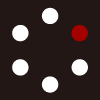
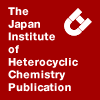
HETEROCYCLES
An International Journal for Reviews and Communications in Heterocyclic ChemistryWeb Edition ISSN: 1881-0942
Published online by The Japan Institute of Heterocyclic Chemistry
e-Journal
Full Text HTML
Received, 12th May, 2015, Accepted, 28th May, 2015, Published online, 10th June, 2015.
DOI: 10.3987/COM-15-13248
■ Ga(III) Catalyzed Addition of Indole Derivatives and Different Propiolates
Xun Zhu,* Xiuqin Zhou, and Dongsheng Xiang
Department of Chemistry, Yancheng Institute of Industry Technology, Yancheng, Jiangsu, 224005, China
Abstract
Ga(III) was used to catalyze Michael addition of indole derivatives to different propiolates in aqueous solution. Good to excellent yields were obtained under our reaction conditions.As an unique heterocyclic organic compound, indole and its derivatives have attached increasing attentions in medicinal and organic chemistry due to its important biological, pharmaceutical and therapeutic activities, such as intercellular signals, fluorescent dyes, drugs, essential oils, etc.1-10 The bis-indole derivatives, which were often isolated from terrestrial and marine sources, were one of the most important structures with a wide range of biological activities, such as genotoxicity,11,12 antibacterial activity,13,14 coronary dilatory property,15 and so on.16-19
Compared with the versatile methodologies for indole synthesis,20-26 only a few papers were reported on the synthesis of bis-indole derivatives via the direct addition of indole to alkenes or alkynes.27-31 In 2005, the bis-indole product was reported by AuCl3 catalyzed Michael addition of N-methylindole with ethyl propiolate in acetonitrile solution with a yield of 74%.28 The same reaction was also investigated by using PtCl2 as the catalyst in refluxing THF solution, but as a minor product.29 An optimized reaction condition was reported in 2011 by using combined catalysts of FeCl3 and AgOTf in acetic acid solution.30 Three indole-related compounds, indole, N-methylindole and 2-methylindole, were reacted with methyl, ethyl propiolate or propiolic acid to produce both mono- and bis-indole products by controlling the reaction time. However, expensive precious metals, such as gold, platinum and silver, were used as catalysts in the reported papers, which might restrict their practical applications. As a continuous interest,32-35 we reported a new catalyst, gallium(III) dodecyl sulfate, Ga(DS)3, and its application to catalyze the addition of indole derivatives to different propiolates. Compared with precious metal catalysts, Ga(DS)3 has much lower price and its catalyzed reactions can be carried out in aqueous solution. Moreover, the scope of indole derivatives at all seven positions were considered, giving moderate to excellent yields.
Initially, by exploring the reaction condition, indole and ethyl propiolate were catalyzed by different gallium(III) catalysts in various solutions, as collected in Table 1. Ga(OTf)3 was firstly employed to catalyze this reaction in acetonitrile at room temperature (rt). A messy product distribution was observed within 5 min as monitored by the TLC plates. By extending the reaction time to 12 h, neither at room temperature nor refluxing could improve the product distribution (Table 1, entries 1 and 2). Other solvents were also considered, such as dichloromethane (DCM), water, toluene and 1,2-dichloroethane (DCE). The product distributions were either messy or no reaction occurred (Table 1, entries 3-6). Considering the strong Lewis acidity of Ga(OTf)3, a milder Lewis acid, GaI3, was used but still resulted in a messy product distribution (Table 1, entry 7).
However, when switched to Ga(DS)3, the desired product was obtained by stirring at room temperature for 96 h in aqueous solution with 90% yield (Table 1, entry 8). An increase in temperature to 70 oC can both reduce the reaction time to 18 h and increase the yield to 99% (Table 1, entry 9). The reaction yield remained the same when the catalyst amount was reduced to 5 mol% (Table 1, entry 10). To further corroborate the catalytic activity of Ga(DS)3, sodium dodecyl sulfate (SDS) was used as a catalyst with a 63% yield (Table 1, entry 11), which had no further improvement with either increased reaction temperature or longer reaction time. Therefore, our optimized reaction condition was revealed by using 5 mol% Ga(DS)3 as the catalyst in aqueous solution at 70 oC.
