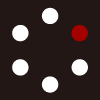
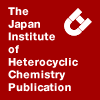
HETEROCYCLES
An International Journal for Reviews and Communications in Heterocyclic ChemistryWeb Edition ISSN: 1881-0942
Published online by The Japan Institute of Heterocyclic Chemistry
e-Journal
Full Text HTML
Received, 11th May, 2015, Accepted, 26th May, 2015, Published online, 8th June, 2015.
DOI: 10.3987/COM-15-13247
■ CoCl2•6H2O-Promoted Pinner-Dimroth Tandem Reaction: Facile Synthesis of 3-Substituted Isoindolinones
Mingxing Liu, Jiarong Li, Qi Zhang, and Daxin Shi*
Department of Chemical Engineering, Beijing Institute of Technology, 5 South Zhongguancun Street, Haidian District, Beijing Postcode: 100081, China
Abstract
A convenient one-pot synthesis of 3-substituted isoindolinones were reported in good yield via Pinner-Dimroth tandem reaction of 1,3-dicarbonyl compounds and 2-cyanobenzaldehyde in the catalysis of CoCl2•6H2O. This method has advantages of time efficiency and mild reaction conditions.Isoindolinone is one of the most important nitrogen heterocycles, being widespread among many naturally occurring substances (Figure 1, aristololactam B,1a nuevamine1b) and present as a key structural unit in a large number of families of therapeutic activities2-8 such as antioxidant,2 anxiolytic,3 antibacterial,4 anticancer,5 platelet aggregation inhibitory,6 antipsychotic7 and reduced the dependence of nicotine8 agents. Therefore, much attention has been devoted to the synthesis of 3-substituted isoindolinones. The classical methods include the condensation of 2-cyanobenzaldehyde with nitroalkanes,9 organolithiums,10 alkenes,11 alcohols,12 amines.13 Palladium-catalyzed reaction of 2-iodo-N-substituted benzamides was proved a highly efficient synthesis of the isoindolinone scaffold.14 But there is still a clear need for versatile methods that give access to 3-substituted isoindolinone systems under environmentally benign conditions, a cheap catalytic system, and easily available starting.
During the development of synthetic methodologies, many advantages in terms of sustainability and green chemistry are offered by one-pot domino or tandem processes. Those methods provide synthetic target products and avoid multistep protocols and tedious purification of intermediates. This kind of strategy has been used for the construction of 3-substituted isoindolinones. For example, Ramström et al.9 successfully assessed a base-promoted tandem reaction to access 3-substituted isoindolinones via Henry reaction, heterocyclization and rearrangement. And in a little while, Massa et al.15 described an efficient method for synthesizing 3-substituted isoindolinones through tandem aldol/cyclization reactions in the presence of potassium carbonate. Mola et al.16 discovered that the electrochemical methodology for synthesizing 3-substitued isoindolinones. Afterwards Massa et al.17 first reported a asymmetric synthesis of 3-substituted isoindolinones by organocatalyst.
In the light of the above considerations and our previous investigations on the Pinner-Dimroth tandem methodologies for the synthesis of dihydroquinazolinones,18 we envisaged CoCl2•6H2O-promoted tandem reaction of 2-cyanobenzaldehyde with 1,3-dicarbonyl compounds to provide a convenient synthesis of 3-substitued isoindolinone derivatives.
Initially, 2-cyanobenzaldehyde (1) and dimethyl malonate (2b) were chosen as model substrates to find the appropriate reaction conditions. As outlined in Table 1, no target product was observed by performing the process without catalyst or in the presence of CuCl2 and BiCl3 (Table 1, entries 1, 4, 5). FeCl3 and AlCl3 could promote this reaction easily under mild conditions (Table 1, entries 2, 3). However, the yield was lower and the reaction time was longer than CoCl2•6H2O (Table 1, entry 7). The catalytic properties of TiCl4 was similar to CoCl2•6H2O (Table 1, entry 6). Taking into account the extremely water-sensitive of TiCl4, we selected CoCl2•6H2O as the appropriate catalyst. The solvent can affect the stability and catalytic behaviors of the catalyst. Gratifyingly, we found the yield was higher using acetonitrile as solvent than using other solvent (Table 1, entries 8, 9). The amount of catalyst also had effect on the reaction, and the 0.4 equivalent amount of CoCl2•6H2O was the appropriate choice (Table 1, entries 10-12). The temperature had no influence on the yield of reaction. So the room temperature was selected (Table 1, entries 13, 14).
