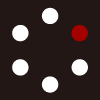
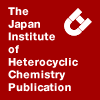
HETEROCYCLES
An International Journal for Reviews and Communications in Heterocyclic ChemistryWeb Edition ISSN: 1881-0942
Published online by The Japan Institute of Heterocyclic Chemistry
e-Journal
Full Text HTML
Received, 30th March, 2015, Accepted, 26th May, 2015, Published online, 4th June, 2015.
DOI: 10.3987/COM-15-13216
■ Structure and Absolute Configuration of the 11-Noriridoid “Chapingolide”
Matilde Villa-García, María Amparo Borja-De-La-Rosa, Holber Zuleta-Prada, Rubén A. Toscano, and Benito Reyes-Trejo*
Laboratorio de Productos Naturales, Área de Química, Departamento de Preparatoria Agrícola, Universidad Autónoma Chapingo, Apartado Postal 74, Oficina de Correos Chapingo, Km 38.5 Carretera México-Texcoco, Chapingo, México 56230, México
Abstract
The natural product chapingolide {systematic name: (4aS, 7R, 7aR)-7-hydroxy-7-(hydroxymethyl)-4,4a,7,7a-tetrahydrocyclopenta[c]pyran-3(1H)-one}, C9H12O4, (1), is a new 11-nor-iridoid isolated from Calatola mollis Standl. The colourless compound has a bicyclic structure with an endo double bond in the five-membered ring. The absolute configuration was established by mean of the anomalous dispersion of the oxygen scatters present in the structure.Calatola is a genus of trees in family Icacinaceae. To date, about seven Calatola species are recorded in America, and most of them are found in rain forest regions.1 Approximately, four species of Calatola plants were recorded in Mexico1 of which, Calatola mollis Standl is a tree up to 20 m tall. The seeds exhibited potently vomitive-purgative activity.2 At present Calatola mollis species had been poorly investigated with only botanical descriptions reported to date.1 Even thought, specimens of this species have been misidentified as Calatola costaricensis,1,3 and hence a phytochemical study of plants of Calatola genus will be useful to establish differences in secondary metabolites composition as a valuable systematic character.
The chapingolide (1), a nor-iridoid, was obtained from the methanolic extract of the leaves of Calatola mollis Standl, as a crystalline colourless compound. Its bicyclic structure is closely related to the structures discussed in Valladares and Rios,4 and Franzyk and Stermitz.5 The main differences reside in the oxidation level in the nor-iridoid skeleton compared with the previous structures, as well as an endo double bond in 1.6,7 In order to ascertain unambiguously the relative position of the hydroxyl groups and the double bond in the structure, as well as to establish the absolute configurations of the asymmetric centres, we analyze herein the thus far unreported crystal structure of chapingolide, isolated from Calatola mollis Standl. Icacinaceae leaves.
In order to assess the relative frequency of nor-iridoids, a search of the Cambridge Structural Database (CSD, Version 5.34)8 was undertaken. Surprisingly few relevant structures were found. There were only eleven structures with a similar 11-noriridoid system (cis-2-oxabicyclo[4.3.0]nonane) and, similarly, only three structures with cis-2-oxabicyclo[4.3.0]nonan-3-one system and fused five membered rings with substitution equivalent to 1.4,5,9 The bond lengths and angles found for 1 (Table 1) closely match the comparable parameters in these literature structures. In order to complete the full characterization of chapingolide and to elucidate its absolute configuration, its crystal structure has been determined.
Figure 1a shows an ellipsoid plot of chapingolide. The molecule is made up of a cyclopentene ring fused to a six-membered lactone ring fused showing, a cis A/B ring junction, C6=C7 double bond and a syn orientation among H-5, H-9 and CH2-10. In accordance with the biosynthetic origin of the iridoids10 the cis A/B ring junction is, and 1 corresponds to 8R,10-dihydroxy-cis-2-oxabicyclo[4.3.0]nonan- 6-en-3-one.
The Cremer & Pople parameters11 for each ring are as follows: For pyran ring, Q = 0.672(2) Å, = 90.53(17) °, and = 234.26(18) °, and for cyclopentene ring, Q = 0.090(2) Å, = 133.4(15) °, indicating “intermediate conformations”.