With the best reaction condition (Table 1, entry 10) in hand, the reaction scope was explored to indole derivatives and different propiolates, as collected in Table 2. Firstly, indole substituents at seven different positions were reacted with ethyl propiolates to investigate the substituent effects on indole (Table 2, entries 2-10). Low yields were obtained for N-methyl- and 4-methylindole under either the optimized condition or at elevated temperature of 110 oC (Table 2, entries 2 and 5), which might due to the lack of hydrogen bondings with water for N-methylindole and the steric effect for 4-methylindole, respectively. The reaction of 2-methyl- and 5-methylindole proceeded smoothly under the optimized condition, giving excellent yields of 94% and 98%, respectively (Table 2, entries 3 and 6). However, for 3-methyl-, 7-methyl- and 5-bromoindole although the optimized condition gave low yields, they can be improved by increasing the reaction temperature to 110 oC (Table 2, entries 4, 8 and 9). On the contrary, an increased yield was observed for 6-methylindole at lower reaction temperature (Table 2, entry 7). Disubstituted 5,7-dibromoindole was also considered, giving a low yield under the optimized condition, and a good yield at an elevated temperature (Table 2, entry 10). After investigating the substituent effects of indole derivatives, different propiolates were also considered. The reaction of indole and 2-methylindole with methyl propiolate gave the desired products with excellent yields (Table 2, entries 11 and 12). A mono-indole addition product was obtained for the reaction of dimethyl but-2-ynedioate with indole with a low yield (Table 2, entry 13).
In conclusion, a new catalyst, Ga(DS)3, was synthesized and employed to catalyze the Michael addition of indole derivatives to different propiolates in aqueous solution. This environmental benign condition, as well as high yield, will promote this reaction into wide applications.
EXPERIMENTAL
All chemicals (AR grade) were obtained from commercial resources and used without further purification. 1H and 13C NMR spectroscopy was performed using a Bruker Ultrashield 400 MHz (100 MHz for 13C NMR) instrument. Fourier transform infrared spectra (FT-IR) were collected on a Nicolet 6700 spectrometer and analyzed with OMNIC32 software. The melting point was measured using XT-4 apparatus. High-resolution mass spectra (HRMS) were obtained using a GCT-TOF instrument.
Synthesis of gallium(III) dodecyl sulfate
Gallium(III) oxide (187 mg, 1.0 mmol) was added into excess HCl(conc.) solution. This solution was refluxed until all solid were dissolved. Water was added and evaporated repeatedly until excess HCl was removed. After cooling, a solution of sodium dodecyl sulfate (1.44 g, 5.0 mmol) in 50 mL water was added into the solution with the formation of white precipitates. This solution was stirred for another 20 min before filtration to obtained the desired product, which was dried over vacuum for 48 h.
General procedure for the reaction of indole derivatives and propiolates
To a mixture of Ga(DS)3 (0.05 mmol), indole derivative (1.0 mmol) and propiolate (1.0 mmol) in 10 mL water. The mixture was stirred at desired temperature for an appropriate time. After cooling to rt, brine was added and the product was extracted by EtOAc. The organic layer was separated, dried over anhydrous Na2SO4 and evaporated. The residue was purified by column chromatography, using acetone/petroleum ether as the eluent.
Characterizations for part of the compounds:
Gallium(III) dodecyl sulfate. mp 62–63 oC. 1H NMR (acetone-d6, 400 MHz) δ 4.15–4.00 (m, 6H, 3CH2), 1.74–1.55 (m, 6H, 3CH2), 1.48–1.02 (m, 54H, 27CH2), 0.97–0.71 (m, 9H, 3CH3); 13C NMR (acetone-d6, 100 MHz) δ 69.6, 32.6, 30.4, 30.2, 30.1, 29.3, 26.5, 23.3, 14.2; FT-IR: 1252, 1243. Anal. Calcd for (C36H75GaO12S3): C, 49.94; H, 8.73; S, 11.11; Ga, 8.05%. Found: C, 49.89; H, 8.87; S, 11.08; Ga, 7.91%.
Ethyl 3,3-bis(3-methyl-1H-indol-2-yl)propanoate (3d). mp 159–160 oC. 1H NMR (CDCl3, 400 MHz) δ 8.64 (br, 2H, 2NH), 7.57 (d, 2H, J = 7.5 Hz, ArH), 7.31 (d, 2H, J = 7.8 Hz, ArH), 7.24–7.11 (m, 4H, ArH), 5.12 (t, 1H, J = 6.5 Hz, CH), 4.11 (q, 2H, J = 7.0 Hz, CH2), 3.20 (d, 2H, J = 6.5 Hz, CH2), 2.33 (s, 6H, 2CH3), 1.13 (t, 3H, J = 7.0 Hz, CH3); 13C NMR (CDCl3, 100 MHz) δ 173.5, 135.7, 135.5, 129.8, 122.1, 119.9, 119.0, 111.5, 108.3, 61.9, 39.3, 31.8, 14.4, 9.2; HR-MS(EI): calcd for C23H24N2O2: 360.1838, found: 360.1835.
