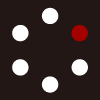
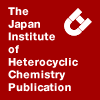
HETEROCYCLES
An International Journal for Reviews and Communications in Heterocyclic ChemistryWeb Edition ISSN: 1881-0942
Published online by The Japan Institute of Heterocyclic Chemistry
e-Journal
Full Text HTML
Received, 27th April, 2015, Accepted, 28th May, 2015, Published online, 8th June, 2015.
DOI: 10.3987/COM-15-13238
■ A Simple, Efficient and Green Procedure for Knoevenagel Condensation in Hydroxy-Functionalized Ionic Liquids
Yuting Liu, Rong Li, and Yanjun Xing*
College of Chemistry, Chemical Engineering and Biotechnology, Donghua University, Renmin Road 2999#, Shanghai 201620, China
Abstract
An efficient and simple Knoevenagel condensation catalyzed by hydroxy-functionalized ionic liquids proceeded smoothly in high yields under ambient and solvent-free conditions. The condensation procedures of aryl aldehydes and 2,4-thiazolidinedione was involved in hydrogen bonding interactions between the hydroxy groups of the ILs and the carbonyl group of the aldehyde. The ionic liquids can be reused for five times without significant loss in activity.INTRODUCTION
As an alternative green medium, ionic liquids have been found widespread applications such as in analysis, synthesis, catalysis and separation.1-4 Physicochemical properties and reactivity of ionic liquids can be easily tuned by selecting proper combination of organic cations with anions and rational functionalization of ions or the substitutes.5,6 Ionic liquids derived from natural product choline chloride7 have been reported and ionic liquids with hydroxy-containing functional group could get close to the charge-carrying core of the cation. Without losing the potential solvent properties and benefits of anionic liquid system,8 enzymatic catalysts in ionic liquids and other organo-active systems could be stabilized by providing a hydrogen-bond-rich microenvironment. Therefore, introduction of hydroxy-groups into ionic liquids9 have attracted lots of chemists’ attention in the last decade.
As a common and classic method for the formation of the C-C bond in organic synthesis, Knoevenagel condensation has been used to obtain a range of substituted alkenes and α,β-unsaturated nitriles.10,11 2,4-Thiazolidinedione derivatives were important structural elements in medicinal chemistry and played significant role in pharmaceutical chemistry.11,12 Meanwhile, they all could be prepared through the Knoevenagel condensation between aldehydes and active methylene compounds. Synthesis of 2,4-thiazolidinedione derivatives via condensation of 2,4-thiazolidinedione with aryl aldehydes have been a subject of considerable interest. Most of the existing protocols for 2,4-thiazolidinediones suffered from drastic conditions, multi-step, metal-catalysis and tedious work-up procedures.13-17 Though functionalized ionic liquids18-20 had been used to promote this reaction, disadvantages including long reaction times, low yields and poor thermal stability for recycling in these catalytic procedures remained to be solved. Therefore, facile and efficient procedures for 2,4-thiazolidinedione derivatives is still desirable.
Herein, we have developed four novel hydroxy-functionalized ionic liquids based on amidines and halohydrins to catalyze Knoevenagel condensation of aryl aldehydes with 2,4-thiazolidinedione efficiently. Much to our delight, these ionic liquids could also be used to promote the condensation of aryl aldehydes and ethyl cyanoacetate. Moreover, these catalysts can be reused several times with considerable reaction activity in these two applications, offering a convenient and green route to Knoevenagel condensation reaction.
RESULTS AND DISCUSSION
As shown in Scheme 1 and experimental section, the hydroxy-functionalized ionic liquids were synthesized from amidines and halohydrins.
The structures of all the synthesized ionic liquids are confirmed by 1H NMR, 13C NMR, IR and ESI-MS. The chemical shift of 1H NMR of -CH2-N+= (imino nitrogen) and 13C NMR of -CH2-N+= are both downfield in all the ionic liquids compared to the raw amides. This indicated that only the imino-nitrogen was cationalized, which agreed with the results of previously reported investigations.21 These changes of structure after reactions completed can also be demonstrated via the IR spectra. The symmetric stretching of N=C bond in amidines varied either in the range of 1670 cm-1 to 1677 cm-1 or 1622 cm-1 to 1665 cm-1, which had redshifts of 20 cm-1 to 27 cm-1 and 17 cm-1 to 44 cm-1, respectively. The presence of peaks at 2944, 2949, 2939 and 2951 cm-1 in IR, respectively, confirmed the formation of C-N+(-C)=C in all ionic liquids.
