hsung, and Y. Zhang, Org. Lett., 2006, 8, 231. CrossRef
27. (a) K. Bera, S. Sarkar, S. Biswas, S. Maiti, and U. Jana, J. Org. Chem., 2011, 76, 3539; CrossRef (b) K. Bera, S. Jalal, S. Sarkar, and U. Jana, Org. Biomol. Chem., 2014, 12, 57; CrossRef (c) S. Jalal, K. Bera, S. Sarkar, K. Paul, and U. Jana, Org. Biomol. Chem., 2014, 12, 1759; CrossRef (d) See also, K. Bera, S. Sarkar, S. Jalal, and U. Jana, J. Org. Chem., 2012, 77, 8780.
28. Y. Jung and I. Kim, J. Org. Chem., 2015, 80, 2001. CrossRef
29. K. Kumari, D. S. Raghuvanshi, and K. N. Singh, Tetrahedron, 2013, 69, 82. CrossRef
30. TiCl4/tBuNH2-mediated ring-closing reaction of terminal alkynal derivatives with the similar regioselectivity is reported to procced via the hydroamination of tBuNH2 to alkynes followed by the intramolecular aldol condensation of the resulting enamine intermediates. See, G. Abbiati, A. Casoni, V. Canevari, D. Nava, and E. Rossi, Org. Lett., 2006, 8, 4839.
31. (a) S. Maiti, P. Biswas, J. Ghosh, M. G. B. Drew, and C. Bandyopadhyay, Tetrahedron, 2014, 70, 334; CrossRef (b) The authors reported a construction of endo-cyclic enones by pyridine-catalyzed reactions of chromenone-tethered 6-alkynals bearing internal alkynes in the presence of active methylene compounds. See, P. Biswas, J. Ghosh, S. Maiti, and C. Bandyopadhyay, Tetrahedron Lett., 2014, 55, 6882. CrossRef
32. (a) E. M. L. Sze, W. Rao, M. J. Koh, and P. W. H. Chan, Chem. Eur. J., 2011, 17, 1437; CrossRef (b) Examples of nitrogen analogues: E. M. L. Sze, M. J. Koh, Y. M. Tjia, W. Rao, and P. W. H. Chan, Tetrahedron, 2013, 69, 5558. CrossRef
33. (a) L.-P. Liu, D. Malhotra, R. S. Paton, K. N. Houk, and G. B. Hammond, Angew. Chem. Int. Ed., 2010, 49, 9132; CrossRef (b) L.-P. Liu, D. Malhotra, Z. Jin, R. S. Paton, K. N. Houk, and G. B. Hammond, Chem. Eur. J., 2011, 17, 10690. CrossRef
34. (a) M. Nayak and I. Kim, Org. Biomol. Chem., 2015, 13, 9697; CrossRef (b) Y. Jung and I. Kim, Org. Lett., 2015, 17, 4600. CrossRef
35. Fe(III) halides: (a) T. Xu, Z. Yu, and L. Wang, Org. Lett., 2009, 11, 2113; (b) D. Zheng, W. Gong, Z. Ma, B. Ma, X. Zhao, Z. Xie, and Y. Li, Tetrahedron Lett., 2011, 52, 314; TiCl4; (c) N. A. Nikolic, E. Gonda, C. P. D. Longford, N. T. Lane, and D. W. Thompson, J. Org. Chem., 1989, 54, 2748; CrossRef (d) Y.-H. Kim, K.-Y. Lee, C.-Y. Oh, J.-G. Yang, and W.-H. Ham, Tetrahedron Lett., 2002, 43, 837; TMSI; CrossRef (e) K. Takami, H. Yorimitsu, H. Shinokubo, S. Matsubara, and K. Oshima, Synlett, 2001, 293.
36. (a) J. D. Cuthbertson, A. A. Godfrey, W. P. Unsworth, and R. J. K. Taylor, Heterocycles, 2012, 84, 1013; CrossRef (b) J. D. Cuthbertson, A. A. Godfrey, and R. J. K. Taylor, Org. Lett., 2011, 13, 3976; CrossRef (c) J. D. Cuthbertson, W. P. Unsworth, C. L. Moody, and R. J. K. Taylor, Tetrahedron Lett., 2015, 56, 3123. CrossRef
37. M. Nayak and I. Kim, J. Org. Chem., 2015, 80, 11460. CrossRef
38. S. J. Pastine and D. Sames, Org. Lett., 2003, 5, 4053. CrossRef
39. Before the reports of domino reaction based on the catalytic alkyne-carbonyl metathesis, Curran et al. demonstrated that BF3•OEt2 (15 equiv) promoted domino reaction of alkyny1 acetals with cyclic silyl acyloins to give polycyclic enediones. See, ref. 12h, i. Shvo et al. reported the formation of cyclic enone from diyens in formic acid media, in which alkynyl ketones was proposed to be formed as an intermediate. See also, N. Menashe and Y. Shvo, J. Org. Chem., 1993, 58, 7434.
