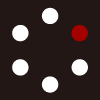
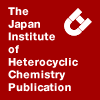
HETEROCYCLES
An International Journal for Reviews and Communications in Heterocyclic ChemistryWeb Edition ISSN: 1881-0942
Published online by The Japan Institute of Heterocyclic Chemistry
e-Journal
Full Text HTML
Received, 23rd April, 2015, Accepted, 20th May, 2015, Published online, 3rd June, 2015.
DOI: 10.3987/COM-15-13235
■ New Synthetic Approach Leading to 1- or 1,3-Disubstituted 2-Thiouracil-5-carboxylates via Dimroth Rearrangement of Isomeric Thiazines
Veronika Hladíková, Richard Kammel, and Jiří Hanusek*
Institute of Organic Chemistry and Technology, University of Pardubice, Studentská 573, Pardubice, 532 10, Czech Republic
Abstract
Methyl 1-substituted and 1,3-disubstituted-2-thiouracil-5-carboxylates (3b-d) were prepared using new synthetic approach involving base-catalyzed cyclization of easily available S-[3-methoxy-2-(methoxycarbonyl)-3-oxoprop-1-en-1-yl]isothiuronium salts (1a-d) to methyl 2-substituted imino-4-oxo-3,4-dihydro-2H-1,3-thiazine-5-carboxylates (2a-d) and their nucleophile-assisted Dimroth rearrangement to methyl 1-substituted and 1,3-disubstituted-2-thiouracil-5-carboxylates (3b-d). Cyclization step is very sensitive towards the base used – e.g. 1a react with stronger bases or bases in excess to give bis-[3-methoxy-2-(methoxycarbonyl)-3-oxoprop-1-en-1-yl]sulfide (4) as the only isolated product.INTRODUCTION
Thiouracil represents well known heterocyclic scaffold with significant biological activity, e.g. antithyroidal (propylthiouracil1) or anti-HIV2,3 properties. For example, ethyl 2-thiouracil-5-carboxylate was recently used4 as a ligand in new dinuclear and mononuclear copper(I) halide complexes with significant cytotoxic activity against human pulmonary carcinoma cells. Thiouracils can also serve as intrinsic photoaffinity probes of nucleic acid structure and nucleic acid-protein interactions.5 Therefore, the synthesis of thiouracil derivatives is still of interest.6 There exist two principal methods of synthesis of 2-thiouracil-5-carboxylates involving either condensation of (un)substituted thiourea with alkoxymethylidenemalonates7 (Scheme 1) or the reaction of triazadienium iodides with acyl chlorides.8 However both these methods have one substantial disadvantage – they were applied only for the synthesis of unsubstituted and 3-substituted-2-thiouracil-5-carboxylates. In the case of reaction of alkoxymethylidenemalonate with 1,3-disubstituted thioureas (dimethyl, diphenyl), corresponding thiobarbituric7 acids were surprisingly formed instead of expected 1,3-disubstituted-2-thiouracil-5-carboxylates (Scheme 1).
In this work, we discovered new synthetic approach (Scheme 2) involving cyclization of S-[3-methoxy-2-(methoxycarbonyl)-3-oxoprop-1-en-1-yl]isothiuronium salts (1a-d) to methyl 2-substituted imino-4-oxo-3,4-dihydro-2H-1,3-thiazine-5-carboxylates (2a-d) and their Dimroth rearrangement to isomeric methyl 1-substituted and 1,3-disubstituted- 2-thiouracil-5-carboxylates (3b-d).