Initially, Knoevenagel condensation was conducted using benzaldehyde and 2,4-thiazolidinedione as model reaction (Table 1). The control experiment showed that the presence of catalyst was necessary (entry 1). Attempts to increase the efficiency by varying concentrations of 3a at 60 °C (entries 2-5) indicated that 0.2 equiv of 3a was the best. The product was observed in 93% yield, which was much higher than that of dicationic imidazolium-based ionic liquid.22 The yield increased as the reaction time increased, the yield came to the best when the mixture reacted 60 min (entries 5 and 6). The temperature was also an important factor for the reaction (entries 6-9). Increasing the temperature increased the yields significantly. However, as the temperature was further raised to 90 °C, yield of 6a decreased slightly to 93%. Therefore, 80 °C was chosen as the optimal temperature, in which the yield (95%) was much higher than that of Alum and ionic liquid [TMG][Lac].16,23
Ionic liquids 3b-3d were also used as catalysts for Knoevenagel condensation. The experiment (entry 8) revealed that 3a provided higher yield than the other three ones (entries 10-12). The results indicated that the cation of ionic liquids influenced the reaction efficiency, which can be attributed to stronger hydrogen bond and less steric hindrance of 3a. Furthermore, the nucleophilicity of active methylene caused by H-bonding interaction between chloride anion of 3a was higher than that of bromide anion. 3a was also proved better when compared with typical ionic liquids [DBN-Bu]Cl24 (entry 13), [BMIM]Cl (entry 14) and hydroxy-functionalized imidazolium ionic liquid [HOEtMIM]Cl (entry 15).
With the optimized reaction conditions established, we embarked on the evaluation of the substrate scope to the reaction of 2,4-thiazolidinedione with various aryl aldehydes (Table 2). The effects of substituents at the aromatic ring on the Knoevenagel condensation were also studied. Aryl aldehydes bearing para-, ortho-, meta-substituents were all tolerated and the corresponding products in excellent yields. The reaction time of electron-withdrawing group substituted aromatic aldehydes was shorter than that of electron-donating group substituted aromatic aldehydes (entries 2-6).23 Furthermore, the reaction activity of aryl aldehyde can be enhanced due to H-bonding interaction between substituents hydroxy or fluoro and 3a (entries 1 and 5). These comparative experiments (entries 2-4 and 6) also showed that the formation of hydrogen-bond played an important role in the process of the catalytic reaction. Meanwhile, we also found that the hindrance of substituents such as orth- and meta-substituted groups could retard the reaction and decreased the product yields (entries 4, 7 and 6, 10).
With the results of synthesis of 5-arylidene-2,4-thiazolidinediones in hand, we next tried our attempts to use these ILs to promote the condensation reaction of aryl aldehydes and ethyl cyanoacetate. It was notable that these reactions proceeded smoothly to give corresponding products in excellent yields at room temperature. Only E isomers were obtained when ethyl cyanoacetate was used as the active methylene compound, and this was confirmed by 1H NMR and melting point data of all the products.25 The results were summarized in Table 3.
The recovery and reusability of 3a were also investigated. As shown in Figure 1, 3a remained a highly catalytic activity for synthesis of 6a and 8a with five runs. Compared with the traditional catalysts, the easy recycling performance was also an attractive property of the ionic liquids for the environmental protection and economic reasons.
On the basis of the reported work previously23,26 and our researches concerning on the synthesis of 5-benzylidene-2,4-thiazolidinedione catalyzed by 3a, a plausible reaction mechanism was depicted in Scheme 2. The hydrogen atom of the active methylene in 4a interacts with a lone pair of electrons of nitrogen in IL catalysts to form a carbon anion, which facilitates the attack of carbonyl carbon followed by the formation of C-C bond.22,26 Meanwhile, the hydroxy group of ionic liquids interacts with the carbonyl group in C5 to form the hydrogen bonding interactions, which therefore increase the electrophilicity of carbonyl groups attached. Moreover, nucleophilicity of active methylene can also be enhanced because of H-bonding interaction between chloride anion of 3a and active methylene of 2,4-thiazolidinedione.22 Naturally, the condensation reaction proceeds efficiently due to the multiple modes of interaction.
The mechanism could be verified by comparing the chemical shift of 13C NMR concerned about the raw materials and their adduct intermediates of 3a, which was shown in Figure 2. It is found that the 13C NMR chemical shift of active methyl in 2,4-thiazolidinedione is found at 36.29 ppm instead of 30.66 ppm in 2,4-thiazolidinedione-3a mixture. The chemical shift is upfield by 5.63 ppm compared to pure 2,4-thiazolidinedione, which indicates a carbon anion formed.