With these pleased results in hand, we felt encouraged to explore the substrate scope for this processes, so a series of β-dicarbonyl compounds were studied and the results were presented in Table 2. As shown in Table 2, different β-dicarbonyl compounds had a certain effect on this reaction because they contained various ring tension and steric hindrance. The reaction of 2-cyanobenzaldehyde and malonic acid diesters provided the highest yield (Table 2, entries 2, 7). Cyclic β-diketones tested gave the target products in good yields (Table 2, entries 1, 4, 6). And the reaction of linear β-dicarbonyl compounds gave the corresponding products in fair to good yields (Table 2, entries 3, 5). It should be noted that acetophenone can’t reacted with 2-cyanobenzaldehyde under the same conditions. However, the target compound was obtained in the presence of TiCl4 (Table 2, entry 8). The result showed that Lewis acid was superior to base.19
The possible reaction mechanism for the 3-substitued isoindolinones 3 from the 2-cyanobenzaldehyde 1 is illustrated in Scheme 1. Carbonyl compounds 2 transform into enol I under the presence of CoCl2•6H2O Then enol I reacts with 1 to provide the key intermediate II. The latter carries out intramolecular nucleophilic addition to form the cyclization product III, which ring-opened to the intermediate IV. And the final product 3 was obtained through the cyclization of IV. (Dimroth rearrangement20).
All products were characterized by IR, 1H NMR, 13C NMR and ESI spectra. And the structure 3b was undoubtedly confirmed by X-ray crystallographic analysis (Figure 2).21
EXPERIMENTAL
Melting points were measured on XT4 microscope melting point apparatus (uncorrected). Infrared (IR) spectra were performed on Perkin Elmer FT-IR spectrophotometer with KBr pellets. 1H and 13C NMR spectra were performed on Bruker 400 MHz spectrometer with TMS as internal standard. Mass spectra were performed on ZAB-HS mass spectrometer using ESI ionization. Elemental analyses were performed on Elementar Vario EL. All reagents and solvents were commercially available and used without further purification.
General procedure for the synthesis of 3a-h: 2-Cyanobenzaldehyde (1, 1.0 mmol) was added to a mixture of 1,3-dicarbonyl compound (2, 1.2 mmol) and CoCl2•6H2O (0.4 mmol) in MeCN (5.0 mL). At the end of the reaction (TLC monitoring), the mixture was diluted was water and extracted with EtOAc (3×10 mL). The combined organic layers were dried with Na2SO4 and evaporated under reduced pressure. The residue was purified by column chromatography on silica gel (200-300 mesh silica gels) to afford pure 3.
3-(2-Hydroxy-4,4-dimethyl-6-oxocyclohex-1-en-1-yl)isoindolin-1-one (3a): White solid; mp 234-237 °C; Rf = 0.33 (EtOAc : HCOOH = 60 : 1); 1H NMR (400 MHz, DMSO-d6) (δ, ppm): 8.31 (s,1H), 8.13 (s, 1H), 7.59-7.57 (d, J = 7.6 Hz, 1H), 7.48-7.44 (t, J = 8.4 Hz, 1H), 7.39-7.35 (t, J =7.2 Hz, 1H), 7.18-7.16 (d, J = 7.6 Hz, 1H), 5.75 (s, 1H), 2.22 (s, 4H), 1.00 (s, 6H); 13C NMR (100 MHz, DMSO-d6) (δ, ppm): 170.0, 163.0, 148.5, 133.3, 131.0, 131.0, 126.8, 122.2, 121.7, 109.6, 56.1, 50.0, 50.0, 31.7, 27.8, 27.8; IR (KBr, v, cm-1): 3210, 2966, 2902, 2569, 1724, 1663, 1563, 1384, 1285, 1032, 679; ESI-MS (m/z) = 294 ([M+Na]+); Anal. Calcd for C16H17NO3: C, 70.83; H, 6.32; N, 5.16%. Found: C, 71.01; H, 6.22; N, 5.09%.
