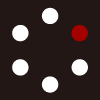
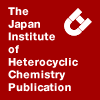
HETEROCYCLES
An International Journal for Reviews and Communications in Heterocyclic ChemistryWeb Edition ISSN: 1881-0942
Published online by The Japan Institute of Heterocyclic Chemistry
e-Journal
Full Text HTML
Received, 14th April, 2015, Accepted, 7th May, 2015, Published online, 15th May, 2015.
DOI: 10.3987/COM-15-13227
■ Preparation of Acylthiophenes by Iron(III) Chloride Catalyzed Reactions of Tris(2-thienyl)stibanes with Acyl Chlorides
Naoki Kakusawa,* Yoshie Nakagawa, Yutarou Toshima, Shuji Yasuike, and Jyoji Kurita
Faculty of Pharmaceutical Sciences, Hokuriku University, 3-Ho, Kanagawa machi, Kanazawa 920-1181, Japan
Abstract
The reactions of tris(2-thienyl)stibanes with various acyl chlorides, using a catalytic amount of iron(III) chloride, afforded 2-acylthiophenes. Iron(III) chloride is presumed to act as a Lewis acid, and the ipso substituent of each 2-thienyl group of tris(2-thienyl)stibane is replaced with an acyl group. The reaction is highly atom-efficient in that all three thiophene rings of tris(2-thienyl)stibane take part in the reaction. The reaction procedure is so simple that it can also be carried out under solvent-free and aerobic conditions.The acylthiophene moiety is an important building block for the synthesis of functional organic materials,1 pharmaceuticals, 2 and biologically active compounds.3 Much attention has been paid given to the development of general and efficient methods for the synthesis of acylthiophenes. Typical methods involve the Lewis acid mediated Friedel–-Crafts acylation of thiophene,4 transition- metal- catalyzed cross-coupling reactions,5 carbonylative coupling reactions,6 oxidative arylation of thiophene-2- aldehyde, 7 and oxidation of thiophene alcohol1-(2-thienyl)ethanols with mild oxidants. 8 These methods for the functionalization of electron- rich five- membered heteroaromatic compounds often have some disadvantages. For example, the conventional Friedel–-Crafts acylation suffers from use the requirement of a stoichiometric amount of a moisture- sensitive Lewis acid, low regioselectivity, and poor compatibility of with functional groups. With respect to the cross-coupling reactions, expensive and toxic transition metals are needed as catalysts, and elaboratecomplicatedd phosphine ligands tends to easily undergo air-oxidation in air. Carbonylative couplings reactions are often conducted under high pressures of carbon monoxide. Therefore, a simple and atom-efficient reaction for the preparation of acylthiophenes, using under an air- and moisture-stable catalyst with a reasonable price, and high atom-efficiency is still desired.
As a part of our studies on organoantimony compounds,9 we have investigated the reaction of tris(2-thienyl)stibane derivativess (1) 10 with acyl chlorides (2), under using a catalytic amount of iron (III) chloride, to find that acylthiophenes were formed in high yields. One of the aAdvantages of the use of tris(2-thienyl)stibanes (1) is are mild reaction conditions and high atom efficienciesy, because ofresulting from the high reactivity of organoantimony compounds. In addition, iron catalyst is an attractive substitute for transition- metal catalysts because of its high abundance, reasonable cost, and low toxicity, and environmentally benign nature. Iron-catalyzed reactions has have been the focus of attention and many reports have recently been published recently.11 We now report an operationally simple and atom-efficient procedure for the synthesis of acylthiophenes using from tris(2-thienyl)stibanes and acyl chlorides, under using an iron (III) chloride catalyst.
Optimization experiments were conducted using 1a and benzoyl chloride (2a) as model substrates, and the results are summarized in Table 1. A mixture of 1a and 2a was heated at 80 °C without a transition-metal catalyst. Although the reaction did not proceed at all (even after prolonged heating) in the solvent 1-methyl-2-pyrrolidone (NMP), the slow formation of 2-benzoylthiophene (3aa) was observed in benzene and 1,2-dichloroethane (1,2-DCE). The reaction can also be conducted under solvent-free conditions to give 2-benzoylthiophene (3aa) (Table 1, entries 1–4).