It is also noted that the chemical shift of carbonyl in benzaldehyde was found at 193.23 ppm instead of 193.76 ppm in benzaldehyde-3a mixture (Table 4). The chemical shift is downfield by 0.53 ppm, which indicates an interaction between the hydroxy groups of 3a with the carbonyl group of benzaldehyde shown in supporting information.
In summary, four novel hydroxy-functionalized ionic liquids based on amidines were successfully synthesized. These ionic liquids can be used as facile and recyclable catalyst/solvent in Knoevenagel condensation of aryl aldehydes with active methylene compounds in considerably high yields. These ionic liquids could be recovered by simple procedures and reused for five times, which was economic and would make contribution to the development of catalysts used in green and continuous chemical processes.
EXPERIMENTAL
General procedures for hydroxy-functionalized ionic liquids
1-[2-(2-Hydroxyethoxy)ethyl]-1,5-diazabicyclo[4.3.0]non-5-ene chloride (3a): 2-(2-Chloroethoxy)ethanol (1.11 g, 10 mmol) was added to a solution of 1,5-diazabicyclo[4.3.0]non-5-ene (1.24 g, 10 mmol) dissolved in EtOAc (20 mL). The mixture was stirred at 80 °C for 24 h under N2 atmosphere. The reaction was monitored by TLC. Upon completion, the solvent was evaporated under reduced pressure and the residue was washed with Et2O to give the product. The product was dried in vacuum for 24 h at 100 °C. Yield 97%, colorless oil. 1H NMR (400 MHz, D2O) δ: 3.62 (t, J = 4.0 Hz, 2H), 3.56-3.58 (m, 4H), 3.47-3.51 (m, 4H), 3.35 (t, J = 8.0 Hz, 2H), 3.26 (t, J = 4.0 Hz, 2H), 2.90 (t, J = 8.0 Hz, 2H), 1.89-2.03 (m, 4H); 13C NMR (100 MHz, D2O) δ: 165.08, 72.00, 67.16, 60.26, 54.06, 52.22, 44.46, 41.91, 30.24, 18.55, 17.86; IR (KBr): 3324, 2944, 1670, 1449, 1313, 1109, 1061; MS (ESI) for the cation of [C11H21O2N2]Cl: m/z = 213.1. Anal. Calcd for C11H21N2O2Cl: C, 53.17; H, 8.51; N, 11.26. Found: C, 53.24; H, 8.59; N, 11.15.
1-(3-Hydroxypropyl)-1,5-diazabicyclo[4.3.0]non-5-ene bromide (3b): 3-Bromo-1-propanol (1.38 g, 10 mmol) was added to a solution of 1,5-diazabicyclo[4.3.0]non-5-ene (1.24 g, 10 mmol) dissolved in EtOAc (20 mL). The mixture was stirred at room temperature for 24 h under N2 atmosphere. The reaction was monitored by TLC. Upon completion, the solvent was evaporated under reduced pressure and the residue was washed with Et2O to give the product. The product was dried in vacuum for 24 h at 100 °C. Yield 99%, white solid, mp 39.8 °C. 1H NMR (400 MHz, D2O) δ: 3.58 (t, J = 8.0 Hz, 2H), 3.52 (t, J = 8.0 Hz, 2H), 3.38 (t, J = 8.0 Hz, 2H), 3.31 (t, J = 8.0 Hz, 2H), 3.26 (t, J = 8.0 Hz, 2H), 2.89 (t, J = 8.0 Hz, 2H), 1.99-2.10 (m, 2H), 1.93-1.97 (m, 2H), 1.75-1.80 (m, 2H); 13C NMR (100 MHz, D2O) δ: 164.41, 58.34, 53.94, 49.55, 43.88, 42.01, 29.96, 29.01, 18.53, 17.79; IR (KBr): 3318, 2949, 1677, 1447, 1315, 1101, 1060; MS (ESI) for the cation of [C10H19ON2]Br: m/z =183.1. Anal. Calcd for C10H19N2OBr: C, 45.63; H, 7.28; N, 10.65. Found: C, 45.62; H, 7.33; N, 10.54.
