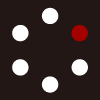
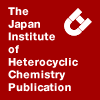
HETEROCYCLES
An International Journal for Reviews and Communications in Heterocyclic ChemistryWeb Edition ISSN: 1881-0942
Published online by The Japan Institute of Heterocyclic Chemistry
e-Journal
Full Text HTML
Received, 23rd March, 2015, Accepted, 21st April, 2015, Published online, 11th May, 2015.
DOI: 10.3987/COM-15-13213
■ Synthesis of 3-Ethenylindoles via Intramolecular Cyclization of Aryl Radical with Allene Generated by Samarium(II) Diiodide
Kenji Suzuki, Hiroki Iwasaki, Fumihito Ichiyoshi, Mao Tominaga, Naoto Kojima, Minoru Ozeki, and Masayuki Yamashita*
Kyoto Pharmaceutical University, 5 Misasagi-Nakauchi, Yamashina, Kyoto, Japan
Abstract
N-(2,3-Butadien-1-yl)-N-(tert-butoxycarbonyl)-2-iodoanilines cyclized intramolecularly to form 3-ethenyl-2,3-dihydroindoles after treatment with samarium(II) diiodide. The dihydroindoles were easily oxidized to the corresponding 3-ethenylindoles in good to moderate yields.As indole and dihydroindole scaffolds are found in various chemical agents, such as medicines and natural products, etc.,1 the development of new methods for their synthesis is one of the important subjects in synthetic chemistry (Figure 1). Various methods for their construction have been developed.2
Samarium(II) diiodide (SmI2), which is easily prepared from Sm metal and 1,2-diiodoethane, diiodomethane, iodine or so on, is a low-toxicity single-electron reducing agent.3 SmI2 can work in neutral conditions at room temperature or below, and SmI2-mediated reactions for the synthesis of complicated products exhibit functional group tolerance.4 In addition, the fact that SmI2 has high affinity for heteroatoms (oxygen, etc.) makes control of the reductive ability possible through the use of appropriate additives.5 Reactions mediated by SmI2 are classified into two types: 1) carbon-carbon bond formation reaction, such as the Barbier-type reaction and the pinacol-type reaction; and 2) functional group selective reduction, such as amide, ester, cyano, and nitro group reduction.6 In a previous communication, we reported an intramolecular cyclization reaction of N-allenyl-2-iodoanilines (1) with SmI2 to produce indole derivatives (2) (Scheme 1).7
In this paper, we report a novel method for the formation of 3-ethenylindole scaffolds under mild conditions.
A SmI2-mediated intramolecular cyclization of 3a having a methylene group between the allene group and the nitrogen atom was designed. The possible product would be tetrahydroquinoline (4) via 6-endo cyclization, dihydroquinoline (5) via 6-exo cyclization, or 2,3-dihydroindole (6a) via 5-exo cyclization (Scheme 2). Aniline having an allene group (3a) was subjected to the same reaction conditions as those described in Scheme 1, namely, SmI2 in the presence of hexamethylphosphoramide (HMPA) and i-PrOH. The radical cyclization proceeded selectively via 5-exo cyclization to afford 2,3-dihydroindole (6a) in high yield (Scheme 2). This fact was consistent with the result reported in the literatures.8
To examine the scope of the reaction, substrates 3b-h possessing a substituent on the aromatic ring were prepared and subjected to the same reaction conditions. The results are shown in Table 1.
The reaction of substrates possessing an electron-donating group, such as methoxy group 3b and methyl group 3c, afforded corresponding 6b and 6c in good yields (Entries 2 and 3). The reaction of substrates possessing an electron-withdrawing group, such as methoxycarbonyl group 3d and chloro group 3e, gave corresponding 6d and 6e in moderate yields (Entries 4 and 5). Judging from the results, the electronic density on the aromatic ring would be an important factor for the intramolecular cyclization of a phenyl radical onto an allene group. Next, the effect of substitution position was examined, and the reaction of substrate 3f, 3g or 3h having a methoxy group at 6-, 5- or 3-position afforded corresponding 6f, 6g or 6h in good yields, respectively (Entries 6-8).
Prepared dihydroindoles (6) were easily converted into corresponding 3-ethenylindoles (7) by oxidation with 2,3-dichloro-5,6-dicyano-1,4-benzoquinone (DDQ) in the usual manner (Table 2).9 The reaction of 6a afforded 7a in 70% yield (Entry 1). Substrates possessing an electron-donating group (6b and 6c) afforded corresponding 7b and 7c in good to moderate yields (Entries 2 and 3), and those possessing an electron-withdrawing group (6d and 6e) gave 7d and 7e in moderate yields (Entries 4 and 5). To clarify the effect of substitution position in the aromatic ring, the results of 6f-h having a methoxy group were compared with that of 6b. The reaction of 4-methoxy-substituted 6b gave the best yield (Entries 2, 6, 7, and 8). The reaction of 6-methoxy-substituted 6f afford 7f in only 9% yield. This is probably due to the steric interaction between the 6-methoxy group and the Boc group in 7f (Entry 6).10,11
In general, 3-ethenylindole derivatives are prepared by the Wittig reaction, Peterson reaction, and use of Nysted reagent of the corresponding 3-formylindoles,12 and are useful as building blocks in the functionalization of the ethenyl group,13 side chain elongation,14 and further ring formation by the Diels-Alder reaction,15 the Povarov reaction,16 or so on.17
In conclusion, we have developed an easy and useful method for the preparation of 3-ethenyldihydroindoles (6) and 3-ethenylindoles (7). The method involves treatment of N-(2,3-butadien-1-yl)-N-(tert-butoxycarbonyl)-2-iodoanilines (3) with samarium(II) diiodide to afford intramolecularly cyclized products (6). Subsequent oxidation with DDQ gave corresponding 7 in good to moderate yields in two steps.