In order to accelerate the reaction, sSeveral kinds types of catalysts were added to the reaction mixture to accelerate the reaction. With palladium catalysts, quick consumption of 1a was observed on by TLC;, however, the yield of 3aa was moderate (entries 5-–7). The aAddition of p-toluenesulfphonic acid as a protic acid catalyst did not shorten the reaction time, nor did it increase the yield (entry 8). Screening of several Lewis acid catalysts revealed that the reaction completed in a short time with a catalytic amount of boron trifluoride etherate and aluminum chloride (entries 9 and 10). Titanium tetrachloride, zinc chloride, and ytterbium (III) trifluoromethanesulfphonate were not effective in reducing the reaction time (entries 119–-13). Further examination revealed that a catalytic amount (10 mol%) of iron (III) chloride promoted the reaction to give the 3aa in 96% yield within 1 h in the solvent 1,2-DCE as solvent (entry 14). The yield of 3aa was calculated based on 2a, suggesting that all the three thiophene rings on of 1a took take part in the reaction. We employed this condition as thethese optimized one conditions in the following reaction due tobecause of its atom -efficiency. BesidesMoreover, the iron (III) chloride catalyzed reaction was revealed shown to proceed under solvent- free -conditions. When iron (III) chloride was added directly, under aerobic conditions, to the mixture of 1a and 2a, an exothermic reaction occurred and the formation of 3aa was observed instantly (entry 15).
With
Having determined the optimized conditions in hands,12 we next investigated the reaction of 1a with various acyl chlorides (2a–-l). In the case of aromatic acyl chlorides, electron- donating substituents such as methyl and methoxy groups on benzene the aromatic ring facilitated the reaction to afford the dbiaryl ketones in good yields in a short time (Table 2, entries 2 and, 3). Electron- rich 2-thiophenecarboxylic acid chloride (2d) is was also found to be a good substrate (entry 4). On the contrary, benzoyl chlorides with electron- withdrawing substituents exhibited lower reactivitiesy. The reaction of 1a with p-cyano-, p-nitro-, and p-trifluoromethyl-subustituted benzoyl chlorides (2e–-g) required prolonged reaction times and the yields of the products were moderate (entries 5–-7). Aliphatic acyl chlorides are were also found to be good substrates. The reaction of 1a with acid chlorides with primary secondary and secondary tertiary α-carbons gave the alkyl 2-thienyl ketones in good yields within 1 h (entries 8–-11). However, 2,2-dimethyl propanoyl chloride (2l), which bear has a tertiary quaternary carbon in the at α-position, gave an inferior result (entry 12). Aliphatic acyl chlorides are generally poorer substrates than aromatic acyl chlorides in palladium- catalyzed cross-coupling reactions. 13
For the preparation of alkyl 2-thienyl ketones, our iron-catalyzed reaction is superior to conventional palladium- catalyzed cross-coupling reactions.
Iron(III) chloride catalyzed reactions of substituted tris(2-thienyl)stibanes (1b–d) with benzoyl chloride (2a) were also attempted (Scheme 1). 5-Sbstituted tris(2-thienyl)stibanes (1b,c) underwent ipso acylation exclusively to give 3ba and 3ca, respectively, while the reaction of tris(3-methyl-2-thienyl)stibane (1d) with benzoyl chloride (2a) gave a mixture of ipso-benzoylated 3da and 2-benzoyl-3-methylthiophene 3′da. Benzoylation of tris(3-methyl-2-thienyl)stibane (1d) occurred at the less hindered α-position of the thiophene ring, because the ipso position is sterically hindered by the adjacent methyl substituent
In conclusion, we have found that iron (III) catalyzed reactions of tris(2-thienyl)stibane with various acyl chlorides give ipso-substituted acyl thiophenes in good yields. The reaction can also be conducted under solvent-free conditions. Further investigations ofn this reaction, including the reaction mechanism,14 and of the benzoyl migration, are in progress.
ACKNOWLEDGEMENTS
We acknowledge financial support from the Special Research Found from of Hokuriku University and the Institute of Pharmaceutical Life Science, Aichi Gakuin University.