1-[2-(2-Hydroxyethoxy)ethyl]-1,8-diazabicyclo[5.4.0]und-7-ecene chloride (3c) was prepared as the procedure of 3a. Yield 95%, colorless oil. 1H NMR (400 MHz, D2O) δ: 3.57-3.63 (m, 4H), 3.50 (t, J = 4.0 Hz, 2H), 3.38-3.44 (m, 4H), 3.18 (t, J = 8.0 Hz, 4.0 Hz, 2H), 2.73-2.78 (m, 2H), 2.50 (t, J = 8.0 Hz, 2H), 1.92-1.96 (m, 2H), 1.54-1.59 (m, 6H); 13C NMR (100 MHz, D2O) δ: 167.06, 72.10, 67.77, 60.49, 54.84, 52.80, 48.84, 46.87, 32.59, 27.63, 25.29, 22.54, 19.65; IR (KBr): 3325, 2939, 1622, 1445, 1321, 1116, 1069; MS (ESI) for the cation of [C13H25O2N2]Cl: m/z = 241.1. Anal. Calcd for C13H25N2O2Cl: C, 56.41; H, 9.10; N, 10.12. Found: C, 56.51; H, 9.04; N, 10.07.
1-(3-Hydroxypropyl)-1,8-diazabicyclo[5.4.0]und-7-ecene bromide (3d) was prepared as the procedure of 3b. Yield 93%, colorless oil. 1H NMR (400 MHz, D2O) δ: 3.62 (t, J = 4.0 Hz, 2H), 3.56-3.58 (m, 4H), 3.47-3.51 (m, 4H), 3.35 (t, J = 8.0 Hz, 2H), 3.26 (t, J = 8.0 Hz, 2H), 2.90 (t, J = 8.0 Hz, 2H), 1.89-2.03 (m, 6H); 13C NMR (100 MHz, D2O) δ: 166.40, 58.43, 54.84, 50.48, 48.80, 46.87, 32.88, 30.29, 27.69, 25.59, 22.57, 19.65; IR (KBr): 3327, 2951, 1665, 1446, 1310, 1109, 1061; MS (ESI) for the cation of [C12H23ON2]Br: m/z = 211.2. Anal. Calcd for C12H23N2OBr: C, 49.49; H, 7.96; N, 9.62. Found: C, 49.45; H, 8.07; N, 9.59.
General procedure for Knoevenagel condensation
A round bottom flask was charged with aryl aldehyde (10 mmol), 2,4-thiazolidinedione (10 mmol) and ionic liquid (2 mmol). The reaction mixture was stirred and monitored by TLC. Upon completion, water was added and the mixture was stirred. The mixture was allowed to stand to separate into two layers, affording the product and ionic liquid. The separated solid product was suction-filtered and further purified by crystallization from hot EtOH. The filtrate containing the ionic liquid was then evaporated under reduced pressure, and the ionic liquid was reused directly for the next run. The melting point and spectra data of products are given as below.
5-Benzylidene-2,4-thiazolidinedione (6a):22 white solid. mp 240-242 °C; 1H NMR (400 MHz, DMSO-d6) δ: 12.65 (s, 1H, NH), 7.81 (s, 1H, CH), 7.62 (m, 2H, ArH), 7.56 (m, 3H, ArH); 13C NMR (100 MHz, DMSO-d6) δ: 168.32, 167.75, 133.49, 132.26, 130.89, 130.47, 129.71, 123.92.
5-(4-Hydroxybenzylidene)-2,4-thiazolidinedione (6b):27 white solid, mp 281-284 °C. 1H NMR (400 MHz, DMSO-d6) δ: 12.49 (s, 1H, NH), 10.34 (s, 1H, OH), 7.74 (s, 1H, CH), 7.47 (m, 2H, ArH), 6.92 (m, 2H, ArH); 13C NMR (100 MHz, DMSO-d6) δ: 168.53, 167.97, 160.35, 132.87, 124.39, 119.45, 116.80.
5-(4-Methylbenzylidene)-2,4-thiazolidinedione (6c):22 white solid, mp 224-227 °C. 1H NMR (400 MHz, DMSO-d6) δ: 12.59 (s, 1H, NH), 7.77 (s, 1H, CH), 7.51 (d, J = 8.0 Hz, 2H, ArH), 7.37 (d, J = 8.0 Hz, 2H, ArH), 2.37 (s, 3H, CH3); 13C NMR (100 MHz, DMSO-d6) δ: 168.33, 167.84, 141.18, 132.34, 130.73, 130.54, 130.41, 122.76, 21.89.