EXPERIMENTAL
All reactions were performed using dried glasswares under an atmosphere of argon. Anhydrous THF and anhydrous toluene were purchased from Kanto Chemicals Inc. and used without further purification. HMPA was distilled from CaH2 under reduced pressure. All other chemicals were purchased at the highest commercial grade and used without further purification. Melting point was measured with a Yanaco MP micro-melting apparatus and uncorrected. NMR spectra were measured on JEOL JNM-LA-500 (1H: 500 MHz; 13C: 125 MHz), JEOL ECS-400 (1H: 400 MHz; 13C: 100 MHz), and Varian INOVA 400NB (1H: 400 MHz, 13C: 100 MHz) spectrometers with tetramethylsilane as an internal standard. Chemical shifts are reported in ppm. IR spectra were taken with Shimadu FTIR-8400 spectrophotometers. A JEOL JMS-GC mate spectrometer was used for low-resolution and high-resolution electron ionizations MS (LR-EIMS and HR-EIMS). Silica gel 60N (60-230 mesh, Kanto Chemical Co., Inc.) for column chromatography, silica gel 60 F254 pre-coated glass plates (0.25 mm-thickness, Merck) for analytical thin-layer chromatography (TLC) and silica gel 60 F254 (0.5 mm and 1.0 mm-thickness, Merck) for preparative TLC were used.
General procedure for samarium(II)-mediated cyclization of 3.
N-(tert-Butoxycarbonyl)-3-ethenyl-2,3-dihydroindole (6a)
A mixture of samarium (190 mg, 1.26 mmol) and 1,2-diiodoethane (266 mg, 0.943 mmol) in THF (9.0 mL) was stirred for 1.5 h at room temperature. After cooling to 0 ºC, HMPA (0.59 mL, 3.39 mmol) was added to the mixture, and stirring was continued for 20 min at 0 ºC. A solution of allene 3a (70.0 mg, 0.189 mmol) and i-PrOH (0.03 mL, 0.377 mmol) in THF (4.6 mL) was added dropwise to the mixture, and the mixture was stirred for additional 20 min. After the mixture was exposed to air, saturated NaHCO3 was added to the mixture, and the whole was extracted with Et2O. The extracts were washed with saturated aq. NaHCO3 and brine, and dried over MgSO4. The filtrate was concentrated under reduced pressure to leave a residue, which was purified by column chromatography over silica gel with n-hexane- EtOAc (12:1) to give 6a (44.7 mg, 97% yield).
Colorless oil; IR (CHCl3) cm-1; 1693; 1H NMR (500 MHz, CDCl3) δ 1.56 (s, 9H), 3.68 (brs, 1H), 3.95 (q, J = 8.5 Hz, 1H), 4.13-4.26 (m, 1H), 5.14 (dd, J = 10.0, 1.0 Hz, 1H), 5.20 (dt, J = 17.0, 1.0 Hz, 1H), 5.83 (ddd, J = 17.0, 10.0, 8.0 Hz, 1H), 6.95 (td, J = 7.5, 1.0 Hz, 1H), 7.08 (d, J = 7.5 Hz, 1H), 7.19 (t, J = 7.5 Hz, 1H), 7.78-7.92 (m, 1H); 13C NMR (125 MHz, CDCl3) δ 28.4 (3C), 44.5, 53.6, 81.5, 98.0, 114.7, 116.4, 122.3 (2C), 124.6, 128.0, 138.6, 152.4; LR-EIMS m/z (%) 245 (M+, 25.7), 190 (13), 189 (100), 145 (27), 144 (23), 130 (13), 117 (12), 57 (69); HR-EIMS calcd for C15H19NO2 (M+): 245.1416; found: 245.1410.
N-(tert-Butoxycarbonyl)-3-ethenyl-2,3-dihydro-5-methoxyindole (6b)
2,3-Dihydroindole 6b was synthesized with a similar manner to 6a. Colorless oil (149 mg, 89%); IR (CHCl3) cm-1; 1686; 1H NMR (400 MHz, CDCl3) δ 1.55 (brs, 9H), 3.61-3.72 (m, 1H), 3.76 (s, 3H), 3.88-3.97 (m, 1H), 4.12-4.25 (m, 1H), 5.15 (ddd, J = 10.0, 1.2, 0.4 Hz, 1H), 5.21 (dt, J = 17.2, 1.2 Hz, 1H), 5.82 (ddd, J = 17.2, 10.0, 8.4 Hz, 1H), 6.65 (dd, J = 2.8, 0.8 Hz, 1H), 6.72 (dd, J = 8.8, 2.8 Hz, 1H), 7.68-7.81 (m, 1H); 13C NMR (100 MHz, CDCl3) δ 28.5 (3C), 44.8, 53.8, 55.7, 80.3, 110.9, 112.8, 113.2, 115.2, 116.1, 116.6, 138.3, 152.3, 155.5; LR-EIMS m/z (%) 275 (M+, 18.7), 220 (19), 219 (100), 175 (17), 174 (12), 160 (30), 57 (20); HR-EIMS calcd for C16H21NO3 (M+): 275.1521; found: 275.1524.