References
1. I. F. Perepichka and D. F. Peripichka, ‘Handbook of Thiophene-Based Materials’, John Wiley & Sons, Ltd., Chichester, 2009. CrossRef
2. Y. Mori, M. Shibata, K. Toyoshi, S. Baba, M. Horie, Y. Oshika, and K. Ohira, Radioisotopes, 1981, 30, 584. CrossRef
3. A. N. Christ, L. Labzin, G. T. Bourne, H. Fukunishi, J. E. Weber, J. M. Sweet, M. L. Smythe, and J. U. Flanagan, J. Med. Chem., 2010, 53, 5536; CrossRef S. Ozaki, E. Ebisu, K. Hamada, J.-I. Goto, A. Z. Suzuki, A. Terauchi, and K. Mikoshiba, Bioorg. Med. Chem. Lett., 2010, 20, 1141. CrossRef
4. J. R. Johnson and G. E. May, Org. Synth., 1943, Coll. Vol. 2, 8; K. P. Boroujeni, Chin. Chem. Lett., 2010, 21, 1395.
5. D. Ogawa, K. Hyodo, M. Suetsugu, J. Li, Y. Inoue, M. Fujisawa, M. Iwasaki, K. Takagi, and Y. Nishihara, Tetrahedron, 2013, 69, 2565; CrossRef D. Ogawa, K. Hyodo, M. Suetsugu, J. Li, Y. Inoue, M. Fujisawa, M. Iwasaki, K. Takagi, and Y. Nishihara, Tetrahedron, 2013, 69, 2565; CrossRef L. J. Gooßen and K. Ghosh, Angew. Chem. Int. Ed., 2001, 40, 3458. CrossRef
6. M. V. Khedkar, P. J. Tambade, Z. S. Qureshi, and B. M. Bhanage, Eur. J. Org. Chem., 2010, 6981; CrossRef P. H. Lee, S. W. Lee, and K. Lee, Org. Lett., 2003, 5, 1103. CrossRef
7. N. Kakusawa, S. Yasuike, and J. Kurita, Heterocycles, 2010, 80, 163.
8. S. Kodama, S. Hashidate, A. Nomoto, S. Yano, M. Ueshima, and A. Ogawa, Chem. Lett., 2011, 40, 495. CrossRef
9. N. Kakusawa, S. Yasuike, and J. Kurita, Heterocycles, 2010, 80, 163. CrossRef
10. H. Mahalakshmi, V. K. Jain, and E. R. T. Tiekink, Polyhedron, 2003, 22, 549; CrossRef H. Mahalakshmi, V. K. Jain, and E. R. T. Tiekink, Polyhedron, 2003, 22, 549. CrossRef
11. R. B. Bedford, P. B. Brenner, E. Carter, J. Clifton, P. M. Cogswell, N. J. Gower, M. F. Haddow, J. N. Harvey, J. A. Kehl, D. M. Murphy, E. C. Neeve, M. L. Neidig, J. Nunn, B. E. R. Snyder, and J. Taylor, Organometallics, 2014, 33, 5767. CrossRef
12. Experimental procedure for 3aa: A mixture of tris(2-thienyl)stibane (370 mg, 1.00 mmol), benzoyl chloride (422 mg, 3.00 mmol), and FeCl3 (16.2 mg, 0.10 mmol) in 1,2-dichloroethane (DCE)(3 mL) was stirred for 1 h at 80 °C under an argon atmosphere. The solvent was removed in vacuo and the residue was purified by silica gel column chromatography using hexane/EtOAc (10:1) as eluent to afford 541 mg (96% yield) of 3aa as a yellow oil. Recrystallization from hexane/Et2O gave yellow needle crystals: mp 56–57 °C. LRMS (EI): m/z 188 (M+). 1H NMR (400 MHz, CDCl3): δ 7.16 (1H, t, J = 4.35 Hz), 7.49 (2H, t, J = 7.79 Hz), 7.58 (2H, t, J = 7.79 Hz), 7.64 (1H, d, J = 4.35 Hz), 7.71 (1H, d, J = 4.35 Hz), 7.85 (1H, d, J = 7.79 Hz). 13C NMR (100 MHz, CDCl3): δ 127.9 (d), 128.4 (d), 129.2 (d), 132.3 (d), 134.2 (d), 134.8 (d), 138.1 (s), 143.6 (s), 188.2 (s). IR (KBr): ν 1635 cm-1 (C=O).
13. M. Haddach and J. R. McCarthy, Tetrahedron Lett., 1999, 40, 3109; CrossRef J. Ye, R. K. Bhatt, and J. R. Falck, J. Am. Chem. Soc., 1994, 116, 1. CrossRef
14. A plausible reaction mechanism: Slightly negatively charged carbon atom at ipso position of 1a attacked 2a, activated by FeCl3 catalyst, to form acyl-stibanothiophene adduct (4). Elimination of bis(2-thienyl)antimony(III) chloride from 4, accompanied by aromatization of thiophene, furnished 3aa. Two thiophene rings of bis(2-thienyl)antimony chloride undergo similar reaction sequence successively.