5-(4-Methoxybenzylidene)-2,4-thiazolidinedione (6d):22 white solid, mp 217-220 °C. 1H NMR (400 MHz, DMSO-d6) δ: 12.53 (s, 1H, NH), 7.74 (s, 1H, CH), 7.54 (d, J = 8.0 Hz, 2H, ArH), 7.08 (d, J = 8.0 Hz, 2H, ArH), 2.09 (s, 3H, CH3); 13C NMR (100 MHz, DMSO-d6) δ: 168.41, 167.87, 161.43, 132.54, 132.29, 125.94, 120.65, 115.34, 55.82.
5-(4-Chlorobenzylidene)-2,4-thiazolidinedione (6e):22 yellow solid, mp 223-224 °C. 1H NMR (400 MHz, DMSO-d6) δ: 12.68 (s, 1H, NH), 7.78 (s, 1H, CH), 7.58-7.63 (m, 4H, ArH); 13C NMR (100 MHz, DMSO-d6) δ: 168.08, 167.66, 135.45, 132.39, 132.08, 129.83, 124.77.
5-(4-Fluorobenzylidene)-2,4-thiazolidinedione (6f):28 white solid, mp 221-224 °C. 1H NMR (400 MHz, DMSO-d6) δ: 12.69 (s, 1H, NH), 7.81 (s, 1H, CH), 7.63-7.65 (m, 4H, ArH); 13C NMR (100 MHz, DMSO-d6) δ: 168.22, 167.69, 161.71, 132.97, 132.88, 131.17, 130.20, 123.71, 117.07, 116.85.
5-(4-Nitrobenzylidene)-2,4-thiazolidinedione (6g):28 white solid, mp 259-262 °C. 1H NMR (400 MHz, DMSO-d6) δ: 12.74 (s, 1H, NH), 7.81 (s, 1H, CH), 7.65 (d, J = 8.0 Hz, 2H, ArH), 7.62 (d, 2H, J = 8.0 Hz, ArH); 13C NMR (100 MHz, DMSO-d6) δ: 168.17, 167.64, 135.47, 132.42, 132.10, 130.85, 129.87, 124.91.
5-(2-Chlorobenzylidene)-2,4-thiazolidinedione (6h):29 yellow solid, mp 289-230 °C. 1H NMR (400 MHz, DMSO-d6) δ: 12.79 (s, 1H, NH), 7.93 (s, 1H, CH), 7.58 (d, J = 8.0 Hz, 2H, ArH), 7.52-7.56 (m, 2H, ArH); 13C NMR (100 MHz, DMSO-d6) δ: 168.10, 167.45, 134.92, 132.32, 131.48, 130.82, 129.35, 128.59, 127.75, 127.16.
5-(3-Hydroxybenzylidene)-2,4-thiazolidinedione (6i):29 white solid, mp 250-251 °C. 1H NMR (400 MHz, DMSO-d6) δ: 12.61 (s, 1H, NH), 9.86 (s, 1H, OH), 7.69 (s, 1H, CH), 7.33 (t, J = 8.0 Hz, 1H, ArH), 7.03 (d, J = 8.0 Hz, 2H, ArH), 6.98 (s, 1H, ArH), 6.88 (d, J = 8.0 Hz, 1H, ArH); 13C NMR (100 MHz, DMSO-d6) δ: 168.42, 167.79, 158.32, 134.65, 132.46, 130.83, 123.74, 121.78, 118.20, 116.38.
5-(3-Methoxybenzylidene)-2,4-thiazolidinedione (6j):29 white solid, mp 186-188 °C. 1H NMR (400 MHz, DMSO-d6) δ: 12.64 (s, 1H, NH), 7.76 (s, 1H, CH), 7.45 (t, J = 8.0 Hz, 1H, ArH), 7.14 (s, 1H, ArH), 7.05 (d, J = 8.0 Hz, 1H, ArH), 3.80 (s, 3H, CH3); 13C NMR (100 MHz, DMSO-d6) δ: 168.30, 167.73, 160.08, 134.86, 132.22, 130.89, 122.32, 116.76, 115.78, 55.82.
5-(4-Nitrobenzylidene)-2,4-thiazolidinedione (6k):29 white solid, mp 180-182 °C. 1H NMR (400 MHz, DMSO-d6) δ: 12.80 (s, 1H, NH), 8.42 (s, 1H, CH), 8.27 (d, J = 8.0 Hz, 1H, ArH), 7.99 (t, J = 8.0 Hz, 1H, ArH), 7.93 (s, 1H, ArH), 7.81 (t, J = 8.0 Hz, 1H, ArH); 13C NMR (100 MHz, DMSO-d6) δ: 167.74, 167.47, 148.67, 135.88, 135.20, 131.33, 129.82, 127.05, 124.90, 124.79.