Dimethyl 2-(3-oxoisoindolin-1-yl)malonate (3b):22 White solid; mp 154-156 °C; Rf = 0.29 (EtOAc : petroleum ether = 1 : 1); 1H NMR (400 MHz, CDCl3) (δ, ppm): 7.88-7.86 (d, J = 8 Hz, 1H), 7.59-7.50 (m, 2H), 7.34-7.32 (d, J = 7.2 Hz, 1H), 6.81 (s, 1H), 5.20-5.18 (d, J = 8 Hz, 1H), 3.87 (s, 3H), 3.70 (s, 3H), 3.63-3.61 (d, J = 8 Hz, 1H); 13C NMR (100 MHz, CDCl3) (δ, ppm): 170.0, 167.7, 167.2, 143.7, 132.2, 132.1, 129.2, 124.2, 123.0, 56.1, 54.9, 53.2, 53.1; IR (KBr, v, cm-1): 3183, 3083, 1744, 1701, 1435, 1267, 1144, 965, 769, 751; ESI-MS (m/z) = 286 ([M+Na]+).
3-(3-Oxoisoindolin-1-yl)pentane-2,4-dione (3c):22 White solid; mp 152-153 °C; Rf = 0.33 (EtOAc : petroleum ether = 1 : 1); 1H NMR (400 MHz, CDCl3) (δ, ppm): 7.87-7.86 (d, J = 7.2 Hz, 1H), 7.56-7.51 (m, 2H), 7.31-7.29 (d, J = 8 Hz, 1H), 6.85 (s, 1H), 5.26-5.24 (d, J = 6.8 Hz, 1H), 4.04-4.02 (d, J = 6.8 Hz, 1H), 2.33 (s, 3H), 2.08 (s, 3H); 13C NMR (100 MHz, CDCl3) (δ, ppm): 202.3, 201.1, 170.1, 144.3, 132.4, 132.1, 129.2, 124.5, 122.9, 71.3, 55.0, 31.6, 30.8; IR (KBr, v, cm-1): 3188, 3079, 2914, 2871, 1718, 1416, 1359, 1318, 1275, 1148, 747, 686; ESI-MS (m/z) = 230 ([M-H]-).
3-(2-Hydroxy-6-oxocyclohex-1-en-1-yl)isoindolin-1-one (3d):22 White solid; mp 271-274 °C. Rf = 0.25 (EtOAc : HCOOH = 60 : 1); 1H NMR (400 MHz, DMSO-d6) (δ, ppm): 10.87 (s, 1H), 8.25 (s, 1H), 7.58-7.56 (d, J = 8 Hz, 1H), 7.47-7.44 (t, J = 7.2 Hz, 1H), 7.38-7.35 (t, J = 7.2 Hz, 1H), 7.20-7.18 (d, J = 7.6 Hz, 1H), 5.75 (s, 1H), 2.32 (s, 2H), 1.86-1.84 (d, J = 6 Hz, 2H); 13C NMR (100 MHz, DMSO-d6) (δ, ppm): 194, 170.0, 145.5, 133.1, 130.9, 128.8, 122.2, 121.9, 111.0, 50.0, 30.3, 20.2; IR (KBr, v, cm-1): 3287, 2932, 1646, 1630, 1387, 1302, 996, 684; ESI-MS (m/z) = 242 ([M-H]-).