N-(tert-Butoxycarbonyl)-3-ethenyl-2,3-dihydro-5-methylindole (6c)
2,3-Dihydroindole 6c was synthesized with a similar manner to 6a. Colorless oil (163 mg, 81%); IR (CHCl3) cm-1; 1686; 1H NMR (400 MHz, CDCl3) δ 1.56 (s, 9H), 2.29 (s, 3H), 3.58-3.78 (m, 1H), 3.91 (q, J = 8.4 Hz, 1H), 4.18 (t, J = 10.0 Hz, 1H), 5.14 (dd, J = 10.0, 1.2 Hz, 1H), 5.20 (dt, J = 16.8, 1.2 Hz, 1H), 5.82 (ddd, J = 16.8, 10.0, 8.4 Hz, 1H), 6.89 (s, 1H), 6.99 (d, J = 8.0 Hz, 1H), 7.62-7.83 (m, 1H); 13C NMR (100 MHz, CDCl3) δ 20.8, 28.5 (3C), 44.6, 53.7, 80.4, 114.4, 116.3, 125.3, 128.4, 131.8 (2C), 138.7 (2C), 152.4; LR-EIMS m/z (%) 259 (M+, 25.0), 204 (18), 203 (100), 188 (11), 159 (25), 158 (27), 144 (25), 132 (11), 131 (16), 57 (33); HR-EIMS calcd for C16H21NO2 (M+): 259.1572; found: 259.1566.
N-(tert-Butoxycarbonyl)-3-ethenyl-2,3-dihydro-5-methoxycarbonylindole (6d)
2,3-Dihydroindole 6d was synthesized with a similar manner to 6a. Colorless prisms (23.8 mg, 48%); mp 118-20 ºC (n-hexane-AcOEt = 10:1); IR (KBr) cm-1; 1710; 1H NMR (400 MHz, CDCl3) δ 1.57 (s, 9H), 3.74 (dd, J = 10.8, 7.6 Hz, 1H), 3.87 (s, 3H), 3.97 (q, J = 8.8 Hz, 1H), 4.24 (t, J = 10.8 Hz, 1H), 5.19 (dt, J = 10.0, 1.2 Hz, 1H), 5.23 (dt, J = 16.8, 1.2 Hz, 1H), 5.83 (ddd, J = 16.8, 10.0, 8.4 Hz, 1H), 7.77-7.96 (m, 1H), 7.74 (s, 1H), 7.92 (dd, J = 8.0, 0.8 Hz, 1H); 13C NMR (100 MHz, CDCl3) δ 28.3 (3C), 43.7, 51.8, 54.0, 81.5, 113.9, 117.0, 124.0, 126.1, 130.6, 133.2, 137.8, 146.6, 152.0, 166.7; LR-EIMS m/z (%) 303 (M+, 21.9), 248 (18), 247 (100), 216 (12), 203 (31), 202 (11), 188 (32), 144 (19), 115 (11), 57 (61); HR-EIMS calcd for C17H21NO4 (M+): 303.1471; found: 303.1474.
N-(tert-Butoxycarbonyl)-5-chloro-3-ethenyl-2,3-dihydroindole (6e)
2,3-Dihydroindole 6e was synthesized with a similar manner to 6a. Colorless oil (29.9 mg, 62%); IR (CHCl3) cm-1; 1697; 1H NMR (400 MHz, CDCl3) δ 1.55 (s, 9H), 3.69 (t, J = 8.8 Hz, 1H), 3.93 (q, J = 8.8 Hz, 1H), 4.20 (t, J = 8.8 Hz, 1H), 5.16-5.24 (m, 2H), 5.81 (ddd, J = 17.2, 10.0, 8.8 Hz, 1H), 7.02-7.04 (m, 1H), 7.14 (dd, J = 8.8, 2.0 Hz, 1H), 7.58-7.89 (m, 1H); 13C NMR (100 MHz, CDCl3) δ 28.4 (3C), 44.3, 53.8, 81.0, 115.6, 116.3, 117.1, 119.6, 124.9, 127.2, 127.9, 137.7, 152.2; LR-EIMS m/z (%) 281 (M++2, 15), 279 (M+, 42.3), 225 (35), 224 (13), 223 (100), 179 (11); HR-EIMS calcd for C15H18ClNO2 (M+): 279.1026; found: 279.1022.