Ethyl 3,3-bis(4-methyl-1H-indol-3-yl)propanoate (3e). mp 85–87 oC. 1H NMR (CDCl3, 400 MHz) δ 7.90 (br, 2H, 2NH), 7.20–7.06 (m, 4H, ArH), 6.88 (d, 2H, J = 6.8 Hz, ArH), 6.72 (s, 2H, ArH), 5.72 (t, 1H, J = 7.5 Hz, CH), 4.11 (q, 2H, J = 7.0 Hz, CH2), 3.08 (d, 2H, J = 7.5 Hz, CH2), 2.70 (s, 6H, 2CH3), 1.19 (t, 3H, J = 7.0 Hz, CH3); 13C NMR (CDCl3, 100 MHz) δ 172.9, 137.6, 131.2, 125.3, 122.7, 122.3, 121.5, 120.2, 109.5, 60.9, 44.3, 33.0, 20.7, 14.4; HR-MS(EI): calcd for C23H24N2O2: 360.1838, found: 360.1836.
Ethyl 3,3-bis(5-methyl-1H-indol-3-yl)propanoate (3f). mp 44–45 oC. 1H NMR (CDCl3, 400 MHz) δ 7.80 (br, 2H, 2NH), 7.50 (s, 2H, ArH), 7.20 (d, 2H, J = 8.4 Hz, ArH), 7.08 (d, 2H, J = 8.4 Hz, ArH), 6.82 (s, 2H, ArH), 5.17 (t, 1H, J = 7.6 Hz, CH), 4.15 (q, 2H, J = 7.0 Hz, CH2), 3.26 (d, 2H, J = 7.6 Hz, CH2), 2.50 (s, 6H, 2CH3), 1.20 (t, 3H, J = 7.0 Hz, CH3); 13C NMR (CDCl3, 100 MHz) δ 173.5, 135.5, 128.9, 127.4, 124.0, 122.5, 119.7, 118.5, 111.5, 60.9, 41.8, 31.3, 22.1, 14.5; HR-MS(EI): calcd for C23H24N2O2: 360.1838, found: 360.1835.
Ethyl 3,3-bis(6-methyl-1H-indol-3-yl)propanoate (3g). mp 42–45 oC. 1H NMR (CDCl3, 400 MHz) δ 7.80 (br, 2H, 2NH), 7.49 (d, 2H, J = 8.2 Hz, ArH), 7.10 (s, 2H, ArH), 6.91 (d, 2H, J = 8.2 Hz, ArH), 6.86 (s, 2H, ArH), 5.09 (t, 1H, J = 7.8 Hz, CH), 4.08 (q, 2H, J = 7.2 Hz, CH2), 3.19 (d, 2H, J = 7.8 Hz, CH2), 2.45 (s, 6H, 2CH3), 1.15 (t, 3H, J = 7.2 Hz, CH3); 13C NMR (CDCl3, 100 MHz) δ 173.2, 137.5, 132.0, 125.0, 121.9, 121.5, 119.7, 119.0, 111.6, 60.9, 41.7, 31.3, 22.2, 14.5; HR-MS(EI): calcd for C23H24N2O2: 360.1838, found: 360.1837.
Ethyl 3,3-bis(7-methyl-1H-indol-3-yl)propanoate (3h). mp 52–54 oC. 1H NMR (CDCl3, 400 MHz) δ 7.83 (br, 2H, 2NH), 7.53 (d, 2H, J = 7.2 Hz, ArH), 7.14–6.99 (m, 4H, ArH), 6.80 (s, 2H, ArH), 5.19 (t, 1H, J = 7.6 Hz, CH), 4.10 (q, 2H, J = 7.0 Hz, CH2), 3.25 (d, 2H, J = 7.6 Hz, CH2), 2.45 (s, 6H, 2CH3), 1.20 (t, 3H, J = 7.0 Hz, CH3); 13C NMR (CDCl3, 100 MHz) δ 173.2, 136.5, 126.6, 122.8, 121.9, 120.9, 119.8, 119.5, 117.6, 60.8, 41.6, 31.4, 17.0, 14.5; HR-MS(EI): calcd for C23H24N2O2: 360.1838, found: 360.1837.
