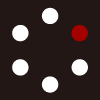
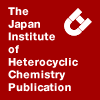
HETEROCYCLES
An International Journal for Reviews and Communications in Heterocyclic ChemistryWeb Edition ISSN: 1881-0942
Published online by The Japan Institute of Heterocyclic Chemistry
e-Journal
Full Text HTML
Received, 4th May, 2015, Accepted, 19th June, 2015, Published online, 9th July, 2015.
DOI: 10.3987/COM-15-13240
■ Development of a Practical Synthesis of 7-Bromo-8-methoxycarbonyl-3,3-dimethyl-3,4-dihydro-1H-quinoxalin-2-one
Kazuhiro Kudo,* Takahiro Honda, and Noriyoshi Yamamoto
Product Supply Division, Santen Pharmaceutical Co., Ltd., 348-3, Aza Suwa Oaza Shide Taga-cho Inugami-gun Shiga, Japan
Abstract
Synthesis of 7-bromo-8-methoxycarbonyl-3,3-dimethyl-3,4-dihydro-1H-quinoxalin-2-one from 5-amino-2-bromobenzoic acid was achieved in an overall yield of 27% over four steps. This is a significant improvement from the previous six-step process, which afforded only a 12% yield. The previous process was optimized by adding a high-yielding alkylation step under mild conditions as well as by reducing the number of isolation steps and eliminating purification by column chromatography.INTRODUCTION
Glucocorticoids are commonly used to treat inflammatory diseases. Apart from their potent anti-inflammatory activities, long-term and high dose treatment may lead to serious adverse effects.1 Selective glucocorticoid receptor agonists (SEGRA) that can separate an anti-inflammatory activity, and their adverse effects are reported in recent years.1,2 SEGRA compounds with 3,3-dimethyl-3,4-dihydro-1H-quinoxalin-2-one framework (Figure 1) have been developed as candidates for SEGRA and show a remarkable anti-inflammatory effect after topical dosing to the eye and side-effect dissociation by in vivo assay.2b-e The structure of SEGRA compounds was not a steroid framework and was reportedly the most desirable example of drug efficacy to have dimethyl functions in 3, 3-position and carbonyl function at 2-position. To evaluate their activities in preclinical and clinical studies, an efficient, reliable, and cost-effective synthetic method is required. Thus, a practical synthesis of a 3,3-dimethyl-3,4-dihydro-1H-quinoxalin-2-one framework must be developed for the construction of SEGRA compounds.
In the conventional synthetic route, a number of SEGRA compounds were synthesized from key intermediate 1a (Scheme 1).2b-e A six-step procedure was used to synthesize 1a, the first step was the reduction of the nitro group on 3 by SnCl2 (94% yield). This was followed by the protection of the amino group on 4a (98% yield), nitration of 5, and purification by silica gel column chromatography to afford 6 in 62% yield. Deacetylation afforded 7 in quantitative yield, and alkylation with ethyl 2-bromoisobutyrate (2a) and isolation by silica gel column chromatography afforded 8a in 31% yield. Finally, reduction of the nitro group and successive intramolecular cyclization and column chromatography provided 1a in 70% yield. Although 1a was successfully synthesized in an overall 12% yield over six steps, this method has many disadvantages, such as low yield, use of a halogenated solvent, and several purification steps using silica gel column chromatography. Step 5 was particularly problematic as it resulted in a low yield (31%), excess use of 2a as a solvent, use of expensive Cs2CO3, high temperature, and long reaction time (85 °C, 4 d). Purification after this step was also difficult and required two consecutive purifications by silica gel column chromatography. Since this synthetic method was found to be very difficult, an inexpensive, stable, and more efficient synthetic route for 1a is desirable.
