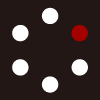
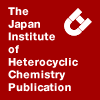
HETEROCYCLES
An International Journal for Reviews and Communications in Heterocyclic ChemistryWeb Edition ISSN: 1881-0942
Published online by The Japan Institute of Heterocyclic Chemistry
e-Journal
Full Text HTML
Received, 16th April, 2015, Accepted, 20th May, 2015, Published online, 26th May, 2015.
DOI: 10.3987/COM-15-13229
■ A New N-Glucosylated Indole Alkaloid and a New O-Serine Glycoside from Berberis koreana
Ki Hyun Kim, Hee Rae Kang, Eunjung Moon, Sang Un Choi, Ki Sung Kang, Sun Yeou Kim, and Kang Ro Lee*
Natural Products Laboratory, School of Pharmacy, Sungkyunkwan University, 300 Chunchun-dong, Jangan-ku, Suwon 440-746, Korea
Abstract
A new N-glucosylated indole alkaloid, 1-(1-β-glucopyranosyl)-3-(hydroxymethyl)-1H-indole (1) and a new O-serine glycoside, 3-O-α-D-xylopyranosyl-L-serine methyl ester (2), along with five known compounds (3-7) were isolated from the trunk of Berberis koreana. The structures of the new compounds were elucidated by 1D and 2D NMR data analysis and chemical reaction. Compounds 1, 2, 4, 5, and 7 reduced nitric oxide (NO) production in lipopolysaccharide (LPS)-activated BV-2 cells, a microglial cell line. In addition, compounds 4 and 5 showed moderate anti-proliferative activity against A549 and HCT-15 cell lines.Berberis koreana Palib. (Berberidaceae), commonly known as ‘Korean barberry’, is an endemic species distributed throughout northern Korea. B. koreana has been used in Korean traditional medicine for the treatment of various disorders such as fever, gastroenteritis, sore throats, conjunctivitis, dysentery, jaundice, pneumonia, indigestion, and stomach aches.1 Various alkaloids with medicinal properties have been reported from the genus Berberis,2 and previous phytochemical investigations on B. koreana demonstrated the presence of alkaloids, including benzylisoquinoline and protoberberine derivatives as well as pyrrole acids.3-6 In our preliminary study, our group found that a MeOH extract of the trunk of B. koreana exhibited significant cytotoxicity against some human tumor cell lines, which has led us to investigate the MeOH extract.7-12 In the systematic study of B. koreana for its bioactive constituents, our investigation of B. koreana has resulted in the isolation and identification of many bioactive compounds, such as biphenyls, lignans, triterpenoids, sesquiterpene, steroids, and phenolics with cytotoxic and anti-inflammatory activities.7-12 In the process of our continuing efforts to study this source, a new N-glucosylated indole alkaloid (1) and O-serine glycoside (2), along with five known compounds (3-7) (Figure 1) were isolated using a bioassay guided fractionation technique. The structures of 1 and 2 were determined by spectroscopic data interpretation, particularly by extensive 1D and 2D NMR experiments and chemical reaction. All compounds were tested for their anti-inflammatory effects in lipopolysaccharide (LPS)-stimulated murine microglia BV2 cells and their anti-proliferative activities against four human tumor cell lines (A549, SK-OV-3, SK-MEL-2, and HCT-15). We report herein the isolation and structural elucidation of compounds 1 and 2, and the biological effects of the isolates.