RESULTS AND DISCUSSION
In the first step, we have prepared and characterized isothiuronium chlorides (1a-d) from easily available dimethyl (chlormethylidene)malonate10 and corresponding (un)substituted thiourea. This reaction proceeds very quickly through a nucleophilic vinylic substitution11 (SNV). While the reaction of structurally similar diethyl (ethoxymethylidene)malonate with thioureas involves nucleophilic attack through nitrogen (see Scheme 1) in our case we observed only attack through sulfur, which resulted in the formation of isothiuronium salt 1a-d (Scheme 2). This S-attack was confirmed by NMR measurements and in the case of diethyl analogue of 1b also by X-ray diffraction. It is obvious that the quality of a leaving group (chlorine vs. alkoxy group) in the starting methylidenemalonate is responsible for the difference in the product structure. Thioureas primarily behave as S-nucleophiles towards electrophilic centers. However thiourea also represents relatively good nucleofuge. In the case of reaction with polarized double bond (either carrying chlorine or alkoxy group in β-position) the attack through sulfur is always faster than the attack through nitrogen to give corresponding SNV zwitterionic adduct (kinetic control). This adduct can either undergo fast reverse decomposition to starting compounds or the cleavage of a leaving group. If this leaving group (e.g. Cl) is better than thiourea, then S-product is irreversibly formed. On the other hand if the reverse cleavage of the thiourea is faster than the cleavage of a leaving group (e.g. alkoxy), then slower N-attack giving more thermodynamically stable product occurs. We found only one exception in the literature12 involving reaction of diethyl (ethoxymethylidene)malonate with imidazol-2-thiol (which is “bridged” form of N,N’-disubstituted thiourea) which gives ethyl 5-oxoimidazo[2,1-b][1,3]thiazin-6-carboxylate.
Both salts 1a and 1b are completely stable in the solid state and it can be stored under ambient conditions for several days. However, in aqueous solution they undergo slow decomposition to thiourea and dimethyl (hydroxymethylidene)malonate. Both salts carrying phenyl group (1c-d) are unstable even in the solid state at –18 °C and it has to be freshly prepared and characterized prior to the next reaction step. Due to high instability of 1c-d in aqueous and DMSO solution, only the 1H NMR spectra were measured.
Due to the instability of prepared salts 1a-d in solution the optimal reaction conditions for their cyclization giving thiazines 2a-d were sought. It was found that all strong bases in excess (sodium hydroxide, sodium methoxide, triethylamine) cause complete decomposition of these salts to a complex mixture of many products. For example when salt 1a was treated with an equivalent of triethylamine (pKa = 10.75) in water, no cyclization was observed and only bis-[3-methoxy-2-(methoxycarbonyl)-3-oxoprop-1-en-1-yl]sulfide (4) was isolated (Scheme 3). Its formation must involve the cleavage of cyanamide from conjugated base of 1a to give 3-methoxy-2-(methoxycarbonyl)-3-oxoprop-1-ene-1-thiolate which subsequently acts as a S-nucleophile towards the second molecule of starting salt 1a to give 4.
Therefore weaker base (aqueous ammonia, pKa = 9.25) was used to accomplish the cyclization of 1a, 1c and 1d. Indeed, in the case of reaction of salt 1a the expected methyl 2-imino-4-oxo-3,4-dihydro-2H-1,3-thiazin-5-carboxylate (2a) was isolated in 35% yield together with sulfide 4 (ca 5%). The identity of 2a was confirmed by 1H and 13C-NMR spectra. While the isomeric8 methyl 2-thiouracil-5-carboxylate (3) has chemical shift of CH at 7.96 ppm and for C=S at 176.5 ppm (in DMSO-d6), our product 2a has chemical shifts 8.32 ppm and 165.1 ppm, respectively. The latter 13C-NMR chemical shift is typical13 for N–C(S)=N grouping in iminothiazines.
Completely different reaction product was isolated when salts 1c and 1d were treated with one equivalent of aqueous ammonia. In both cases no thiazine 2c or 2d was detected and only corresponding isomeric 2-thiouracil-5-carboxylates 3c or 3d were isolated in moderate yields. Their structures were confirmed by NMR spectra as well. Both 13C-NMR spectra of 3c or 3d contain peak typical for C=S group in 2-thiouracil8 at 177.5 ppm and 177.1 ppm, respectively. However, there exist two positional isomers of 2-thiouracils carrying phenyl group in the position 1- or 3-. In the literature7 both 1H and 13C-NMR spectra can be found for ethyl 3-phenyl-2-thiouracil-5-carboxylate (Scheme 1, R: Ph). These spectra are completely different than those measured for our product 3c. For instance, the 1H-NMR chemical shift for H-6 proton in 3c is 8.26 ppm, whereas for 3-phenyl isomer7 is 8.08 ppm. In order to prove the position of the phenyl group in 3c and 3d we conducted 1H-13C heteronuclear multiple-bond correlation (HMBC) experiments, which clearly identified long-range coupling between the proton H-6 and the carbon C-1 of the phenyl group.