The reason for the resultant low yield in step 5 was assumed to be that the tertiary bromide 2a is a hindered electrophile and 7 shows low nucleophilicity due to the presence of a strong electron withdrawing group in the o-position. To improve the yield of alkylation, we selected 4 as a starting material, which does not have a strong electron withdrawing group and was easy to procure. Alkylation with 2 was investigated under mild conditions. We also explored the nitration of 8 to afford 1, completing this new synthetic route (Scheme 2).
RESULTS AND DISCUSSION
Alkylation of tertiary, hindered electrophile 2 with 4 was investigated.3 In the case of 4a and 2a, base/solvent combinations of K2CO3/DMF, Et3N/DMF, and Et3N/2-PrOH at 50 °C did not proceed at all and only the starting material 4a was recovered (Table 1, entries 1–3). When carboxylic acid 4b was reacted in K2CO3/DMF, 4b and O-alkylation product A were found in a ratio of 59:41, with no detection of target material 9b (entry 4). In the case of Et3N/DMF, the conversion was relatively high (76%) and the remaining 4b, 9b, and A were found in a ratio of 24:41:35 (entry 5).4 In Et3N/2-PrOH, the conversion was low and mainly recovered 4b, a small amount of 9b, and A (85:12:3, entry 6).
Conversely, alkylation of 4b and carboxylic acid 2b in K2CO3/DMF showed low conversion to 9d (44%). However, mainly 4b and 9d were observed in the reaction mixture, with 4b being converted to target material 9d in high amounts (Table 2, entry 1). In the case of Et3N/DMF, 99% conversion was observed; however, only 15% was target material 9d, while the rest were impurities (entry 2). The use of 2-PrOH as the solvent and Et3N as the base gave high conversion with few impurities, affording 9d in 85% yield (entry 3). In the case of methyl ester 4a, 85% conversion was confirmed and the yield of target material 9c slightly decreased to 68% (entry 4). The use of EtOH as the solvent resulted in a decreased yield of 48% due to residual unreacted 4b (entry 5). When MeOH was used as the solvent, a further decrease in conversion was observed (24%) and only the remaining 4b and 9d were confirmed. In the reaction mixtures in Table 2, impurity B was not observed through 1H NMR spectroscopic analysis.
The lower yield of the alkylation reaction shown in Table 2 was due to the decreased steric effect of the alcohol, which was investigated through the addition of H2O to 2-PrOH. The reaction was performed at 50 °C for 18 h and with the same equivalents of reagents. Under these conditions, the ratio of the remaining 4b increased in proportion to H2O addition and the conversion decreased (Table 3).
Therefore, the combination of carboxylic acid 2b and 4b in Et3N/2-PrOH afforded the best results, with high conversion and high yield of 9d. Furthermore, it was found that the addition of H2O or a low steric effect solvent decreased the yield of 9d. The mechanism was believed to involve 2b forming highly active intermediate 10, which could then react with the amino group on 4b (Scheme 3). Active intermediate 10 was able to react with H2O and low steric effect alcohols, resulting in low yields.6 In the case of O-alkylation to yield B, the retro-O-alkylation proceeds intramolecularly due to the vicinal effect of the carboxylic acid, making B unstable.
The nitration reaction of 9 was subsequently investigated (Table 4). Reaction of dicarboxylic acid 9d in both HNO3/H2SO4 and NaNO3/TFA7 yielded a complex mixture with a small amount of remaining 9d (entries 1 and 2). Nitration of 9c, where R4 is a methyl ester, in NaNO3/TFA also yielded a complex mixture and no remaining 9c (entry 3). The reaction mixture of dimethyl ester 9e in HNO3/H2SO4 contained not only remaining 9e and target material 8e but also regioisomer 11e and a slight amount of dinitration product 12e (27:42:23:8, entry 4).8 For the reaction of 9e in NaNO3/TFA, no 9e remained and the reaction progress was confirmed as the ratio of target material 8e, 11e, and 12e was 62:22:16, with a 57% HPLC assay yield of 8e.9 Impurities were removed through recrystallization in 2-PrOH, affording 8e in 38% yield and 99% purity. It was found that dimethyl esterification from 9d to 9e was necessary for the nitration reaction and was accomplished in 80% yield by stirring 9d in MeOH in the presence of H2SO4.