Compound 1 was isolated as a yellowish gum with a positive Dragendorff reaction on TLC. The molecular formula was established as C15H19NO6 based on the [M + Na]+ peak at m/z 332.1117 (calcd. for C15H19NO6Na, 332.1110) in the HR-ESIMS. In compliance with the molecular formula, the presence of hydroxyl groups in the molecule could be proposed from the IR absorption band of 1 at 3395 cm-1. The 1H NMR (Table 1) and 1H-1H COSY spectra displayed a spin coupling system of four aromatic proton signals at δH 7.65 (d, J = 7.5 Hz), 7.48 (d, J = 8.0 Hz), 7.13 (dd, J = 8.0, 7.5 Hz), and 7.05 (t, J = 7.5 Hz), indicating an ortho-substituted aromatic ring, and a separated aromatic proton signal at δH 7.29 (s), which were typical of a 3-substituted indole moiety. The substituent at C-3 was determined as a hydroxymethyl group, supported by the signals at δH 4.81 (2H, s, H-8) and δC 63.4 (C-8), together with HMBC correlations of H-8/C-2, H-8/C-3, and H-8/C-3a (Figure 2). Detailed analysis of NMR spectral data of 1 led to assign the skeleton of indole-3-methanol (Figure 2).13 It also revealed the presence of a glucopyranoside, which was supported by the anomeric proton signal at δH 5.41 (d, J = 8.5 Hz), other remaining proton signals at δH 3.94-3.52 (6H), and the carbon signals at δC 85.2, 79.0, 77.4, 72.1, 70.1, and 61.3.14 The large coupling constant (8.5 Hz) of the anomeric proton indicated a β-configuration of the glucose, and the anomeric proton exhibited HMBC correlations with C-2 and C-7a, indicating an N-glucoside. Thus, the structure of 1 was established as 1-(1-β-glucopyranosyl)-3-(hydroxymethyl)-1H-indole. To our knowledge, four N-glucosyl-1H-indole derivatives were isolated from fruiting bodies of the basidiomycete Cortinarius brunneus (Pers.) Fr., recently.14 Nonetheless, the group of N-glucosyl-1H-indole derivatives has been rare in the natural products, and has rarely been found so far. Literature survey revealed that 3-carboxymethyl-indole-1-N-β-D-glucopyranoside and its methyl ester derivative were isolated from red currants (Ribes rubrum).15
Compound 2 was isolated as a colorless gum with a positive Dragendorff reaction on TLC. The molecular formula was established as C9H17NO7, which was deduced from the [M + Na]+ peak at m/z 274.0908 (calcd. for C9H17NO7Na, 274.0903) in the HR-ESIMS. The 1H and 13C NMR spectra of 2 (Table 1) displayed typical features of an amino acid at δH 4.35 (1H, dd, J = 15.0, 3.5 Hz), 4.06 (1H, dd, J = 15.0, 8.5 Hz), and 4.04 (1H, dd, J = 8.5, 3.5 Hz), and δC 172.6, 67.6, and 56.0, along with one methoxy signal at δH 3.68 (3H, s) and δC 50.8, indicating the presence of a serine methyl ester, which was confirmed by 1H-1H COSY, HMQC, and HMBC data (Figure 2). The remaining signals in the 1H and 13C NMR spectra of 2 at δH 4.76 (1H) and 3.86-3.63 (5H), and δC 99.8, 73.7, 71.8, 69.4, and 62.7 were assigned to an α-D-xylopyranose.16 The small coupling constant (3.5 Hz) of the anomeric proton at δH 4.76 indicated an α-configuration of the xylose, and the HMBC correlation of H-1/C-3 implied that the sugar group was linked to C-3. Acid hydrolysis of 2 was performed for 1 h to obtain aglycone (serine) and D-xylose. The D-xylose was confirmed by the sign of its specific rotation value ([α]D25+25.3, H2O). The advanced Marfey’s method using FDAA (1-fluoro-2,4-dinitrophenyl-5-alanine amide) was applied to determine the absolute configuration of the aglycone (serine).17 LC/MS analyses of the L- and D-FDAA derivatives allowed us to determine that the absolute configuration of α-carbon in the aglycone is S (L). Thus, the structure of 2 was assigned as 3-O-α-D-xylopyranosyl-L-serine methyl ester. Amino acid glycosides have been unusual in natural products, and only a few compounds have been reported up to date.18,19 To our knowledge, compound 2 represents the first example of a serine glycoside from a natural plant source.
The five known compounds were identified as 3,4,5-trimethoxyphenyl-β-D-apiofuranosyl-(1→6)-β-D-glucopyranoside (3),20 (-)-lyoniresinol (4),21 (+)-lyoniresinol (15),21 (-)-lyoniresinol 3α-O-β-D-glucopyranoside (6),21 and (+)-lyoniresinol 3α-O-β-D-glucopyranoside (7),21 by comparing their spectroscopic data with the reported data. The absolute configurations of the above known compounds were established on the basis of their 1H NMR coupling constant values, optical rotation values, and CD spectroscopic data. This is the first time that all of these known compounds (3-7) were isolated from B. koreana.