Above-mentioned cyclization method using ammonia completely failed for the isothiuronium salt 1b. In this case, the formation of a complex mixture of sparingly separable products was observed. Therefore methanolic solution of 1b was treated with one equivalent of sodium methoxide at room temperature. After 90 min, the reaction mixture was evaporated and the solid residue was submitted to column chromatography to yield product whose composition corresponds to thiazine 2b or thiouracil 3b. According to 13C-NMR (typical signal for C=S group at 177.6 ppm) and NOESY NMR experiment which confirms non-bonding interaction between hydrogens of 1-methyl group and the proton H-6, the structure 3b has been proven.
From the observation that the thiouracils 3b-d are formed from 1b-d it is clear that the reaction mechanism must involve two steps. First step involves expected cyclization of 1b-d to 2b-d (like in the case of 1a). However the thiazines formed are unstable under basic conditions and they are undergoing a nucleophile-assisted Dimroth rearrangement to give 2-thiouracils 3b-d. This Dimroth rearrangement must involve addition of any nucleophile present in the reaction mixture to the activated position 6-, followed by the ring opening to give N-acylthiourea intermediate. Such intermediate then undergoes another SNV reaction – i.e. nucleophilic attack through nitrogen and elimination of primary nucleophile.
According to the literature retrieval, only two examples14,15 of nucleophile-assisted Dimroth rearrangement are known which involve the transformation of structurally similar 2-imino-2H-1,3-thiazines to corresponding pyrimidin-2-thiones. Other examples concerns nucleophile-assisted Dimroth rearrangement of either 6-imino-6H-1,3-thiazines16 or their precursors.17 From the synthetic point of view, the reaction sequence involving cyclization and Dimroth rearrangement leading to 1-substituted or 1,3-disubstituted thiouracils has not been described yet.
CONCLUSION
The major synthetic methods of 2-thiouracil-5-carboxylates reported so far have structure limitations. They can only be applied for the synthesis of unsubstituted and 3-substituted-2-thiouracil-5-carboxylates. On the other hand our method starting from rather unstable isothiuronium salts gives corresponding 2-imino-1,3-thiazines which immediately undergo nucleophile-assisted Dimroth rearrangement to 1-substituted and 1,3-disubstituted-2-thiouracil derivatives. Although the isolated yields are moderate, the method is operationally simple.
EXPERIMENTAL
The 1H and 13C-NMR spectra were recorded on a Bruker Avance 3 - 400 MHz instrument in DMSO-d6 solution. Chemical shifts δ are referenced to the solvent residual peaks δ (DMSO-d6) = 2.50 (1H) and 39.6 (13C) ppm. Coupling constants J are quoted in Hz. 13C NMR spectra were also measured in a standard way and by means of the APT (Attached Proton Test) pulse sequence to distinguish CH, CH3 and CH2, Cquart. All NMR experiments were performed with the aid of the manufacturer’s software. Mass spectra were recorded on a MALDI LTQ Orbitrap XL (Thermo Fisher Scientific, Bremen, Germany) equipped with nitrogen UV laser (337 nm, 60 Hz, 8–20 μJ) in positive ion mode. For the CID experiment using the linear trap quadrupole (LTQ), helium was used as the collision gas and 2,5-dihydroxybenzoic acid (DHB) as the MALDI matrix. Starting dimethyl (chlormethylidene)malonate was always freshly prepared using published method.10 All thioureas and other chemicals were purchased from commercial suppliers and used as received.