Ethyl 3-oxo-2-(3-oxoisoindolin-1-yl)butanoate (3e):22 White solid; mp 210-220 °C; Rf = 0.37 (EtOAc : petroleum ether = 2 : 1); 1H NMR (400 MHz, CDCl3) (δ, ppm): 7.85-7.83 (d, J = 8 Hz, 1H), 7.56-7.48 (m, 2H), 7.31-7.29 (d, J = 7.6 Hz, 1H), 6.75 (s, 1H), 5.24-5.23 (d, J = 7.2 Hz, 1H), 4.15-4.10 (q, J = 7.2 Hz, 2H), 3.81-3.79 (d, J = 8 Hz, 1H), 2.35 (s, 3H), 1.15-1.11 (t, J = 7.2 Hz, 3H); 13C NMR (100 MHz, CDCl3) (δ, ppm): 201.7, 169.9, 166.6, 144.3, 132.1, 129.0, 129.0, 128.9, 124.1, 123.0, 62.9, 62.3, 54.5, 31.2, 13.9; IR (KBr, v, cm-1): 3226, 3083, 2988, 2938, 1731, 1717, 1697, 1473, 1339, 1255, 1200, 1107, 1025, 754, 702; ESI-MS (m/z) = 284 ([M+Na]+).
3-(2-Hydroxy-5-oxocyclopent-1-en-1-yl)isoindolin-1-one (3f): Sepia solid; mp 202-204 °C; Rf = 0.22 (EtOAc : HCOOH = 60 : 1); 1H NMR (400 MHz, DMSO-d6) (δ, ppm): 8.27 (s, 1H), 7.58-7.56 (d, J = 7.6 Hz, 1H), 7.47-7.43 (t, J = 7.2 Hz, 1H), 7.39-7.35 (t, J = 7.2 Hz, 1H), 7.22-7.20 (d, J = 7.6 Hz, 1H), 5.32 (s, 1H), 2.26-2.19 (dt, J = 13.6 Hz, 4H); 13C NMR (100 MHz, DMSO-d6) (δ, ppm): 201.1, 199.35, 174.89, 138.19, 136.23, 132.26, 127.88, 127.83, 127.46, 103.9, 55.35, 36.14, 36.14; IR (KBr, v, cm-1): 3246, 2918, 1677, 1558, 1468, 1395, 1287, 1022, 772; ESI-MS (m/z) = 252 ([M+Na]+); Anal. Calcd for C13H11NO3: C, 68.11; H, 4.84; N, 6.11%. Found: C, 68.30; H, 4.79; N, 5.99%.
Diethyl 2-(3-oxoisoindolin-1-yl)malonate (3g):15 White solid; mp 94-96 °C; Rf = 0.32 (EtOAc : petroleum ether = 1 : 1); 1H NMR (400 MHz, CDCl3) (δ, ppm) (δ, ppm): 7.88-7.86 (d, J = 6.8 Hz, 1H), 7.59-7.50 (m, 2H), 7.39-7.37 (d, J = 7.2 Hz, 1H), 6.79 (s, 1H), 5.17-5.16 (d, J = 7.2 Hz, 1H), 4.33-4.27 (q, J =7.2 Hz, 2H), 4.15-4.09 (q, J =7.2 Hz, 2H), 3.65-3.63 (d, J = 7.6 Hz, 1H), 1.34-1.31 (t, J = 7.2 Hz, 3H), 1.14-1.11 (t, J = 7.2 Hz, 3H); 13C NMR (100 MHz, CDCl3) (δ, ppm): 167.5, 167.5, 166.7, 146.1, 132.2, 129.1, 129.1, 124.2, 123.1, 62.3, 62.3, 56.2, 54.9, 14.2, 13.9; IR (KBr, v, cm-1): 3199, 3086, 2976, 2870, 1743, 1720, 1698, 1471, 1346, 1179, 1158, 1040, 747; ESI-MS (m/z) = 314 ([M+Na]+).