The isolated compounds 1-7 were evaluated by examining NO production in lipopolysaccharide (LPS)-activated BV-2 cells, a microglial cell line.22 NO is a gaseous signaling molecule which has pivotal roles in immune and inflammatory responses and neuronal transmission in the brain.23 Under normal conditions, NO has neuroprotective and antioxidative effects. However, overproduction of NO from activated microglia plays a role in various neurodegenerative diseases such as Alzheimer’s disease and Parkinson’s disease through mitochondrial dysfunction and neuronal cell death via the formation of an extremely potent oxidizing and neurotoxic agent, peroxynitrite (ONOO-).24 As shown in Table 2, compounds 1, 2, 4, 5, and 7 decreased NO levels in the medium with IC50 values in the range of 69.14-156.87 μM without affecting cell viability. Compound 5 strongly reduced NO levels with an IC50 value of 69.14 μM, while the new compounds 1 and 2 showed weak activity with IC50 values of 120.51 and 156.87 μM, respectively. Interestingly, glycosylation of (-)- and (+)-lyoniresinols apparently reduces the NO activity when compared with the tested activities among compounds 4-7 (Table 2). Furthermore, (+)-lyoniresinol derivatives (5 and 7) show better anti-inflammatory activity than (-)-lyoniresinol derivatives (4 and 6).
The anti-proliferative activities of the isolates (1-7) were also evaluated by determining their inhibitory effects on four human tumor cell lines, including A549 (non-small cell lung carcinoma), SK-OV-3 (ovary malignant ascites), SK-MEL-2 (skin melanoma), and HCT15 (colon adenocarcinoma) using a SRB assay.25 Compounds 4 and 5 exhibited moderate inhibitory activity against the proliferation of A549 and HCT-15 cell lines with IC50 values in the range of 22.13-7.14 μM (Table 3), while their glycosides, compounds 6 and 7, exhibited no cytotoxicity against all tested cell lines (IC50 >30.0 μM). Similarly, glycosylation of the lignans (compounds 4 and 5) leads to the loss of their anti-proliferative activity, and (+)-lyoniresinol (compound 5) display better cytotoxicity than (-)-lyoniresinol (compound 4). The other compounds (1-3) did not have inhibitory effects (IC50 >30.0 μM) against any of the cell lines.
In conclusion, we isolated and identified two unusual compounds, a new N-glucosylated indole alkaloid, 1-(1-β-glucopyranosyl)-3-(hydroxymethyl)-1H-indole (1) and a new O-serine glycoside, 3-O-α-D-xylopyranosyl-L-serine methyl ester (2), along with five known compounds (3-7) from the trunk of B. koreana. All the isolates were evaluated for their ability to reduce NO production in LPS-activated microglia BV-2 cells and for anti-proliferative activity against four human tumor cell lines (A549, SK-OV-3, SK-MEL-2, and HCT-15). The present study indicates that compounds 4 and 5 are good candidates for future studies, and should be further investigated as anti-inflammatory and antitumor agents.
EXPERIMENTAL
General. Optical rotations were measured by a Jasco P-1020 polarimeter (Jasco, Easton, MD, USA). IR spectra were recorded by a Bruker IFS-66/S FT-IR spectrometer (Bruker, Karlsruhe, Germany). Electrospray ionization (ESI) and HR-ESI mass spectra were recorded by a SI-2/LCQ DecaXP Liquid chromatography (LC)-mass spectrometer (Thermo Scientific, West Palm Beach, PL, USA). Nuclear magnetic resonance (NMR) spectra, including 1H-1H COSY, HMQC, and HMBC experiments, were recorded by a Varian UNITY INOVA 500 NMR spectrometer (Varian, Palo Alto, CA, USA) operating at 500 MHz (1H) and 125 MHz (13C), with chemical shifts given in ppm (δ) using tetramethylsilane (TMS) as an internal standard (0 ppm) for 1H and 13C NMR analysis. Preparative high performance liquid chromatography (HPLC) used a Gilson 306 pump (Gilson, Middleton, WI, USA) with a Shodex refractive index detector (Shodex, New York, NY, USA). Silica gel 60 (Merck, 70-230 mesh and 230-400 mesh) and RP-C18 silica gel (Merck, 40-63 μm) were used for column chromatography. Merck precoated Silica gel F254 plates and RP-18 F254s plates (Merck, Darmstadt, Germany) were used for TLC. Spots were detected on TLC under UV light or by heating after spraying with anisaldehyde-sulfuric acid.