General procedure for the preparation of isothiuronium salts 1a-d
Corresponding thiourea (3 mmol) was dissolved in 50 mL of MeCN and the solution of dimethyl (chlormethylidene)malonate (0.54 g, 3 mmol) in 5 mL of MeCN was added in one portion. Reaction mixture was stirred for 2 h at room temperature and then precipitated crystals of isothiuronium salts 1a-d were filtered-off and washed with cold MeCN and well dried in a vacuum desiccator.
S-[3-Methoxy-2-(methoxycarbonyl)-3-oxoprop-1-en-1-yl]isothiuronium chloride (1a): white solid, yield: 0.60 g (78%), mp 141-144 °C; 1H NMR: δ 3.75 and 3.79 (2×s, 6H, 2×OCH3), 8.47 (s, 1H, CH), 9.85 and 10.11 (2×bs, 4H, 2×NH2). 13C NMR: δC: 52.8, 53.1, 121.5, 147.7, 162.6, 164.3, 166.3 Anal. Calcd for C7H11ClN2O4S: C, 33.01; H, 4.35; N, 11.00; S, 12.59; Cl, 13.92. Found: C, 32.79; H, 4.35; N, 10.85; S, 12.31; Cl, 14.11. HRMS (MALDI) Calcd. for C7H11N2O4S [M–Cl–]+ 219.0434. Found: 219.0435.
S-[3-Methoxy-2-(methoxycarbonyl)-3-oxoprop-1-en-1-yl]-N,N’-dimethylisothiuronium chloride (1b): white solid, yield: 0.65 g (77%), mp 160-163 °C; 1H NMR: δ 2.99. and 3.04 (2×s, 6H, 2×NCH3), 3.74 and 3.80 (2×s, 6H, 2×OCH3), 8.44 (s, 1H, CH), 10.31 and 10.55 (2×bs, 2H, 2×NH). 13C NMR: δC: 31.6, 32.2, 52.7, 52.9, 121.3, 150.3, 162.5, 162.9, 164.4. Anal. Calcd for C9H15ClN2O4S: C, 38.23; H, 5.35; N, 9.91; S, 11.34; Cl, 12.54. Found: C, 38.27; H, 5.38; N, 9.89; S, 11.32; Cl, 12.36. HRMS (MALDI) Calcd. for C9H15N2O4S [M–Cl–]+ 247.0747. Found: 247.0748.
S-[3-Methoxy-2-(methoxycarbonyl)-3-oxoprop-1-en-1-yl]-N-phenylisothiuronium chloride (1c): white solid, yield: 0.84 g (83%); mp 155-157 °C (decomp.); 1H NMR: δ 3.76 and 3.81 (2×s, 6H, 2×OCH3), 7.37-7.41 (m, 3H, Ar-H), 7.49-7.53 (m, 2H, Ar-H), 8.68 (s, 1H, CH), 9.99 (vbs, 2H, NH+NH2). HRMS (MALDI) Calcd. for C13H15N2O4S [M–Cl–]+ 295.0741. Found: 295.0744.
S-[3-Methoxy-2-(methoxycarbonyl)-3-oxoprop-1-en-1-yl]-N-methyl-N’-phenylisothiuronium chloride (1d): white solid, yield: 0.90 g (87%); mp 145-148 °C (decomp.); 1H NMR: δ 3.15 (s, 3H, NCH3), 3.71 and 3.72 (2×s, 6H, 2×OCH3), 7.35-7.40 (m, 3H, Ar-H), 7.44-7.49 (m, 2H, Ar-H), 8.56 (s, 1H, CH), 10.96 (vbs, 1H, NH). Calcd. for C13H15N2O4S [M–Cl–]+ 309.0898. Found: 309.0907.
General procedure for the transformation of isothiuronium salts 1a-d to 2a and 3b-d
Method A: To a suspension of isothiuronium salt 1a, 1c or 1d (0.5 g) in 5 mL of water 1 equivalent of conc. aqueous ammonia was added. The solution or suspension was stirred for 30 min, and then precipitated white solid was filtered-off and crystallized from MeCN (2a) or MeOH (3c or 3d) to give analytically pure products. When salt 1a was treated with triethylamine instead of ammonia, product 4 precipitated instead of 2a.