N-(tert-Butoxycarbonyl)-3-ethenyl-2,3-dihydro-7-methoxyindole (6f)
2,3-Dihydroindole 6f was synthesized with a similar manner to 6a. Colorless oil (186 mg, 90%); IR (CHCl3) cm-1; 1689; 1H NMR (400 MHz, CDCl3) δ 1.50 (s, 9H), 3.74 (dd, J = 11.2, 8.0 Hz, 1H), 3.86 (s, 3H), 3.92 (q, J = 8.0 Hz, 1H), 4.30 (dd, J = 11.2, 8.0 Hz, 1H), 5.15-5.25 (m, 2H), 5.79 (ddd, J = 17.2, 10.4, 8.4 Hz, 1H), 6.74 (dt, J = 8.0, 1.2 Hz, 1H), 6.80 (d, J = 8.0 Hz, 1H), 7.04 (dd, J = 8.0, 7.2 Hz, 1H); 13C NMR (100 MHz, CDCl3) δ 28.2 (3C), 46.6, 55.6, 56.9, 80.4, 111.7, 116.6, 117.1, 125.4, 131.3, 137.6, 138.1, 149.8, 153.8; LR-EIMS m/z (%) 275 (M+, 32.9), 219 (24), 202 (13), 176 (15), 175 (100), 174 (51), 160 (49), 159 (12), 147 (22), 57 (72); HR-EIMS calcd for C16H21NO3 (M+): 275.1521; found: 275.1518.
N-(tert-Butoxycarbonyl)-3-ethenyl-2,3-dihydro-6-methoxyindole (6g)
2,3-Dihydroindole 6g was synthesized with a similar manner to 6a. Pale yellow oil (141 mg, 85%); IR (CHCl3) cm-1; 1697; 1H NMR (400 MHz, CDCl3) δ 1.57 (s, 9H), 3.69 (t, J = 8.4 Hz, 1H), 3.79 (s, 3H), 3.88 (q, J = 8.4 Hz, 1H), 4.19 (t, J = 8.4 Hz, 1H), 5.08-5.20 (m, 2H), 5.81 (ddd, J = 17.2, 10.0, 8.4 Hz, 1H), 6.50 (dd, J = 8.4, 2.4 Hz, 1H), 6.94 (d, J = 8.4 Hz, 1H), 7.46-7.61 (m, 1H); 13C NMR (100 MHz, CDCl3) δ 28.4 (3C), 43.7, 54.3, 55.4, 80.6, 100.5, 101.5, 107.6, 108.8, 115.9, 124.9, 138.9, 152.2, 156.0; LR-EIMS m/z (%) 275 (M+, 21.6), 220 (15), 219 (100), 192 (12), 175 (17), 174 (25), 160 (28), 148 (15), 147 (11), 85 (41), 83 (62), 58 (14), 57 (41); HR-EIMS calcd for C16H21NO3 (M+): 275.1521; found: 275.1526.
N-(tert-Butoxycarbonyl)-3-ethenyl-2,3-dihydro-4-methoxyindole (6h)
2,3-Dihydroindole 6h was synthesized with a similar manner to 6a. Colorless oil (181 mg, 88%); IR (CHCl3) cm-1; 1690; 1H NMR (400 MHz, CDCl3) δ 1.56 (s, 9H), 3.80 (s, 3H), 3.82-4.01 (m, 2H), 4.05 (dd, J = 10.8, 9.6 Hz, 1H), 5.02-5.09 (m, 2H), 5.92 (ddd, J = 17.2, 10.0, 6.8 Hz, 1H), 6.52 (d, J = 8.4 Hz, 1H), 7.16 (t, J = 8.4 Hz, 1H), 7.36-7.66 (m, 1H); 13C NMR (100 MHz, CDCl3) δ 28.4 (3C), 41.1, 53.8, 55.3, 80.3, 105.1, 107.9 (2C), 114.4 (2C), 129.4, 138.3, 152.4, 156.4; LR-EIMS m/z (%) 275 (M+, 31.3), 220 (15), 219 (100), 204 (17), 191 (11), 175 (25), 174 (24), 160 (35), 148 (15), 147 (20), 85 (34), 83 (51), 57 (63); HR-EIMS calcd for C16H21NO3 (M+): 275.1521; found: 275.1519.
General procedure for oxidation of dihydroindoles 6.
N-(tert-Butoxycarbonyl)-3-ethenyl-5-methoxyindole (7b)
DDQ (65.2 mg, 0.287 mmol) was added to a solution of 6b (65.9 mg, 0.239 mmol) in toluene (2.4 mL) at room temperature and the mixture was heated at 80 ºC with stirring for 3 h. The mixture was extracted with EtOAc. The extracts were washed with H2O and brine, and dried over MgSO4. The filtrate was concentrated under reduce pressure to leave a residue, which was purified by column chromatography over silica gel with n-hexane-EtOAc (12:1) to give 7b (58.5 mg, 89%).
Pale yellow oil; IR (CHCl3) cm-1; 1726; 1H NMR (400 MHz, CDCl3) δ 1.66 (s, 9H), 3.88 (s, 3H), 5.31 (d, J = 11.2 Hz, 1H), 5.76 (d, J = 17.6 Hz, 1H), 6.78 (dd, J = 17.6, 11.2 Hz, 1H), 6.95 (dd, J = 8.8, 2.4 Hz, 1H), 7.24 (d, J = 2.4 Hz, 1H), 7.60 (brs, 1H), 8.04 (d, J = 8.0 Hz, 1H); 13C NMR (100 MHz, CDCl3) δ 28.2 (3C), 55.8, 83.7, 103.0, 110.8, 113.0 (2C), 114.1, 116.0 (2C), 119.0, 124.5, 128.1, 156.0; LR-EIMS m/z (%) 273 (M+, 66.1), 219 (16), 218 (14), 217 (100), 173 (32), 57 (10); HR-EIMS calcd for C16H19NO3 (M+): 273.1365; found: 273.1363.