Plant material. The trunk of B. koreana was collected on Jeju Island, Korea, in December, 2005. Samples of plant material were identified by one of the authors (K. R. Lee). A voucher specimen (SKKU 2005-10) has been deposited in the herbarium of the School of Pharmacy, Sungkyunkwan University, Suwon, Korea.
Extraction and isolation. The trunk of B. koreana (2.7 kg) was dried, chopped, and then extracted with 80% aqueous MeOH two times (2 × 4 hours) under reflux, and filtered. The filtrate was concentrated under vacuum to obtain a MeOH extract (220 g), which we suspended in distilled water (7.2 L) and then successively partitioned with n-hexane, CHCl3, and n-BuOH, yielding 8, 10, and 50 g of residue, respectively. Each fraction was evaluated for cytotoxicity against human cancer cell lines using a SRB assay. The n-hexane-soluble and CHCl3-soluble fractions showed significant cytotoxic activity against tested cancer cell lines, while the n-BuOH-soluble fraction showed weak cytotoxic activity. The phytochemical investigation of the n-hexane-soluble fraction was conducted in our previous study.7,11 The CHCl3-soluble fraction (10 g) was separated on a silica gel (230-400 mesh, 250 g) column chromatography (CC) using a solvent system of n-hexane-EtOAc (1:1) and CHCl3-MeOH (10:1, 5:1) to yield ten fractions (A - J). Fractions G and H were consolidated and the fraction (4.3 g) was subjected to further RP-C18 silica gel (230-400 mesh, 200 g) CC using a gradient solvent system of MeOH-H2O (1:1 - 4:1) to give six subfractions (fr. G1 – G6). Fraction G1 (1.5 g) was applied to a Sephadex LH-20 column using a solvent system of CH2Cl2-MeOH (1:1) to obtain seven fractions (fr. G11 – G17). Fraction G15 (100 mg) was purified by preparative reversed-phase HPLC with a solvent system of MeCN-H2O (1:4, flow rate; 2 mL/min) to afford compound 4 (25 mg, tR=12.0 min). Fraction G16 (250 mg) was purified by preparative normal-phase HPLC, using a solvent system of n-hexane-CHCl3-MeOH (9:6:2) to furnish compound 5 (5 mg, tR=16.3 min). The n-BuOH-soluble fraction (50 g) was separated by silica gel (230-400 mesh, 1500 g) column chromatography (CC) with CHCl3-MeOH-H2O (9:3:0.5) to give four fractions (B1 - B4). Fraction B2 (13.6 g) was subjected to RP-C18 silica gel (230-400 mesh, 500 g) CC with MeOH-H2O (1:1) to give three subfractions (B21 - B23). Fraction B22 (2.7 g) was further subject to Sephadex LH-20 column with 100% MeOH to give three subfractions (B221 - B223). Among these subfractions, fraction B221 (430 mg) was purified by semi-preparative reversed-phase HPLC (flow rate: 2 mL/min) using a solvent system of MeOH-H2O (2:3) to afford compounds 6 (8 mg, tR=15.5 min) and 7 (5 mg, tR=16.1 min). A portion of fraction B23 (4.5 g) was purified by semi-preparative reversed-phase HPLC system with a solvent system of MeOH-H2O (1:1) to yield compound 3 (85 mg, tR=16.8 min). Fraction B4 (21.6 g) was subjected to RP-C18 silica gel (230-400 mesh, 500 g) CC with MeOH-H2O (2:3) to give four subfractions (B41 - B44). Fraction 42 (4.2 g) was further applied to Sephadex LH-20 column with 90% MeOH-H2O to give six subfractions (B421 - B426). Then, fraction B4251 (140 mg) was obtained by RP-C18 silica gel (230-400 mesh, 500 g) CC with 35% MeOH-H2O from fraction 425 (1.4 g), and the fraction was further purified by semi-preparative reversed-phase HPLC system with 35% MeOH-H2O to afford compounds 1 (8 mg, tR=12.5 min) and 2 (6 mg, tR=13.9 min).