Ethyl (E)-2-cyano-3-phenyl-2-propenoate (8a):30 white solid, mp 49-50 °C. 1H NMR (400 MHz, DMSO-d6) δ: 8.41 (s, 1H, CH), 8.05 (d, J = 8.0 Hz, 2H, ArH), 7.58-7.65 (m, 3H, ArH), 4.30-4.35 (m, 2H, CH2), 1.31 (t, J = 8.0 Hz, 1H, ArH); 13C NMR (100 MHz, DMSO-d6) δ: 162.25, 155.56, 133.87, 131.74, 131.19, 129.79, 116.05, 103.08, 62.85, 14.61.
Ethyl (E)-2-cyano-3-(4-hydroxyphenyl)-2-propenoate (8b):30 white solid, mp 168-170 °C. 1H NMR (400 MHz, DMSO-d6) δ: 10.86 (s, 1H, OH), 8.25 (s, 1H, CH), 8.00 (d, J = 8.0 Hz, 2H, ArH), 6.95 (d, J = 8.0 Hz, 2H, ArH), 4.27-4.32 (m, 2H, CH2), 1.30 (t, J = 8.0 Hz, 3H, CH3); 13C NMR (100 MHz, DMSO-d6) δ: 163.35, 163.08, 155.16, 134.53, 123.25, 116.94, 97.31, 61.82, 14.30.
Ethyl (E)-2-cyano-3-(4-methylphenyl)-2-propenoate (8c):31 white solid, mp 90-92 °C. 1H NMR (400 MHz, DMSO-d6) δ: 8.37 (s, 1H, CH), 7.98 (d, J = 8.0 Hz, 2H, ArH), 7.41 (d, J = 8.0 Hz, 2H, ArH), 4.29-4.35 (m, 2H, CH2), 2.41(s, 3H, CH3), 1.31 (t, J = 8.0 Hz, 3H, CH3); 13C NMR (100 MHz, DMSO-d6) δ: 162.43, 155.46, 144.90, 131.36, 130.45, 129.15, 116.25, 101.54, 62.41, 21.60, 14.61.
Ethyl (E)-2-cyano-3-(4-methoxyphenyl)-2-propenoate (8d):30 white solid, mp 80-82 °C. 1H NMR (400 MHz, DMSO-d6) δ: 8.32 (s, J = 8.0 Hz, 1H, CH), 8.07 (d, J = 8.0 Hz, 2H, ArH), 7.14 (d, J = 8.0 Hz, 2H, ArH), 4.28-4.33 (m, 2H, CH2), 3.87 (s, 3H, CH3), 1.31 (t, J = 8.0 Hz, 3H, CH3); 13C NMR (100 MHz, DMSO-d6) δ: 162.00, 154.16, 138.48, 132.91, 130.71, 129.95, 115.76, 103.65, 63.05, 14.31.
Ethyl (E)-2-cyano-3-(4-chlorophenyl)-2-propenoate (8e):30 yellow solid, mp 91-92 °C. 1H NMR (400 MHz, DMSO-d6) δ: 8.42 (s, 1H, CH), 8.07 (d, J = 8.0 Hz, 2H, ArH), 7.68 (d, J = 8.0 Hz, 2H, ArH), 4.30-4.36 (m, 2H, CH2), 1.32 (t, J = 8.0 Hz, 3H, CH3); 13C NMR (100 MHz, DMSO-d6) δ: 162.0, 154.21, 138.44, 132.83, 130.81, 129.88, 115.78, 103.62, 62.80, 14.31.
Ethyl (E)-2-cyano-3-(4-fluorophenyl)-2-propenoate (8f):32 white solid, mp 94-95 °C. 1H NMR (400 MHz, DMSO-d6) δ: 8.43 (s, 1H, CH), 8.15-8.19 (m, 2H, ArH), 7.48 (t, J = 8.0 Hz, 2H, ArH), 4.30-4.36 (m, 2H, CH2), 1.32 (t, J = 8.0 Hz, 3H, CH3); 13C NMR (100 MHz, DMSO-d6) δ: 166.39, 163.78, 162.13, 154.45, 133.57, 128.21, 117.22, 117.00, 115.57, 102.68, 62.86, 14.30.