In the final synthesis of 1a, the use of SnCl2 and MeOH under reflux afforded 1a in 70% yield with a conventional method.2b-e Our investigation revealed that Fe powder (φ = 45 μm) with AcOH/MeOH (1:1) reduced the nitro group, followed by an intramolecular cyclization to provide 1a in 96% yield (Scheme 4).10
Optimization of the total synthetic procedure was investigated to further improve the synthesis of 1a. Products 9d and 9e were found to be an amorphous solid and liquid, respectively. They were thus difficult to purify by simple methods such as recrystallization (Scheme 5). When the alkylation step was complete, the reaction solvent was substituted with MeOH and H2SO4 was added, allowing for a subsequent esterification. Upon completion, water soluble salts and impurities were removed by extraction. The organic layer was substituted with TFA, and nitration was performed by the addition of NaNO3 without the isolation of 9e. The reaction mixture was then extracted, the organic layer was substituted for 2-PrOH, and 8e was recrystallized in 29% yield from 4b11 and 99.0% HPLC purity. This was then reduced and cyclized with Fe, which was then removed by filtration and extracted with aqueous HCl using a reduction-cyclization step. Finally, the precipitated solid was washed with n-hexane/AcOEt (5:1) to afford 1a in 93% yield with over 99.9% HPLC purity.
In the previous synthesis, the total yield of 1a was 12% with six steps. It required six isolation steps and purification by four silica gel columns. With this improved procedure, 1a was produced in 27% yield with no need for purification by silica gel column chromatography and with only two isolation steps (Table 5).
CONCLUSION
A practical, efficient four-step process for the synthesis of 1a was developed. This novel procedure afforded 1a in high purity and an improved overall yield of 27% compared with the previous yield of 12%. Alkylation with hindered electrophiles 2b and 4b afforded 9d in high yield when a combination of Et3N and 2-PrOH was used. Nitration with NaNO3 in TFA preferentially afforded 8e at the desired position with good purity following recrystallization in 2-PrOH. Final product 1a was synthesized using Fe in the nitro reduction of 8e and cyclization, resulting in high yield and purity. Optimization of this synthetic procedure resulted in reducing the isolation steps from six to two, and did not require purification by column chromatography.
EXPERIMENTAL
GENERAL
Unless otherwise noted, all materials were obtained from commercial suppliers and used without further purification. 1H NMR and 13C NMR spectra were recorded on a JEOL GSX400 or JEOL ECP500 or JEOL JNM-ECS400 spectrometer at ambient temperature. Chemical shifts are reported as ppm downfield from tetramethylsilane. Data are reported as follows: chemical shift, multiplicity (s = singlet, d = doublet, t = triplet, q = quartet, br = broad, m = multiplet), coupling constants, and the number of protons. HPLC analyses were conducted on a Waters alliance series. In process method for preparations of 9d, 9c and 9e; C8 column (3.5 μm; 4.6 mm I.D. × 150 mm) (X BridgeTM), Mobile phase A: 0.05% TFA in water, Mobile phase B: 0.05% TFA in MeCN, Gradient condition: 0 min 95% A, 5 min 95% A, 25 min 10% A, 30 min 10% A, column temperature 40 °C, 1.0 mL/min flow rate, UV detector at 225 nm. Retention times: 4b (6.3 min), 9d (13.6 min), 9c (16.5 min), 9e (19.0 min). In process method for preparations of 8e and 1a; C8 column (3.5 μm; 4.6 mm I.D. × 150 mm) (X BridgeTM), isocratic elution with 10 mM CH3CO2NH4 aq/MeCN (60:40), column temperature 40 °C, 1.0 mL/min flow rate, UV detector at 225 nm. Retention times: 1a (4.9 min), 9e (9.8 min), 8e (13.7 min), 11e (16.8 min), 12e (18.9 min).