N-(tert-Butoxycarbonyl)-3-ethenylindole (7a)12c,f,g,k,18
3-Ethenylindole 7a was synthesized with a similar manner to 7b. Pale yellow oil (66 mg, 70%).
N-(tert-Butoxycarbonyl)-3-ethenyl-5-methylindole (7c)
3-Ethenylindole 7c was synthesized with a similar manner to 7b. Pale yellow oil (42.6 mg, 61%); IR (CHCl3) cm-1; 1726; 1H NMR (400 MHz, CDCl3) δ 1.66 (s, 9H), 2.46 (s, 3H), 5.30 (dd, J = 11.2, 1.2 Hz, 1H), 5.79 (dd, J = 17.6, 1.2 Hz, 1H), 6.79 (ddd, J = 17.6, 11.2, 0.8 Hz, 1H), 7.15 (dd, J = 8.4, 1.2 Hz, 1H), 7.57-7.67 (m, 2H), 8.02 (d, J = 8.4 Hz, 1H); 13C NMR (100 MHz, CDCl3) δ 21.5. 28.2 (3C), 83.6, 114.1, 114.9, 119.0, 119.9, 124.0, 125.9, 128.2, 128.9, 132.3, 134.1, 149.6; LR-EIMS m/z (%) 257 (M+, 28.5), 202 (11), 201 (76), 158 (16), 157 (100), 156 (29), 85 (25), 83 (39), 57 (55); HR-EIMS calcd for C16H19NO2 (M+): 257.1416; found: 257.1410.
N-(tert-Butoxycarbonyl)-3-ethenyl-5-methoxycarbonylndole (7d)
3-Ethenylindole 7d was synthesized with a similar manner to 7b. Pale yellow oil (20.8 mg, 63%); IR (KBr) cm-1; 1722; 1H NMR (400 MHz, CDCl3) δ 1.68 (s, 9H), 3.96 (s, 3H), 5.38 (dd, J = 11.2, 0.8 Hz, 1H), 5.87 (dd, J = 17.6, 0.8 Hz, 1H), 6.82 (dd, J = 17.6, 11.2 Hz, 1H), 7.67 (s, 1H), 8.04 (dd, J = 8.8, 1.6 Hz, 1H), 8.21 (d, J = 8.8 Hz, 1H), 8.51 (d, J = 1.6 Hz, 1H); 13C NMR (125 MHz, CDCl3) δ 28.2 (3C), 52.1, 84.5, 114.8, 119.6, 122.0, 122.3, 124.8, 125.9, 126.2, 127.5, 128.5, 138.5, 149.2, 167.5; LR-EIMS m/z (%) 301 (M+, 34.2), 247 (16), 246 (18), 245 (89), 214 (15), 202 (21), 201 (95), 170 (52), 142 (13), 115 (20), 114 (19), 57 (100): HR-EIMS calcd for C17H19NO4 (M+): 301.1314; found: 301.1319.
N-(tert-Butoxycarbonyl)-5-chloro-3-ethenylindole (7e)
3-Ethenylindole 7e was synthesized with a similar manner to 7b. Pale yellow oil (31.4 mg, 41%); IR (CHCl3) cm-1; 1732; 1H NMR (400 MHz, CDCl3) δ 1.67 (s, 9H), 5.33 (dd, J = 11.2, 1.2 Hz, 1H), 5.76 (dd, J = 18.0, 1.2 Hz, 1H), 6.74 (ddd, J = 18.0, 11.2, 1.2 Hz, 1H), 7.28 (dd, J = 8.8, 2.0 Hz, 1H), 7.62 (s, 1H), 7.74 (d, J = 2.0 Hz, 1H), 8.08 (d, J = 8.8 Hz, 1H); 13C NMR (100 MHz, CDCl3) δ 28.1 (3C), 84.3, 116.3, 118.7, 119.5, 119.8, 124.6, 124.9, 127.5, 128.7, 129.9, 134.3, 149.2; LR-EIMS m/z (%) 279 (M++2, 20), 277 (M+, 53.4), 223 (37), 222 (13), 221 (100), 179 (18), 177 (54), 57 (29); HR-EIMS calcd for C15H16ClNO2 (M+): 277.0870; found: 277.0865.