In order to assess in a more quantitative way the real significance of the verbal description for “intermediate conformations”, a quantitative conformational analysis was performed by using the program RICON12 showing that the pyran ring displays an “intermediate conformation” between boat (80%) and twist (19%) conformation, while the cyclopentene ring poses an “intermediate conformation” between twist (61%) and envelope (39%) conformations. The dihedral angle between cyclopentene and pyran rings is 81.99(12)°.
As the compound consists only of C, H and O atoms, to establish the absolute configuration of 1 we subject the crystals to a crystallographic analysis by the anomalous X-ray scattering from the oxygen atoms13 using Cu K radiation and by carefully measuring a large number of Bijvoet differences (812 Friedel pairs, 99%).
This yielded a refined Flack x parameter14 and standard uncertainty (s.u.) of 0.18 (25) for the “hole-in-one” fit. We note that the obtained s.u. value is above the suggested upper confidence limit of 0.10 for the determination of the absolute structure of an enantiopure compound.13 However, additional confirmation was obtained from the examination of Bayesian statistics on 812 Bijvoet pairs15 carried out using the program PLATON16 and suggested that the chapingolide structure is enantiopure: the probability P2 (true) = P3 (true) = 1.000 with P3(racemate-twin) = 0.9 x 10-12, P3 (false) = 0.2 x 10-59, G = 0.80(10), and the Hooft parameter y = 0.10 (5) for the C8 R enantiomer (5S, 9R). The SHELXL program17 was used to refine the structure and it reported a Flack parameter of 0.176(252), a G parameter of 1.03(12) and a Hooft parameter of 0.090(49) (Parsons’ method).18
Regarding the supramolecular structure of (1), there is one significant intermolecular interaction, involving the hydroxyl O3—H3 group as donor and carbonylic atom O1 as acceptor (Table 2); connecting neighbouring molecules into a C(8) chain structure19 running along b and built up around the twofold screw axis. These [010] chains are connecting by weak O3—H3O1 and C4—H4CO1 interactions. This is observable in Figure 1b and quantitatively assessed by the rather low packing index of 72.7. 20 In addition, hydroxyl O3—H3 displays an intramolecular hydrogen bond with the hydroxyl oxygen atom O4, making a 3-center (bifurcate) hydrogen bond (sum of 3 angles about H3 = 359(4) °).
EXPERIMENTAL
Optical Rotation was measured on a Perkin-Elmer 343 polarimeter. IR spectra were determined with an Agilent Cary FTIR-ATR 630 spectrophotometer. The 13C and 1H NMR spectra were recorded on an Agilent 400 MR DD2 spectrometer (SantaClara, CA, USA) operating at 100 MHz for 13C and 400 MHz for 1H. The 13C and 1H chemical shifts were measured in CDCl3 relative to TMS as an internal standard. HRFAB MS spectrum was measured on a JEOL the MStation JMS-700.
Isolation
Methanol extract (30 g) from the leaves of C. mollis (2 kg) was chromatographed over silica gel (200 g) starting with CH2Cl2 and increasing the polarity with MeOH. Fractions eluted with CH2Cl2/MeOH (95:5) provided 50 mg of a crystalline colourless solid which was submitted to X-ray diffraction study and identified as chapingolide (1). For related structures, see: Ban et al. (2013); Tada et al. (1998).6,7
Chapingolide (1): colourless solid; mp 129-131 °C (CH2Cl2/MeOH (95:5)); αD20 +89.4 (c 0.17, MeOH); IR (neat) 3439, 1711, 1014 cm-1; 1H NMR 2.56 (dd, J = 14, 4 Hz, 1H), 2.68 (dt, J = 9, 4 Hz, 1H), 2.74 (dd, J = 14, 7 Hz, 1H), 3.35 (m, 1H), 3.50 (d, J = 11 Hz, 1H), 3.61 (d, J = 11 Hz, 1H), 4.28 (dd, J = 14, 4 Hz, 1H), 4.57 (dd, J = 14, 3.5 Hz, 1H), 5.82 (br d, J = 6 Hz, 1H), 5.85 (br d, J = 6 Hz, 1H); 13C NMR 34.35, 40.56, 41.26, 66.87, 69.27, 86.32, 135.51, 136.00, 172.29; HRFAB MS m/z 185.08131 (M+H)+ Anal. Calcd for C9H13O4.