2-Bromo-5-(1-carboxy-1-methylethylamino)benzoic acid (9d). To a mixture of 5-amino-2-bromobenzoic acid 4b (10.0 g, 46.3 mmol), 2-bromoisobutyric acid 2b (11.6 g, 69.5 mmol) and anhydrous 2-PrOH (100 mL) was added Et3N (26.0 ml, 0.187 mol), and the mixture was stirred for 20 h at 50 °C. When the reaction was completed, the reaction mixture was evaporated under vacuum pressure. Water (300 mL) and 6M HCl (20 mL) were added and extracted with AcOEt (150 mL×2). The combined organic layer was dried over anhydrous Na2SO4, filtered, and evaporated under vacuum pressure to achieve the 9d as a pale yellow solid (12.3 g, 88%). HPLC purity: 95.8%, which was used in the next reaction without any purification. 1H NMR (DMSO-d6, 400 MHz) δ: 1.42 (s, 6H), 6.51 (dd, J = 8.8, 2.9 Hz, 1H), 6.87 (d, J = 2.9 Hz, 1H), 7.33 (d, J = 8.8 Hz, 1H), 12.86 (br s, 2H); 13C NMR (DMSO-d6, 100 MHz) δ: 25.52, 55.99, 104.57, 115.15, 117.11, 133.40, 133.66, 145.96, 167.67, 176.80; IR (KBr) cm−1: 1035, 1157, 1218, 1257, 1473, 1601, 1717, 2510, 2769, 2987, 3387; Mp 105 °C; HRMS (EI): calcd for C11H12BrNO4 [M+]: 300.9950. Found; 300.9952.
Methyl 2-Bromo-5-(1-carboxy-1-methylethylamino)benzoate (9c). To a mixture of methyl 5-amino-2-bromobenzoate 4a (0.436 g, 1.90 mmol), 2-bromoisobutyric acid 2b (0.479 g, 2.87 mmol) and anhydrous 2-PrOH (4.5 mL) was added Et3N (1.07 mL, 7.68 mmol), and the mixture was stirred for 19 h at 50 °C. When the reaction was completed, the reaction mixture was evaporated under vacuum pressure. Water (30 mL) and 1M HCl (5 mL) were added and extracted with AcOEt (15 mL×2). The combined organic layer was washed with brine and dried over anhydrous Na2SO4. After filtration, the organic solution was concentrated to dryness under reduced pressure. The residue was was purified by column chromatography to afford 9c as a pale yellow solution (0.407 g, 68%). 1H NMR (DMSO-d6, 400 MHz) δ: 1.42 (s, 6H), 3.81 (s, 3H), 6.54 (dd, J = 8.8, 3.3 Hz, 1H), 6.88 (d, J = 3.3 Hz, 1H), 7.36 (d, J = 8.8 Hz, 1H), 12.47 (s, 1H); 13C NMR (DMSO-d6, 100 MHz) δ: 25.52, 52.36, 56.04, 104.54, 115.26, 117.54, 132.11, 133.83, 146.09, 166.57, 176.74; IR (KBr) cm−1: 1032, 1154, 1254, 1334, 1437, 1474, 1601, 1725, 2949, 2991, 3388; HRMS (EI): calcd for C12H14BrNO4 [M+]: 315.0106. Found; 315.0090.