1-(1-β-Glucopyranosyl)-3-(hydroxymethyl)-1H-indole (1): Yellowish gum; [α]D25+20.2 (c 0.20, MeOH); UV (MeOH) λmax (log ε) 267 (3.2), 220 (3.8) nm; IR (KBr) νmax 3395, 2926, 2855, 1718, 1650, 1451, 1354, 1263, 1030 cm-1; 1H (500 MHz) and 13C (125 MHz) NMR data, see Table 1; ESIMS (positive-ion mode) m/z: 332 [M + Na]+. HR-ESIMS (positive-ion mode) m/z: 332.1117 [M + Na]+ (Calcd for C15H19NNaO6, 332.1110).
3-O-α-D-Xylopyranosyl-L-serine methyl ester (2): Colorless gum; [α]D25-15.9 (c 0.15, MeOH); IR (KBr) νmax 3355, 2921, 2855, 1650, 1591, 1364, 1223, 1031 cm-1; 1H (500 MHz) and 13C (125 MHz) NMR data, see Table 1; ESIMS (positive-ion mode) m/z: 274 [M + Na]+. HR-ESIMS (positive-ion mode) m/z: 274.0908 [M + Na]+ (Calcd for C9H17NNaO7, 274.0903).
Determination of the absolute configuration of α-carbon of serine in compound 2. Compound 2 (3 mg) was hydrolyzed in 0.5 mL of 6 N HCl at 115 °C for 1 h, and the reaction mixture was subsequently cooled in ice water for 3 min. The reaction solvent was evaporated in vacuo, and residual HCl was completely removed by the addition of 0.5 mL of water and removal of the solvent three times. The hydrolysate was suspended in water and successively partitioned with EtOAc to yield the EtOAc extract containing the aglycone, serine. The aqueous phase of the hydrolysate were subjected separately to column chromatography over silica gel eluted with MeCN-H2O (8:1) to yield D-xylose with positive specific rotation ([α]D25+25.3, H2O). The EtOAc extract containing serine was divided into two portions, which were transferred into an 8 mL vial. The portion was then dissolved in 100 μL of 1 N NaHCO3. Either 50 μL of 10 mg/mL L-FDAA (1-fluoro-2,4-dinitrophenyl-5-L-alanine amide) or 50 μL of D-FDAA in acetone was added to each of the two vials. The reaction mixtures were incubated at 80 °C for 3 min. A 50 μL aliquot of 2 N HCl was added to neutralize the reaction, and 300 μL of aqueous 50% MeCN was added to the vials. A 20 μL aliquot of each reaction mixture was analyzed by LC-MS using a gradient solvent system (20 to 60% MeCN containing 0.1% formic acid over 40 min, C18 reversed-phase column: 100 × 4.6 mm, detection: UV 340 nm). The L-FDAA derivative eluted before the D-FDAA derivative for serine in the hydrolysate. Thus, the absolute configuration of the aglycone (serine) in 2 was determined to be L.
Measurement of NO production in LPS-activated microglial BV-2 cells. BV-2 cells were maintained in Dulbecco’s modified Eagle’s medium (DMEM) supplemented with 5% fetal bovine serum (FBS) and 1% penicillin-streptomycin. BV-2 microglia cells were stimulated with 100 ng/mL of LPS in the presence or absence of samples for 24 h. Nitrite, a soluble oxidation product of NO, was measured in the culture media by the Griess reaction. The supernatant (50 μL) was harvested and mixed with an equal volume of Griess reagent (1% sulfanilamide, 0.1% N-1-napthylethylenediamine dihydrochloride in 5% phosphoric acid). After 10 min, the absorbance at 540 nm was measured using a microplate reader (Emax, Molecular Device, Sunnyvale, CA, U.S.A.). NG-monomethyl-L-arginine (L-NMMA, Sigma, St. Louis, MO, USA), a well-known nitric oxide synthase inhibitor, was tested as a positive control.
In vitro cytotoxicity test. A sulforhodamine B (SRB) bioassay was used to determine the cytotoxicity of each compound against four cultured human cancer cell lines.25 The assays were performed at the Korea Research Institute of Chemical Technology. The cell lines used were A549 (non-small cell lung adenocarcinoma), SK-OV-3 (ovarian cancer cells), SK-MEL-2 (skin melanoma), and HCT15 (colon adenocarcinoma). Etoposide was used as a positive control.