X-RAY STRUCTURE DETERMINATION OF 1
A colorless prism crystal of C9H12O4 having approximate dimensions of 0.41 × 0.17 × 0.04 mm was mounted on a glass fiber. All measurements were made on a Bruker Venture -diffractometer using Cu-K radiation monochromated by multilayer mirror. Cell constants and an orientation matrix for data collection corresponded to a primitive monoclinic cell with dimensions: a = 5.1034(7) Å, b = 10.8665(15) Å, c = 7.6481(10) Å, = 92.595(6) °, V = 423.7(1) Å3. The crystal belongs to the space group P21. Of the 17267 reflections that were collected, 1729 were unique (Rint = 0.030); equivalent reflections were merged. Data were collected and processed using APEX2 (Bruker). The structure was solved by direct methods and expanded using Fourier techniques. Deposition number CCDC-1056178 for compound No. 1. Free copies of the data can be obtained via http://www.ccdc.cam.ac.uk/conts/retrieving.html (or from the Cambridge Crystallographic Data Centre, 12 Union Road, Cambridge, CB2 1EZ, UK; Fax: +44 1223 336033; e-mail: deposit@ccdc.cam.ac.uk).
ACKNOWLEDGEMENTS
We are grateful to Dr. Enrique Guizar Nolazco for collecting the plant material and Dr. Patricia Vera Calleti, Área de Biología, Departamento de Preparatoria Agrícola, Universidad Autónoma Chapingo, for the identification of the plant material and CONACyT (México) for stimulating support.
References
1. P. Vera-Caletti and T. Wendt, Acta Botánica Mexicana, 2001, 54, 39.
2. M. Martínez, ‘Las Plantas Medicinales de México’, 4th edition, Ediciones Botas, México D.F., México, 1959.
3. The Red List of Mexican Cloud Forest Trees, ICACINACEAE, ed. by M. González-Espinosa, J. A. Meave, G. G. Lorea-Hernández, G. Ibarra-Manríquez, and A. C. Newton, Fauna & Flora International, Cambridge, UK, 2011, p. 38.
4. M. G. Valladares and M. Y. Ríos, J. Nat. Prod., 2007, 70, 100. CrossRef
5. H. Franzyk and F. R. Stermitz, J. Nat. Prod., 1999, 62, 1646. CrossRef
6. N. K. Ban, V. H. Giang, T. M. Linh, L. Q. Lien, N. T. Ngoc, N. H. Nam, N. X. Cuong, P. V. Kiem, C. V. Minh, and D. T. Do Thi Thao, Phytochem. Lett., 2013, 6, 267. CrossRef
7. M. Tada, S. Inoue, T. Miki, S. Onogi, J. Kaminaga, J. Hiraoka, K. Kitano, and K. Chiba, Chem. Pharm. Bull., 1998, 46, 1451. CrossRef
8. F. H. Allen, Acta Cryst., 2002, B58, 380. CrossRef
9. J.-G. Huang, L.-J. Zhou, and H.-H. Xu, Helv. Chim. Acta, 2011, 94, 327. CrossRef
10. V. Plouvier and J. Favre-Bonvin, Phytochemistry, 1971, 10, 1697. CrossRef
11. D. Cremer and J. A. Pople, J. Am. Chem. Soc., 1975, 97, 1354. CrossRef
12. A. Y. Zotov, V. A. Palyulin, and N. S. Zefirov, J. Chem. Inf. Comput. Sci., 1997, 37, 766. CrossRef
13. H. D. Flack and G. Bernardinelli, J. Appl. Cryst., 2000, 33, 1143. CrossRef
14. H. D. Flack, Acta Cryst., 1983, A39, 876. CrossRef
15. R. W. W. Hooft, L. H. Straver, and A. L. Spek, J. Appl. Cryst., 2008, 41, 96. CrossRef
16. A. L. Spek, Acta Cryst., 2009, D65, 148.
17. G. M. Sheldrick, Acta Cryst., 2008, A64, 112. CrossRef
18. S. Parsons, H. D. Flack, and T. Wagner, Acta Cryst., 2013, B69, 249.
19. J. Bernstein, R. E. Davis, L. Shimoni, and N.-L. Chang, Angew. Chem., Int. Ed. Engl., 1995, 34, 1555. CrossRef
20. A. I. Kitaigorodsky, ‘Molecular Crystals and Molecules’, Academic Press, London, 1973.