Methyl 2-Bromo-5-(1-methoxycarbonyl-1-methylethylamino)benzoate (9e). To a mixture of 2-bromo-5-(1-carboxy-1-methylethylamino)benzoic acid 9d (10.8 g, 35.8 mmol) and anhydrous MeOH (150 mL) was added 12% HCl (15 mL), and the mixture was stirred for 22 h at 70 °C. When the reaction was completed, the reaction mixture was evaporated under vacuum pressure. Water (200 mL) was added and extracted with AcOEt (120 mL×2). The combined organic layer was washed with brine and dried over anhydrous Na2SO4. After filtration, the organic solution was concentrated to dryness under reduced pressure. The residue was purified by chromatography to afford 9e as a colorless solution (9.44 g, 80%). 1H NMR (DMSO-d6, 400 MHz) δ: 1.45 (s, 6H), 3.61 (s, 3H), 3.81 (s, 3H), 6.46 (dd, J = 8.7, 2.7 Hz, 1H), 6.51 (s, 1H), 6.85 (d, J = 2.7 Hz, 1H), 7.37 (d, J = 8.7 Hz, 1H); 13C NMR (DMSO-d6, 100 MHz) δ: 25.55, 52.25, 52.37, 56.31, 104.87, 115.51, 117.19, 132.10, 133.99, 145.78, 166.41, 175.64; IR (KBr) cm−1: 1031, 1147, 1247, 1335, 1386, 1436, 1475, 1516, 1601, 1733, 2953, 2994, 3398; HRMS (EI): calcd for C13H16BrNO4 [M+]: 329.0263. Found; 329.0260.
Methyl 6-Bromo-3-(1-methoxycarbonyl-1-methylethylamino)-2-nitrobenzoate. (8e). To a mixture of methyl 2-bromo-5-(1-methoxycarbonyl-1-methylethylamino)benzoate 9e (0.861g, 2.61 mmol) and TFA (4.30 mL) was added NaNO3 (0.224g, 2.63 mmol) at 50 °C, and the mixture was stirred for 5 h at 50 °C. When the reaction was completed, the reaction mixture was evaporated under vacuum. Water (10 mL) and 4M NaOH aq (4.0 mL) were added and extracted with AcOEt (15 mL×2). The combined organic layer was dried over anhydrous Na2SO4, filtered, and evaporated under vacuum pressure. 2-PrOH was added and the mixture was stirred for 20 h, and the precipitated solid was filtered. The filtered cake was further washed with 2-PrOH (0.5 mL) and dried in vacuo at 50 °C to afford compound 8e as an orange solid (0.368 g, 38%). 1H NMR (DMSO-d6, 400 MHz) δ: 1.61 (s, 6H), 3.70 (s, 3H), 3.86 (s, 3H), 6.70 (d, J = 9.3 Hz, 1H), 7.76 (d, J = 9.3 Hz, 1H), 8.01 (s, 1H); 13C NMR (DMSO-d6, 100 MHz) δ: 25.41, 52.98, 53.23, 57.44, 105.20, 118.35, 130.68, 132.59, 138.86, 141.78, 165.24, 174.15; IR (KBr) cm−1: 1031, 1088, 1151, 1217, 1262, 1358, 1387, 1444, 1498, 1605, 1734, 2958, 3004, 3377, 3455; Mp 119 °C; HRMS (EI): calcd for C13H15BrN2O6 [M+]: 374.0113. Found; 374.0113.
7-Bromo-8-methoxycarbonyl-3,3-dimethyl-3,4-dihydro-1H-quinoxalin-2-one (1a). To a mixture of methyl 6-bromo-3-(1-methoxycarbonyl-1-methylethylamino)-2-nitrobenzoate 8e (0.500 g, 1.33 mmol), MeOH (3.75 mL) and AcOH (3.75 mL) was added Fe (φ=45 μm) (0.224 g, 4.01 mmol) at ice cooling, and the mixture was stirred for 12 h at rt. When the reaction was completed, the MeOH and AcOH were evaporated under vacuum pressure. AcOEt (20 mL) was added and the mixture was sttired for 20 min at 60 °C. The mixture was filtered by celite® and the filtrate was added H2O (20 mL) and extracted. The organic layer was evaporated under vacuum pressure to achieve a pale gray solid (0.400 g, 96%). 1H NMR (DMSO-d6, 400 MHz) δ: 1.22 (s, 6H), 3.84 (s, 3H), 6.46 (s, 1H), 6.72 (d, J = 8.4 Hz, 1H), 7.07 (d, J = 8.4 Hz, 1H), 10.09 (s, 1H); 13C NMR (DMSO-d6, 100 MHz) δ: 24.68, 52.79, 53.71, 105.98, 116.25, 120.96, 124.53, 126.28, 134.01, 165.51, 170.29; IR (KBr) cm−1: 1027, 1160, 1265, 1286, 1311, 1358, 1387, 1436, 1469, 1591, 1691, 1716, 1855, 2969, 3060, 3113, 3206, 3325; Mp 156 °C; HRMS (EI): calcd for C12H13BrN2O3 [M+]: 312.0110. Found; 312.0098.