ACKNOWLEDGEMENTS
This research was supported by the Basic Science Research Program through the National Research Foundation of Korea (NRF) funded by the Ministry of Education, Science and Technology (2013R1A1A2A10005315).
References
1. D. K. Ahn, ‘Illustrated Book of Korean Medicinal Herbs’, Kyohaksa, Seoul, 2003.
2. P. L. Schiff, J. Nat. Prod., 1991, 54, 645. CrossRef
3. V. Hrochova and D. Kostalova, Ceskoslov. Farm., 1992, 41, 37.
4. V. Hrochova and D. Kostalova, Ceskoslov. Farm., 1987, 36, 457.
5. D. Kostalova, B. Brazdovicova, and Y. J. Hwang, Farm. Obzor, 1982, 51, 213.
6. D. Kostalova, V. Hrochova, V. Suchy, M. Budesinsky, and K. Ubik, Phytochemistry, 1992, 31, 3669. CrossRef
7. K. H. Kim, S. U. Choi, C. S. Kim, and K. R. Lee, Biosci. Biotechnol. Biochem., 2012, 76, 825. CrossRef
8. K. H. Kim, S. U. Choi, and K. R. Lee, Planta Med., 2012, 78, 86. CrossRef
9. K. H. Kim, E. Moon, S. U. Choi, S. Y. Kim, and K. R. Lee, Bioorg. Med. Chem. Lett., 2011, 21, 2270. CrossRef
10. K. H. Kim, S. U. Choi, and K. R. Lee, Bioorg. Med. Chem. Lett., 2010, 20, 1944. CrossRef
11. K. H. Kim, S. U. Choi, S. K. Ha, S. Y. Kim, and K. R. Lee, J. Nat. Prod., 2009, 72, 2061. CrossRef
12. K. H. Kim, H. K. Kim, and S. U. Choi, Bull. Korean Chem. Soc., 2014, 35, 3346. CrossRef
13. A. Ros Barceló, M. A. Pedreño, M. A. Ferrer, F. Sabater, and R. Muñoz, Planta, 1990, 181, 448. CrossRef
14. A. Teichert, J. Schmidt, A. Porzel, N. Arnold, and L. Wessjohann, Chem. Biodivers., 2008, 5, 664. CrossRef
15. B. Schwarz and T. Hofmann, J. Agric. Food Chem., 2007, 55, 1405. CrossRef
16. K. Dill, R. E. Hardy, J. M. Lacombe, and A. A. Pavia, Carbohydr. Res., 1982, 101, 330. CrossRef
17. K. Fujii, Y. Ikai, H. Oka, M. Suzuki, and K. Harada, Anal. Chem., 1997, 69, 5146. CrossRef
18. M. Mutsch-Eckner, C. A. J. Erdelmeier, O. Sticher, and H. D. Reuter, J. Nat. Prod., 1993, 56, 864. CrossRef
19. Y. Ogawa and T. Konishi, Chem. Pharm. Bull., 2009, 57, 1110. CrossRef
20. H. I. Duynstee, M. C. Koning, G. A. Marel, and J. H. Boom, Tetrahedron, 1999, 55, 9881. CrossRef
21. K. Ohashi, H. Watanabe, Y. Okumura, T. Uji, and I. Kitagawa, Chem. Pharm. Bull., 1994, 42, 1924. CrossRef
22. D. W. Reif and S. A. McCreedy, Arch. Biochem. Biophys., 1995, 320, 170. CrossRef
23. V. Calabrese, C. Mancuso, M. Calvani, E. Rizzarelli, D. A. Butterfield, and A. M. Stella, Nat. Rev. Neurosci., 2007, 8, 766. CrossRef
24. J. Olesen, Neurotherapeutics, 2010, 7, 183. CrossRef
25. P. Skehan, R. Storeng, D. Scudiero, A. Monks, J. MaMahon, D. Vistica, J. T. Warren, H. Bokesch, S. Kenney, and M. R. Boyd, J. Natl. Cancer Inst., 1990, 82, 1107. CrossRef