Method B: To a solution of isothiuronium salt 1b (0.5 g) in 5 mL of MeOH one equivalent of MeONa was added. After stirring for 90 min, the solution was evaporated and semi-solid residue was purified by column chromatography (silica gel, hexane/EtOAc 1:4) to give pure 3b.
Methyl 2-imino-4-oxo-3,4-dihydro-2H-1,3-thiazin-5-carboxylate (2a): white solid; yield 0.12 g (35%); mp 186-192 °C; 1H‑NMR: δH: 3.77 (s, 1H, OCH3); 8.37 (s, 1H, CH); 8.49 (bs, 2H, 2×NH). 13C NMR: δC: 52.3; 122.3; 141.0; 162.9; 164.9; 165.1. Anal. Calcd for C6H6N2O3S: C, 38.70; H, 3.25; N, 15.05; S, 17.22. Found: C, 38.49; H, 3.31; N, 15.01; S, 17.24. HRMS (MALDI) Calcd for C6H6N2O3S [M+H]+ 187.0172. Found: 187.0172.
Methyl 1,3-dimethyl-2-thiouracil-5-carboxylate (3b): white solid; yield: 0.20 g (52%); mp 149-152 °C; 1H‑NMR: δH: 3.58 (s, 1H, NCH3); 3.74 (s, 1H, NCH3); 3.75 (s, 1H, OCH3); 8.74 (s, 1H, CH). 13C NMR: δC: 35.0; 40.2; 51.9; 105.5; 150.6; 156.3, 162.9, 177.6. Anal. Calcd for C8H10N2O3S: C, 44.85; H, 4.70; N, 13.08; S, 14.97. Found: C, 44.75; H, 4.55; N, 12.99; S, 14.73. HRMS (MALDI) Calcd for C8H10N2O3S [M+H]+ 215.0485. Found: 215.0485.
Methyl 1-phenyl-2-thiouracil-5-carboxylate (3c): white solid; yield: 0.21 g (54%); mp 276-279 °C; 1H‑NMR: δH: 3.71 (s, 1H, OCH3); 7.45-7.53 (m, 5H, Ar-H), 8.26 (s, 1H, CH), 13.06 (bs, 1H, NH). 13C NMR: δC: 51.9; 107.7; 127.8; 129.4; 129.4; 142.1, 151.1, 156.9, 162.6, 177.6. Anal. Calcd for C12H10N2O3S: C, 54.95; H, 3.84; N, 10.68; S, 12.23. Found: C, 54.94; H, 3.78; N, 10.49; S, 12.20. HRMS (MALDI) Calcd for C12H10N2O3S [M+H]+ 263.0485. Found: 263.0482.
Methyl 3-methyl-1-phenyl-2-thiouracil-5-carboxylate (3d): white solid; yield: 0.24 g (61%); mp 199-202 °C; 1H‑NMR: δH: 3.62 (s, 3H, NCH3); 3.72 (s, 1H, OCH3); 7.42-7.45 (m, 2H, Ar-H), 7.46-7.55 (m, 3H, Ar-H), 8.37 (s, 1H, CH). 13C NMR: δC: 35.0, 52.0; 105.9; 127.7; 129.3; 129.5; 143.5, 149.1, 156.3, 162.6, 177.1. Anal. Calcd for C13H12N2O3S: C, 56.51; H, 4.38; N, 10.14; S, 11.60. Found: C, 56.63; H, 4.26; N, 9.99; S, 11.50. HRMS (MALDI) Calcd for C13H12N2O3S [M+H]+ 277.0641. Found: 277.0643.