Optimaized process for 1a. To a mixture of 5-amino-2-bromobenzoic acid 4b (500 g, 2.32 mol), 2-bromoisobutyric acid 2b (580 g, 3.47 mol) and 2-PrOH (5.00 L) was added Et3N (1.29 L, 9.26 mol), and the mixture was stirred for 7 h at 50 °C. When the reaction was completed as indicated by HPLC (conv. >92%), the reaction mixture was filtered and the filtrate was evaporated under vacuum pressure and azeotroped with MeOH (2.5 L×2). Dry MeOH (10 L) and 12% HCl (500 mL) were added to the mixture and the mixture was stirred for 19 h at 70 °C. When the reaction was completed as indicated by HPLC (conv. >90%), the reaction mixture was evaporated under vacuum pressure and water (5.00 L) was added and extracted with AcOEt (5.00 L×2). The combined organic layer was evaporated under vacuum pressure to achieve an oil (641 g). TFA (4.80 L) was added to the oil and stirred at 20 °C. NaNO3 (164.9 g, 1.94 mol) was added to the mixture and stirred for 5 h at 50 °C. When the reaction was completed as indicated by HPLC (conv. >98%), the reaction mixture was evaporated under vacuum pressure and neutralized by 4 M NaOH aq (1.44 L). Water (3.6 L) was added to the mixture and extracted with AcOEt (5.00 L). The organic layer was evaporated under vacuum pressure to achieve an oil. 2-PrOH (1.80 L) was added to the oil and stirred for 12 h at rt. The precipitated solid was filtered and the filtered cake was further washed with 2-PrOH (500 mL) and dried in vacuo to a constant weight at 50 °C to afford compound 8e as an orange solid (247 g, 29% yield from 4b, HPLC purity: 99.0%). To a mixture of methyl 6-bromo-3-(1-methoxycarbonyl-1-methylethylamino)-2-nitrobenzoate 8e (247 g, 0.658 mol), MeOH (1.73 L) and AcOH (1.73 L) was added Fe (φ=45 μm) (110 g, 1.98 mol) at ice cooling, and the mixture was stirred for 23 h at rt. When the reaction was completed as indicated by HPLC (conv. >99%), the reaction mixture was filtered by celite® and the filtrate was evaporated under vacuum pressure. To the mixture was added 1M HCl (3.75 L) and extracted with AcOEt (3.75 L). The organic layer was washed by H2O (3.75 L), filtered and evaporated under vacuum pressure to give a solid. The solid was washed with n-hexane:AcOEt (5:1), (250 mL) and dried in vacuo to a constant weight at 50 °C to afford compound 1a as a pale gray solid (191 g, 93%). HPLC purity: >99.9%.
ACKNOWLEDGEMENTS
We thank Ms. Eiko Nishioka for NMR analysis.