Ethyl (E)-2-cyano-3-(4-nitrophenyl)-2-propenoate (8g):30 white solid, mp 170-172 °C. 1H NMR (400 MHz, DMSO-d6) δ: 8.58 (s, 1H, CH), 8.41 (d, J = 8.0 Hz, 2H, ArH), 8.25 (d, J = 8.0 Hz, 2H, ArH), 4.34-4.39 (m, 2H, CH2), 1.33 (t, J = 8.0 Hz, 3H, CH3); 13C NMR (100 MHz, DMSO-d6) δ: 161.75, 153.12, 149.79, 137.84, 132.14, 124.53, 115.27, 107.30, 62.79, 14.28.
Ethyl (E)-2-cyano-3-(2-chlorophenyl)-2-propenoate (8h):33 yellow solid, mp 46-48 °C. 1H NMR (400 MHz, DMSO-d6) δ: 8.53 (s, 1H, CH), 8.11 (d, J = 8.0 Hz, 2H, ArH), 7.56-7.63 (m, 3H, ArH), 4.32-4.38(m, 2H, CH2), 1.32 (t, J = 8.0 Hz, 3H, CH3); 13C NMR (100 MHz, DMSO-d6) δ: 161.59, 151.02, 135.17, 134.68, 130.74, 130.23, 130.03, 128.40, 115.11, 107.16, 62.81, 13.93.
Ethyl (E)-2-cyano-3-(3-methoxyphenyl)-2-propenoate (8i):33 white solid, mp 52-54 °C. 1H NMR (400 MHz, DMSO-d6) δ: 8.31 (s, 1H, CH), 8.08 (d, J = 8.0 Hz, 2H, ArH), 7.14 (d, J = 8.0 Hz, 2H, ArH), 4.28-4.33 (m, 2H, CH2), 3.88 (s, 3H, CH3), 1.32 (t, J = 8.0 Hz, 3H, CH3); 13C NMR (100 MHz, DMSO-d6) δ: 164.01, 162.72, 154.79, 133.90, 124.36, 116.58, 115.42, 99.00, 62.41, 56.09, 14.32.
Ethyl (E)-2-cyano-3-(3-nitrophenyl)-2-propenoate (8j):30 white solid, mp 130-131 °C. 1H NMR (400 MHz, DMSO-d6) δ: 8.94 (s, 1H, CH), 8.62 (s, 1H, OH), 8.44 (d, J = 8.0 Hz, 2H, ArH), 7.90 (t, J = 8.0 Hz, 2H, ArH), 4.33-4.39(m, 2H, CH2), 1.34 (t, J = 8.0 Hz, 3H, CH3); 13C NMR (100 MHz, DMSO-d6) δ: 161.77, 153.30, 148.53, 136.93, 133.31, 131.46, 127.58, 125.42, 115.45, 105.95, 63.14, 14.31.
ACKNOWLEDGEMENTS
This work was supported by the National Science and Technology Ministry (ID 2012BAK30B03) and the Fundamental Research Funds for the Central Universities (No. 2232013A3-05 and CUSF-DH-D2013048).
References
1. F. Xue, C. G. Li, Y. Zhu, T. J. Lou, and G. J. He, Heterocycles, 2014, 89, 2739. CrossRef
2. A. Kaur and V. Singh, Tetrahedron Lett., 2015, 56, 1128; CrossRef C. Zhuo, D. Xian, X. Hui, and L. Mei, Heterocycles, 2011, 83, 1121. CrossRef
3. J. Akbari, A. Ebrahimi, and A. Heydari, Tetrahedron Lett., 2014, 55, 5417. CrossRef
4. A. Hazra, Y. P. Bharitkar, A. Maity, S. Mondal, and N. B. Mondal, Tetrahedron Lett., 2013, 54, 4339; CrossRef H. B. Zhu, Y. C. Fan, Y. L. Qian, H. F. Tang, Z. Ruan, D. H. Liu, and H. Wang, Chin. Chem. Lett., 2014, 25, 465. CrossRef
5. Z. F. Fei, T. J. Geldbach, D. B. Zhao, and J. P. Dyson, Chem. Eur. J., 2006, 12, 2122. CrossRef
6. N. V. Plechkova and K. R. Seddon, Chem. Soc. Rev., 2008, 37, 123; CrossRef H. Tokuda, S. Tsuzuki, M. A. B. H. Susan, K. Hayamizu, and M. Watanabe, J. Phys. Chem. B., 2006, 110, 19593. CrossRef
7. A. P. Abbott, G. Capper, D. L. Davies, H. L. Munro, R. K. Rasheed, and V. Tambyrajah, Chem. Commun., 2001, 2010. CrossRef
8. M. B. Turner, S. K. Spear, J. G. Huddleston, J. D. Holbrey, and R. D. Rogers, Green Chem., 2003, 5, 443. CrossRef
9. L. C. Branco, J. N. Rosa, J. J. M. Ramos, and C. A. M. Afonso, Chem. Eur. J., 2002, 8, 3671. CrossRef
10. L. F. Tietze, Pure Appl. Chem., 2004, 76, 1967. CrossRef
11. J. W. Rumer, S. Y. Dai, M. Levick, L. Biniek, D. J. Procter, and I. McCulloch, J. Polym. Sci., Polym. Chem., 2013, 51, 1285. CrossRef