Bis-[3-methoxy-2-(methoxycarbonyl)-3-oxoprop-1-en-1-yl]sulfide (4): white solid; yield: 0.08 g (13%); mp 91-92 °C; 1H‑NMR: δH: 3.75 and 3.77 (2×s, 12H, 4×OCH3); 8.83 (s, 2H, CH). 13C NMR: δC: 48.7, 52.6, 121.8, 154.5, 162.9, 163.9. Anal. Calcd for C12H14O8S: C, 45.28; H, 4.43; S, 10.07. Found: C, 45.10; H, 4.53; S, 9.98. HRMS (MALDI) Calcd for C12H14O8S [M+H]+ 319.0477. Found: 319.0484.
ACKNOWLEDGEMENTS
The authors thank to Ministry of Education, Youth and Sports of the Czech Republic for institutional support.
References
1. M. Weissel, Exp. Clin. Endocrinol. Diabetes, 2010, 118, 101; CrossRef D. S. Cooper and S. A. Rivkees, J. Clin. Endocrinol. Metabol., 2009, 94, 1881. CrossRef
2. A. Mai, M. Artico, G. Sbardella, S. Massa, A. G. Loi, E. Tramontano, P. Scano, and P. La Colla, J. Med. Chem., 1995, 38, 3258. CrossRef
3. C. Mugnaini, F. Manetti, J. A. Esté, I. Clotet-Codina, G. Maga, R. Cancio, M. Botta, and F. Corelli, Bioorg. Med. Chem. Lett., 2006, 16, 3541. CrossRef
4. I. Papazoglou, P. J. Cox, A. G. Hatzidimitriou, C. Kokotidou, T. Choli-Papadopoulou, and P. Aslanidis, Eur. J. Med. Chem., 2014, 78, 383. CrossRef
5. A. Favre, C. Saintomé, J. L. Fourrey, P. Clivio, and P. Laugâa, J. Photochem. Photobiol., B, 1998, 42, 109. CrossRef
6. T. Pospieszny, I. Małecka, and Z. Paryzek, Tetrahedron Lett., 2010, 51, 4166. CrossRef
7. E. Ballard and T. B. Johnson, J. Am. Chem. Soc., 1942, 64, 794; CrossRef C. W. Whitehead and J. J. Traverso, J. Am. Chem. Soc., 1956, 78, 5294; CrossRef S. Botsi and A. Tsolomitis, Heterocycl. Commun., 2007, 13, 229. CrossRef
8. C. Landreau, D. Deniaud, A. Reliquet, F. Reliquet, and J. C. Meslin, J. Heterocycl. Chem., 2001, 38, 93. CrossRef
9. G. Sbardella, S. Castellano, C. Vicidomini, D. Rotili, A. Nebbioso, M. Miceli, L. Altucci, and A. Mai, Bioorg. Med. Chem. Lett., 2008, 18, 2788. CrossRef
10. R. M. Coates and S. J. Hobbs, J. Org. Chem., 1984, 49, 140; CrossRef G. Egri, E. Fogassy, L. Novák, and L. Poppe, Tetrahedron: Asymmetry, 1997, 8, 547. CrossRef
11. T. Okuyama and G. Lodder, Adv. Phys. Org. Chem., 2002, 37, 1; CrossRef C. F. Bernasconi and Z. Rappoport, Acc. Chem. Res., 2009, 42, 993. CrossRef
12. J. P. Clayton, P. J. O’Hanlon, and T. J. King, J. Chem. Soc., Perkin Trans. 1, 1980, 1352. CrossRef
13. I. Yavari, M. Nematpour, and Z. Hossaini, Monatsh. Chem., 2010, 141, 229. CrossRef
14. W. Schroth, R. Spitzner, M. M. Ezzat, M . Richter, and S. Freitag, J. Prakt. Chem., 1990, 332, 148. CrossRef
15. T. E. Glotova, N. I. Protsuk, L. V. Kanitskaya, G. V. Dolgushin, and V. A. Lopyrev, Chem. Heterocycl. Compd., 2004, 40, 1595. CrossRef
16. D. Briel, Heterocycles, 2003, 60, 2273; CrossRef D. Briel, Heterocycles, 2004, 63, 2319. CrossRef
17. A. Lorente, L. Vaquerizo, A. Martín, and P. Gómez-Sal, Heterocycles, 1995, 41, 71. CrossRef