3-Benzoylisoindolin-1-one (3h):19 White solid; mp 138-140 °C; Rf = 0.22 (EtOAc : petroleum ether = 1 : 8); 1H NMR (400 MHz, CDCl3) (δ, ppm): 7.97-7.92 (m, 4H), 7.68-7.47 (m, 6H), 6.20-6.17 (t, J = 7.2 Hz, 1H), 3.82-3.76 (d, J = 7.6 Hz, 1H), 3.43-3.37 (d, J = 7.2 Hz, 1H); 13C NMR (100 MHz, CDCl3) (δ, ppm): 196.3, 170.5, 156.4, 134.6, 134.2, 129.7, 129.7, 129.1, 129.1, 128.5, 128.5, 126.1, 126.0, 123.1, 77.0, 44.0; IR (KBr, v, cm-1): 3064, 2912, 1769, 1681, 1596, 1447, 1291, 1080, 755; ESI-MS (m/z) = 274 ([M+Na]+).
References
1. X. N. Yang, Y. S. Jin, P. Zhu, and H. S. Chen, Chem. Nat. Compd., 2010, 46, 324; CrossRef J. Selvakumar and R. C. Ramanathan, Org. Biomol. Chem., 2011, 9, 7643. CrossRef
2. I. K. Lee, S. E. Kim, J. H. Yeom, D. W. Ki, M. S. Lee, J. G. Song, Y. S. Kim, S. J. Seok, and B. S. Yun, J. Antibiot., 2012, 65, 95. CrossRef
3. I. Takahashi, T. Kawakami, E. Hirano, H. Yokota, and H. Kitajima, Synlett, 1996, 353. CrossRef
4. L. Q. Fu, C. Y. Ling, X. S. Guo, H. L. He, and Y. S. Yang, Chin. Chem. Lett., 2012, 3, 9. CrossRef
5. I. R. Hardcastle, J. Liu, E. Valeur, A. Watson, S. U. Ahmed, T. J. Blackburn, K. Bennaceur, W. Clegg, C. Drummond, J. A. Endicott, B. T. Golding, R. J. Griffin, J. Gruber, K. Haggerty, R. W. Harrington, C. Hutton, S. Kemp, X. H. Lu, J. M. McDonnell, D. R. Newell, M. E. M. Noble, S. L. Payne, C. H. Revill, C. Riedinger, Q. Xu, and J. Lunec, J. Med. Chem., 2011, 54, 1233; CrossRef M. L. Curtin, R. R. Frey, H. R. Heyman, K. A. Sarris, D. H. Steinman, J. H. Holmes, P. F. Bousquet, G. A. Cunha, M. D. Moskey, A. A. Ahmed, L. J. Pease, K. B. Glaser, K. D. Stewart, S. K. Davidsen, and M. R. Michaelides, Bioorg. Med. Chem. Lett., 2004, 14, 4505. CrossRef
6. M. S. Egbertson, G. D. Hartman, R. J. Gould, B. Bednar, R. A. Bednar, J. J. Cook, S. L. Gaul, M. A. Holahan, L. A. Libby, J. J. Lynch, R. J. Lynch, G. R. Sitko, M. T. Stranieri, and L. M. Vassallo, Bioorg. Med. Chem. Lett., 1996, 6, 2519. CrossRef
7. S. Ito, Y. Hirata, Y. Nagatomi, A. Satoh, G. Suzuki, T. Kimura, A. Satow, S. Maehara, H. Hikichi, M. Hata, H. Ohta, and H. Kawamoto, Bioorg. Med. Chem. Lett., 2009, 19, 5310. CrossRef
8. S. Sidique, R. P. Dhanya, D. J. Sheffler, H. H. Nickols, Y. Li, R. Dahl, M. N. Arianna, L. H. Smith, M. S. D’Souza, S. Semenova, P. J. Conn, A. Markou, and N. D. P. Cosford, J. Med. Chem., 2012, 55, 9434; CrossRef M. S. D’Souza and A. Markou, Addict. Sci. Clin. Pract., 2011, 6, 4.