N-(tert-Butoxycarbonyl)-3-ethenyl-7-methoxyindole (7f)
3-Ethenylindole 7f was synthesized with a similar manner to 7b. Pale yellow oil (3.1 mg, 9%); IR (CHCl3) 1722 cm-1; 1H NMR (300 MHz, CDCl3) δ 1.63 (s, 9H), 3.94 (s, 3H), 5.29 (d, J = 11.1 Hz, 1H), 5.75 (d, J = 18.0 Hz, 1H), 6.79 (dd, J = 11.1, 18.0 Hz, 1H), 6.85 (d, J = 7.8 Hz, 1H), 7.23 (t, J = 7.8 Hz, 1H), 7.39 (d, J = 7.8 Hz, 1H), 7.57 (s, 1H); 13C NMR (100 MHz, CDCl3) δ 27.9 (3C), 55.8, 83.4, 107.1, 112.4, 113.9, 118.7, 123.9, 126.4 (2C), 128.0, 131.6, 148.3, 149.4; LR-EIMS m/z (%) 273 (M+, 76.2), 274 (14), 217 (12), 173 (100), 130 (18); HR-EIMS calcd for C16H19NO3 (M+): 273.1365; found: 273.1360.
N-(tert-Butoxycarbonyl)-3-ethenyl-6-methoxyindole (7g)
3-Ethenylindole 7g was synthesized with a similar manner to 7b. Pale yellow oil (43.1 mg, 62%); IR (CHCl3) cm-1; 1724; 1H NMR (400 MHz, CDCl3) δ 1.67 (s, 9H), 3.88 (s, 3H), 5.30 (dd, J = 11.6, 1.2 Hz, 1H), 5.77 (dd, J = 18.0, 1.2 Hz, 1H), 6.76 (ddd, J = 18.0, 11.6, 0.8 Hz, 1H), 6.91 (dd, J = 8.8, 2.4 Hz, 1H), 7.50 (s, 1H), 7.66 (d, J = 8.8 Hz, 1H), 7.77 (brs, 1H); 13C NMR (100 MHz, CDCl3) δ 28.2 (3C), 55.6, 83.7, 99.4, 112.2, 114.1, 119.2, 120.5 (2C), 122.6, 122.9, 128.3, 149.6, 157.9; LR-EIMS m/z (%) 273 (M+, 65.5), 274 (12), 219 (10), 218 (15), 217 (100), 173 (13); HR-EIMS calcd for C16H19NO3 (M+): 273.1365; found: 273.1371.
N-(tert-Butoxycarbonyl)-3-ethenyl-4-methoxyindole (7h)
3-Ethenylindole 7h was synthesized with a similar manner to 7b. Colorless oil (43.4 mg, 63%); IR (CHCl3) cm-1; 1726; 1H NMR (400 MHz, CDCl3) δ 1.66 (s, 9H), 3.91 (s, 3H), 5.17 (dd, J = 11.2, 1.6 Hz, 1H), 5.64 (dd, J = 17.6, 1.6 Hz, 1H), 6.67 (d, J = 8.0 Hz, 1H), 7.19-7.29 (m, 2H), 7.62 (s, 1H), 7.76 (d, J = 8.0 Hz, 1H); 13C NMR (100 MHz, CDCl3) δ 28.1 (3C), 55.3, 83.7, 103.6, 108.3, 113.2, 118.5, 120.0, 120.1, 125.2, 129.8, 137.0, 149.6, 154.4; LR-EIMS m/z (%) 273 (M+, 28.7), 218 (15), 217 (100), 174 (11), 173 (72), 172 (21), 158 (30), 146 (16), 130 (23), 115 (16), 85 (22), 83 (33), 57 (64); HR-EIMS calcd for C16H19NO3 (M+): 273.1365; found: 273.1372.
ACKNOWLEDGEMENTS
This work was financially supported in part by a Strategic Research Foundation Grant-Aided Project for Private Universities from the Ministry of Education, Culture, Sports, Science and Technology of Japan (MEXT) and Kyoto Pharmaceutical University Fund for the Promotion of Scientific Research.
References
1. For recent reports: (a) T. J. Maimone, Y. Ishihara, and P. S. Baran, Teterahedron, 2014, 70, 1; CrossRef (b) S. Hanessian and E. J. L. Stoffman, Can. J. Chem., 2013, 91, 13; CrossRef (c) S. Biswal, U. Sahoo, S. Sethy, H. K. S. Kumar, and M. Banerjee, Asian J. Pharm. Clin. Res., 2012, 5, 1; (d) W. Liu, H. J. Lim, and T. V. RajanBabu, J. Am. Chem. Soc., 2012, 134, 5496; CrossRef (e) E. J. L. Stoffman and D. L. J. Clive, Tetrahedron, 2010, 66, 4452; CrossRef (f) T. Benkovics, I. A. Guzei, and T. P. Yoon, Angew. Chem. Int. Ed., 2010, 49, 9153; CrossRef (g) A. Steven and L. E. Overman, Angew. Chem. Int. Ed., 2007, 46, 5488; CrossRef (h) Y. J. Chyan, B. Poeggeler, R. A. Omar, D. G. Chain, B. Frangione, J. Ghiso, and M. A. Pappolla, J. Biol. Chem., 1999, 274, 21937. For recent reviews; CrossRef (i) S. E. O’Connor and J. J. Maresh, Nat. Prod. Rep., 2006, 23, 532; CrossRef (j) M. Somei and F. Yamada, Nat. Prod. Rep., 2005, 22, 73. CrossRef