12. Y. Li, Y. Zhang, X. Shen, and Y. W. Guo, Bioorg. Med. Chem. Lett., 2009, 19, 390. CrossRef
13. J. F. Zhou, F. X. Zhu, Y. Z. Song, and Y. L. Zhu, ARKIVOC, 2006, xiv, 175.
14. U. R. Pratap, D. V. Jawale, R. A. Waghmare, D. L. Lingampallea, and R. A. Mane. New J. Chem., 2011, 35, 49. CrossRef
15. M. L. Wrobleski, G. A. Reichard, S. Paliwal, S. Shah, H. C. Tsui, R. A. Duffy, J. E. Lachowicz, C. A. Morgan, G. B. Varty, and N. Y. Shih, Bioorg. Med. Chem. Lett., 2006, 16, 3859. CrossRef
16. K. F. Shelke, S. B. Sapkal, G. K.Kakade, A. Sandip, B. B. Sadaphala, and M. S. Shingare, Green Chem. Lett. Rev., 2010, 3, 17. CrossRef
17. D. H. Yang, B. Y. Yang, B. C. Chen, and S. Y. Chen, Org. Prep. Proced. Int., 2006, 38, 81. CrossRef
18. K. Gong, Z. W. He, Y. Xu, D. Fang, and Z. L. Liu, Monatsh. Chem., 2008, 139, 913. CrossRef
19. D. Morison, D. C. Forbes, and J. H. Davis, Jr, Tetrahedron Lett., 2001, 42, 6053. CrossRef
20. K. F. Shelke, S. B. Sapkal, B. R. Madje, B. B. Shingate, and M. S. Shingare, Bull. Catal. Soc. India, 2009, 8, 30.
21. A. G. Ying, L. Liu, G. F. Wu, G. Cheng, X. Z. Chen, and W. D. Ye, Tetrahedron Lett., 2009, 50, 1653. CrossRef
22. D. V. Jawale, U. R. Pratap, D. L. Lingampalle, and R. A. Mane, Chin. J. Chem., 2011, 29, 942. CrossRef
23. Suresh and J. S. Sandhu, Org. Med. Chem. Lett., 2013, 3, 1. CrossRef
24. J. Nowicki, M. Muszynski, and S. Gryglewicz, J. Chem. Technol. Biotechnol., 2014, 89, 48. CrossRef
25. T. Hayashi, J. Org. Chem., 1966, 31, 3253. CrossRef
26. A. G. Ying, Y. X. Ni, S. L. Xu, S. Liu, J. G. Yang, and R. R. Li, Ind. Eng. Chem. Res., 2014, 53, 5678. CrossRef
27. N. Sachan, S. S. Kadam, and V. M. Kulkarni, Ind. J. Heterocycl. Chem., 2007, 17, 45.
28. K. M. Al-Zaydi, J. Saudi Chem. Soc., 2010, 14, 91. CrossRef
29. D. H. Yang, B. Y. Yang, and B. C. Chen, Org. Prep. Proced. Int., 2006, 38, 81. CrossRef
30. J. C. Zhang, T. Jiang, B. X. Han, A. L. Zhu, and X. M. Ma, Synth. Commun., 2006, 36, 3305. CrossRef
31. X. Xin, X. Guo, H. F. Duan, Y. J. Lin, and H. Sun, Catal. Commun., 2007, 8, 115. CrossRef
32. S. H. Zhao, X. J. Wang, and L. W. Zhang, RSC Adv., 2013, 3, 11691. CrossRef
33. G. W. Li, J. Xiao, and W. Q. Zhang, Green Chem., 2011, 13, 1828. CrossRef