9. M. Angelin, M. Rahm, A. Fischer, T. Brinck, and O. Ramström, J. Org. Chem., 2010, 75, 5882; CrossRef M. Angelin, P. Vongvilai, A. Fischer, and O. Ramström, Chem. Commun., 2008, 6, 768. CrossRef
10. K. Kobayashi, K. Matsumoto, and H. Konishi, Heterocycles, 2011, 83, 99. CrossRef
11. Y. S. Song, C. H. Lee, and K. J. Lee, J. Heterocycl. Chem., 2003, 40, 939; CrossRef S. L. Xu, L. Y. Wang, Y. H. Tang, and Z. J. He, Synthesis, 2014, 46, 2085. CrossRef
12. R. Sato, M. Ohmori, F. Kaitani, A. Kurosawa, T. Senzaki, T. Goto, and M. Saito, Bull. Chem. Soc. Jpn., 1988, 61, 2481. CrossRef
13. R. Sato, T. Senzaki, T. Goto, and M. Saito, Chem. Lett., 1984, 1599. CrossRef
14. M. W. Khan and A. F. G. M. Reza, Tetrahedron, 2005, 61, 11204; CrossRef R. Grigg, L. X. Zhang, S. Collard, and A. Keep, Tetrahedron Lett., 2003, 44, 6979. CrossRef
15. C. Petronzi, S. Collarile, G. Croce, R. Filosa, P. D. Caprariis, A. Peduto, L. Palombi, V. Intintoli, A. D. Mola, and A. Massa, Eur. J. Org. Chem., 2012, 27, 5357. CrossRef
16. A. D. Mola, L. Palombi, and A. Massa, Curr. Org. Chem., 2012, 16, 2302; CrossRef P. Antico, V. Capaccio, A. D. Mola, A. Massa, and L. Palombi, Adv. Synth. Catal., 2012, 354, 1717. CrossRef
17. V. More, R,Rohlmann, O. G. Mancheno, C. Petronzi, L. Palombi, A. D. Rosa, A. D. Mola, and A. Massa, RSC Adv., 2012, 2, 3592; CrossRef A. Massa, P. Rizzo, G. Monaco, and R. Zanasi, Tetrahedron Lett., 2013, 54, 6242; CrossRef S. Tiso, L. Palombi, C. Vigness, A. D. Mola, and A. Massa, RSC Adv., 2013, 3, 19380.
18. L. J. Zhang, D. X. Shi, and J. R. Li, Synth. Commun., 2009, 39, 4010; CrossRef J. R. Li, L. J. Zhang, J. N. Chen, D. X. Shi, and Q. Zhang, J. B. Inst. Techno., 2007, 27, 25; J. R. Li, L. J. Zhang, D. X. Shi, D. Wang, C. X. Wang, Q. Zhang, L. Zhang, and Y. Q. Fan, Synlett, 2008, 233. CrossRef
19. N. Rao, E. N. Prabhakaran, S. K. Das, and J. Iqbal, J. Org. Chem., 2003, 68, 4079. CrossRef
20. O. Dimroth, Ann., 1909, 364, 183; D. J. Brown and J. S. Harper, J. Chem. Soc., 1963, 239, 1276. CrossRef
21. Full details have been deposited the Cambridge Crystallographic Data Centre as supplementary publication no. CCDC-975103. Copies of the data can be obtained, free of charge, on application to CCDC, 12 Union Road, Cambridge CB2 1EZ, UK (fax: +44(0)-1223336033 or e-mail: deposit@ccdc.cam.ac.uk).
22. V. More, A. D. Mola, M. Perillo, P. D. Caprariis, R. Filosa, A. Peduto, and A. Massa, Synthesis, 2011, 3027. CrossRef