2. For recent reports: (a) Z. Shi and F. Glorius, Angew. Chem. Int. Ed., 2012, 51, 9220; CrossRef (b) D. Zhang, H. Song, and Y. Qin, Acc. Chem. Res., 2011, 44, 447; CrossRef (c) J. Alvarez-Builla, J. J. Vaquero, and J. Barluenga, Mod. Heterocycl. Chem., 2011, 1, 377; (d) D. F. Taber and P. K. Tirunahari, Tetrahedron, 2011, 67, 7195; CrossRef (e) D. Liu, G. Zhao, and L. Xiang, Eur. J. Org. Chem., 2010, 21, 3975; CrossRef (f) S. Anas and H. B. Kagan, Tetrahedron: Asymmetry, 2009, 20, 2193; CrossRef (g) Y.-Q. Fang and M. Lautens, J. Org. Chem., 2008, 73, 538; CrossRef (h) M. Arisawa, Y. Terada, K. Takahashi, M. Nakagawa, and A. Nishida, Chem. Rec., 2007, 7, 238; CrossRef (i) L. Ackermann, Synlett, 2007, 507; CrossRef (j) K. Aoki, A. J. Peat, and S. L. Buchwald, J. Am. Chem. Soc., 1998, 120, 3068; CrossRef (k) C. Chen, D. R. Liberman, R. D. Larsen, T. R. Verhoeven, and P. J. Reider, J. Org. Chem., 1997, 62, 2676. For recent reviews; CrossRef (l) M. Inman and C. J. Moody, Chem. Sci., 2013, 4, 29; CrossRef (m) S. Cacchi and G. Fabrizi, Chem. Rev., 2011, 111, PR215; CrossRef (n) O. Miyata, N. Takeda, and T. Naito, Heterocycles, 2009, 78, 843; CrossRef (o) G. Zeni and R. C. Larock, Chem. Rev., 2006, 106, 4644; CrossRef (p) J. Barluenga and C. Valdés, 'Five-Membered Heterocycles: Indole and Related Systems' in Modern Heterocyclic Chemistry, 1st edn., ed. by J. Julio Alvarez-Builla, J. Jose, and J. Barluenga, Wiley-VCH Verlag GmbH & Co. KGaA, 2011, 377.
3. (a) P. Girard, J. L. Namy, and H. B. Kagan, J. Am. Chem. Soc., 1980, 102, 2693; (b) T. Imamoto and M. Ono, Chem. Lett., 1987, 501; CrossRef (c) G. A. Molander and C. Kenny, J. Org. Chem., 1991, 56, 1439; CrossRef (d) M. Szostak, M. Spain, and D. J. Procter, J. Org. Chem., 2012, 77, 3049 and the references cited therein.
4. For recent review: J. M. Concellon and H. Rodriguez-Solla, Eur. J. Org. Chem., 2006, 1613.
5. For recent reviews: (a) M. Szostak, M. Spain, D. Parmar, and D. J. Procter, Chem. Commun., 2012, 48, 330; CrossRef (b) R. A. Flowers II, Synlett, 2008, 1427; CrossRef (c) A. Dahlen and G. Hilmersson, Eur. J. Inorg. Chem., 2004, 3393. CrossRef
6. For recent reviews: (a) M. Szostak, N. J. Fazakerley, D. Parmar, and D. J. Procter, Chem. Rev., 2014, 114, 5959; (b) K. Gopalaiah and H. B. Kagan, Chem. Rec., 2013, 13, 187; CrossRef (c) H. B. Kagan, Tetrahedron, 2003, 59, 10351; CrossRef (d) D. Bradley, G. Williams, K. Blann, and J. Caddy, Org. Prep. Proced. Int., 2001, 33, 565; CrossRef (e) Y. Kamochi and T. Kudo, Heteroat. Chem., 1994, 165; (f) G. A. Molander, Org. React., 1994, 46, 211; (g) D. P. Curran, T. L. Fevig, C. P. Jasperse, and M. J. Totleben, Synlett, 1992, 943. CrossRef
7. H. Iwasaki, K. Suzuki, M. Yamane, S. Yoshida, N. Kojima, M. Ozeki, and M. Yamashita, Org. Biomol. Chem., 2014, 12, 6812. CrossRef
8. (a) R. Zeng, C. Fu, and S. Ma, Angew. Chem. Int. Ed., 2012, 51, 3888; CrossRef (b) J. Shi, M. Zhang, Y. Fu, L. Liu, and Q.-X. Guo, Tetrahedron, 2007, 63, 12681; CrossRef (c) M. Apparu and J. K. Crandall, J. Org. Chem., 1984, 49, 2125. CrossRef
9. For recent reports: (a) Yi. He, X. Li, J. Li, X. Li, L. Guo, L. Hai, and Y. Wu, Tetrahedron Lett., 2014, 55, 3938; CrossRef (b) A. Y. Simonov, E. E. Bykov, S. A. Lakatosh, Y. N. Luzikov, A. M. Korolev, M. I. Reznikova, and M. N. Preobrazhenskaya, Tetrahedron, 2014, 70, 625; CrossRef (c) T. Noro, K. Okano, and H. Tokuyama, Synlett, 2013, 24, 2143; CrossRef (d) G. He, C. Lu, Y. Zhao, W. Nack, and G. Chen, Org. Lett., 2012, 14, 2944. CrossRef
10. Theoretical calculations of the most stable structures of 7b and 7f-h were carried out using density functional theory (DFT) calculation at the B3LYP/6-31G** level. Compound 7f was less stable by approximately 38 kJ/mol than 7b, 7g, and 7h.