40. T. Jin and Y. Yamamoto, Org. Lett., 2008, 10, 3137. CrossRef
41. L. Escalante, C. González-Rodríguez, J. A. Varela, and C. Saá, Angew. Chem. Int. Ed., 2012, 51, 12316. CrossRef
42. (a) S. Manojveer and R. Balamurugan, Org. Lett., 2014, 16, 1712; CrossRef (b) S. Manojveer and R. Balamurugan, Eur. J. Org. Chem., 2015, 4254. CrossRef
43. I. R. Siddiqui, Rahila, S. Shamim, P. Rai, Shireen, M. A. Waseem, and A. A. H. Abumhdi, Tetrahedron Lett., 2013, 54, 6991. CrossRef
44. (a) M.-N. Lin, S.-H. Wu, and M.-C. P. Yeh, Adv. Synth. Catal., 2011, 353, 3290; CrossRef (b) M.-C. P. Yeh, M.-N. Lin, C.-H. Hsu, and C.-J. Liang, J. Org. Chem., 2013, 78, 12381. CrossRef
45. Recent examples of domino intramolecular alkyne-carbonyl metathesis approaches to the synthesis of aliphatic compounds: (a) I. Karpaviciene and I. Cikotiene, Org. Lett., 2013, 15, 224; (b) C. Trujillo, G. Sánchez-Sanz, I. Karpavičienė, U. Jahn, I. Čikotienė, and L. Rulíšek, Chem. Eur. J., 2014, 20, 10360; CrossRef (c) S. Dimova, K. Zaharieva, C. Jossifov, Z. Cherkezova-Zheleva, and I. Mitov, J. Chem. Technol. Metall., 2013, 48, 28.
46. (a) A. Saito, J. Kasai, Y. Odaira, H. Fukaya, and Y. Hanzawa, J. Org. Chem., 2009, 74, 5644; CrossRef (b) A. Saito, J. Kasai, T. Konishi, and Y. Hanzawa, J. Org. Chem., 2010, 75, 6980. CrossRef
47. Before reports by Saito and Hanzawa, Cho et al. reported the formation of α,β-enones from o-alkynylphenol and aldehydes mediated by TMSOTf (2 equiv). See, J. Y. Park, P. R. Ullapu, H. Choo, J. K. Lee, S.-J. Min, A. N. Pae, Y. Kim, D.-J. Baek, and Y. S. Cho, Eur. J. Org. Chem., 2008, 5461.
48. N. Wang, S. Cai, C. Zhou, P. Lu, and Y. Wang, Tetrahedron, 2013, 69, 647. CrossRef
49. C. Zhu and S. Ma, Angew. Chem. Int. Ed., 2014, 53, 13532. CrossRef
50. S. Manojveer and R. Balamurugan, Chem. Commun., 2014, 50, 9925. CrossRef
51. Examples for the alkyne-carbonyl metathesis of siloxyalkynes: (a) J. Sun, V. A. Keller, S. T. Meyer, and S. A. Kozmin, Adv. Synth. Catal., 2010, 352, 839; (b) V. Tran and T. G. Minehan, Org. Lett., 2012, 14, 6100; CrossRef (c) See also ref. 4h.
52. (a) W. Zhao, Z. Li, and J. Sun, J. Am. Chem. Soc., 2013, 135, 4680; CrossRef (b) See also, W. Zhao, Z. Wang, and J. Sun, Angew. Chem. Int. Ed., 2012, 51, 6209.
53. (a) K. Aikawa, Y. Hioki, N. Shimizu, and K. Mikami, J. Am. Chem. Soc., 2011, 133, 20092; CrossRef (b) K. Aikawa, N. Shimizu, K. Honda, Y. Hioki, and K. Mikami, Chem. Sci., 2014, 5, 410. CrossRef
54. (a) S. Asghari and A. Habibi, Phosphorus, Sulfur, Silicon Relat. Elem., 2005, 180, 2451; CrossRef (b) See also, M. Longchar, U. Bora, R. C. Boruah, and J. S. Sandhu, Synth. Commun., 2002, 32, 3611; (c) H. Fan, X. Wang, J. Zhao, X. Li, J. Gao, and S. Zhu, J. Fluorine Chem., 2013, 146, 1 CrossRef