References
1. A. Schottelius, W. D. Döcke, P. Strehlke, S. Jaroch, N. Schmees, H. Rehwinkel, H. Hennekes, and K. Asadullah, Proc. Natl. Acad. Sci. U.S.A., 2004, 101, 227. CrossRef
2. (a) S. S. Hadida-Ruah, H. E. Xiaohui, and J. Y. Nagasawa, WO2004/110385 A2; (b) M. Matsuda, T. Mori, K. Kawashima, M. Yamamoto, M. Kato, M. Takai, M. Nagatsuka, and S. Kobayashi, WO 2007/105766 A1; (c) M. Matsuda, T. Mori, M. Nagatsuka, and S. Kobayashi, WO 2008/146871 A1; (d) M. Kato, M. Takai, T. Matsuyama, T. Kurose, Y. Hagiwara, M. Matsuda, and T. Mori, WO 2009/035067 A1; (e) M. Kato, M. Takai, T. Matsuyama, T. Kurose, Y. Hagiwara, K. Oki, M. Matsuda, and T. Mori, WO 2010/029986 A1.
3. Examples of alkylation of 2 and 4 homologs were reported before, but the reported yields were not stable for every substrate, see: F. Hoffmann, et al. WO2011/128251 A1.; K. Tachibana, et al. EP1790640 A1.; S. D. Clarke, J. C. Metcalfe, and G. A. Smith, J. Chem. Soc., Perkin Trans. 2, 1993, 1187. CrossRef
4. Compounds 9b and A were assumed by 1H NMR. 9b: 1H NMR (DMSO-d6, 400 MHz) δ: 1.09 (t, J = 7.0 Hz, 3H), 1.44 (s, 6H), 4.08 (q, J = 7.0 Hz, 2H), 6.42 (s, 1H), 6.46 (dd, J = 8.7, 3.2 Hz, 1H), 6.84 (d, J = 3.2 Hz, 1H), 7.33 (d, J = 8.7 Hz, 1H), 13.10 (br s, 1H). A: 1H NMR (400 MHz, DMSO-d6) δ: 1.20 (t, J = 7.0 Hz, 3H), 1.59 (s, 6H), 4.14 (q, J = 7.0 Hz, 2H), 5.59 (s, 2H), 6.64 (dd, J = 8.4, 2.9 Hz, 1H), 6.92 (d, J = 2.9 Hz, 1H), 7.29 (d, J = 8.4 Hz, 1H).
5. Sample preparation of 1H NMR analysis: sampling of small amount from reaction mixture and controlling mild acid by HCl aq and H2O. The mixture was extracted with AcOEt and the organic layer was concentrated to dryness.
6. An addition product of H2O or low steric alcohol was not isolated and not observed by 1H NMR and HPLC analysis.
7. P. P. Fu, L. S. Von Tungeln, L.-H. Chiu, D.-J. Zhan, J. Deck, T. Bucci, and J.-C. Wang, Chem. Res. Toxicol., 1998, 11, 937. CrossRef
8. Compounds 11e and 12e were assumed by 1H NMR. 11e: 1H NMR (DMSO-d6, 400 MHz) δ: 1.63 (s, 6H), 3.74 (s, 3H), 3.88 (s, 3H), 6.97 (s, 1H), 8.18 (s, 1H), 8.34 (s, 1H). 12e: 1H NMR (DMSO-d6, 400 MHz) δ: 1.60 (s, 6H), 3.76 (s, 3H), 3.93 (s, 3H), 8.32 (s, 1H), 8.68 (s, 1H).
9. Although the causes of regioselectivity are unknown, it is assumed to be comprehensive affection of substituents on the aromatic and vicinal effect in methyl carbonyl function.
10. G. C. Coutts, N. J. Culbert, M. E. Edwards, J. A. Hadfield, D. R. Musto, V. H. Pavlidis, and D. J. Richards, J. Chem. Soc., Perkin Trans. 1, 1985, 1829. CrossRef
11. Although 4b can be procured at an appropriate cost, the detailed information is not disclosable. 4b was synthesized from 3-aminobenzoic acid with NBS in 78% in laboratory scale, see: K. Kudou, N. Yamamoto, M. Ban, and A. Ohno, WO 2012/108455 A1.