11. The B3LYP/6-31G** DFT calculations were performed with Spartan'14, Wavefunction, Irvine, CA.
12. For recent reports: (a) S. Zhong, M. Nieger, A. Bihlmeier, M. Shi, and S. Braese, Org. Biomol. Chem., 2014, 12, 3265; CrossRef (b) P. N. Naik, A. Khan, and R. S. Kusurkar, Tetrahedron, 2013, 69, 10733; CrossRef (c) W. E. Noland, C. L. Etienne, and N. P. Lanzatella, J. Heterocycl. Chem., 2011, 48, 381; CrossRef (d) C. Gioia, A. Hauville, L. Bernardi, F. Fini, and A. Ricci, Angew. Chem. Int. Ed., 2008, 47, 9236; CrossRef (e) C. A. Faler and M. M. Joullie, Org. Lett., 2007, 9, 1987; CrossRef (f) H. Lebel, M. Davi, S. Diez-Gonzalez, and S. P. Nolan, J. Org. Chem., 2007, 72, 144; CrossRef (g) J. Waser, B. Gaspar, H. Nambu, and E. M. Carreira, J. Am. Chem. Soc., 2006, 128, 11693; CrossRef (h) R. S. Kusurkar and S. K. Goswami, Tetrahedron, 2004, 60, 5315; CrossRef (i) C.-G. Yang, J. Wang, X.-X. Tang, and B. Jiang, Tetrahedron: Asymmetry, 2002, 13, 383. CrossRef
13. For recent reports: (a) S. E. S. Martin and D. A. Watson, J. Am. Chem. Soc., 2013, 135, 13330; CrossRef (b) M. A. Savolainen and J. Wu, Org. Lett., 2013, 15, 3802; CrossRef (c) H. Egami, R. Shimizu, and M. Sodeoka, Tetrahedron Lett., 2012, 53, 5503; CrossRef (d) M. Terada, K. Moriya, K. Kanomata, and K. Sorimachi, Angew. Chem. Int. Ed., 2011, 50, 12586; CrossRef (e) K. Murai, M. Morishita, R. Nakatani, O. Kubo, H. Fujioka, and Y. Kita, J. Org. Chem., 2007, 72, 8947; CrossRef (f) W.-P. Deng, G. Nam, J. Fan, and K. L. Kirk, J. Org. Chem., 2003, 68, 2798; CrossRef (g) C.-G. Yang, J. Wang, X.-X. Tang, and B. Jiang, Tetrahedron: Asymmetry, 2002, 13, 383. CrossRef
14. For recent reports: (a) M. J. Koh, R. K. M. Khan, S. Torker, and A. H. Hoveyda, Angew. Chem. Int. Ed., 2014, 53, 1968; CrossRef (b) Y. Shen, G. Liu, Z. Zhou, and X. Lu, Org. Lett., 2013, 15, 3366; CrossRef (c) M. A. Schafroth, D. Sarlah, S. Krautwald, and E. M. Carreira, J. Am. Chem. Soc., 2012, 134, 20276; CrossRef (d) E. M. Ferreira and B. M. Stoltz, Tetrahedron Lett., 2006, 47, 8579. CrossRef
15. For recent reports: (a) P. N. Naik, A. Khan, and R. S. Kusurkar, Tetrahedron, 2013, 69, 10733; CrossRef (b) Z. Mao, A. Lin, Y. Shi, H. Mao, W. Li, Y. Cheng, and C. Zhu, J. Org. Chem., 2013, 78, 10233; CrossRef (c) D. Enders, C. Joie, and K. Deckers, Chem. Eur. J., 2013, 19, 10818; CrossRef (d) D. G. Hingane, S. K. Goswami, V. Puranik, and R. S. Kusurkar, Synth. Commun., 2012, 42, 1786; CrossRef (e) B. Tan, G. Hernandez-Torres, and C. F. Barbas, J. Am. Chem. Soc., 2011, 133, 12354; CrossRef (f) C. Gioia, L. Bernardi, and A. Ricci, Synthesis, 2010, 161; (g) R. S. Kusurkar and S. K. Goswami, Tetrahedron 2004, 60, 5315; CrossRef (h) P. A. Grieco and M. D. Kaufman, J. Org. Chem., 1999, 64, 7586. CrossRef
16. (a) H.-H. Zhang, X.-X. Sun, J. Liang, Y.-M. Wang, C.-C. Zhao, and F. Shi, Org. Biomol. Chem., 2014, 12, 9539; CrossRef (b) G. Bergonzini, L. Gramigna, A. Mazzanti, M. Fochi, L. Bernardi, and A. Ricci, Chem. Commun., 2010, 46, 327. CrossRef
17. For recent reports: (a) C. A. Faler and M. M. Joullie, Org. Lett., 2007, 9, 1987; CrossRef (b) L. R. Marcin, D. J. Denhart, and R. J. Mattson, Org. Lett., 2005, 7, 2651. CrossRef
18. B. H. Lipshutz, C. Lindsley, and A. Bhandari, Tetrahedron Lett., 1994, 35, 4669. CrossRef