Ethyl 3,3-bis(5-bromo-1H-indol-3-yl)propanoate (3i). mp 62–64 oC. 1H NMR (CDCl3, 400 MHz) δ 8.20 (br, 2H, 2NH), 7.66 (s, 2H, ArH), 7.20–7.17 (m, 2H, ArH), 7.10 (d, 2H, J = 8.6 Hz, ArH), 6.90 (s, 2H, ArH), 4.99 (t, 1H, J = 7.6 Hz, CH), 4.08 (q, 2H, J = 7.1 Hz, CH2), 3.16 (d, 2H, J = 7.6 Hz, CH2), 1.16 (t, 3H, J = 7.1 Hz, CH3); 13C NMR (CDCl3, 100 MHz) δ 173.1, 135.7, 128.6, 125.6, 123.5, 122.1, 118.1, 113.2, 113.0, 61.3, 41.5, 31.2, 14.6; HR-MS(EI): calcd for C21H18Br2N2O2: 487.9735, found: 487.9736.
Ethyl 3,3-bis(5,7-dibromo-1H-indol-3-yl)propanoate (3j). mp 83–85 oC. 1H NMR (CDCl3, 400 MHz) δ 8.24 (br, 2H, 2NH), 7.55 (s, 2H, ArH), 7.44 (s, 2H, ArH), 7.10 (s, 2H, ArH), 4.93 (t, 1H, J = 7.6 Hz, CH), 4.07 (q, 2H, J = 7.1 Hz, CH2), 3.14 (d, 2H, J = 7.6 Hz, CH2), 1.15 (t, 3H, J = 7.1 Hz, CH3); 13C NMR (CDCl3, 100 MHz) δ 172.1, 134.5, 129.1, 127.4, 123.7, 121.5, 119.0, 113.0, 106.0, 61.3, 41.2, 31.2, 14.6; HR-MS(EI): calcd for C21H16Br4N2O2: 643.7945, found: 643.7950.
Dimethyl 2-(1H-indol-3-yl)maleate (3m). yellow liquid. 1H NMR (CDCl3, 400 MHz) δ 8.72 (br, 1H, NH), 7.32–7.25 (m, 2H, ArH), 7.17–7.07 (m, 3H, ArH), 6.90 (s, 1H, =CH), 3.80 (s, 3H, CH3), 3.64 (s, 3H, CH3); 13C NMR (CDCl3, 100 MHz) δ 168.4, 166.8, 138.3, 135.9, 128.2, 126.5, 124.7, 122.5, 120.7, 119.6, 112.0, 108.8, 53.2, 52.2; HR-MS(EI): calcd for C14H13NO4: 259.0845, found: 259.0843.
The structures of compound 3a, 3b, 3c, 3k and 3l were identified with the reported literature.30
ACKNOWLEDGEMENTS
This work is financially supported by Jiangsu Provincial Natural Foundation of China (NO. BK20141260).
References
1. J. W. Huffman and L. W. Padgett, Curr. Med. Chem., 2005, 12, 1395. CrossRef
2. S. Agarwal, S. Cammerer, S. Filali, W. Frohner, J. Knoll, M. P. Krahl, K. R. Reddy, and H.-J. Knölker, Curr. Org. Chem., 2005, 9, 1601. CrossRef
3. T. C. Barden, Indoles: Industrial, Agricultural and Over-the-Counter Uses. In Heterocyclic Scaffolds II: Topics in Heterocyclic Chemistry, ed. by G. W. Gribble, Springer Berlin Heidelberg: 2010; Vol. 26, p. 31. CrossRef
4. T. Wang, K. T. Schlueter, B. L. Riehl, J. M. Johnson, and W. R. Heineman, Anal. Chem., 2013, 85, 9486. CrossRef
5. S. Patil and J. K. Buolamwini, Curr. Org. Synth., 2006, 3, 477. CrossRef
6. Y. Huang, L. Taylor, X. Chen, and N. Ayres, J. Polym. Sci., Part A: Polym. Chem., 2013, 51, 5230. CrossRef
7. S. Zhang, J. Li, X. Wang, Y. Huang, M. Zeng, and J. Xu, J. Mater. Chem. A, 2015, 3, 10119. CrossRef
8. K. Hemalatha, G. Madhumitha, and S. M. Roopan, Chem. Rev. Sci. Lett., 2013, 2, 287.
9. T. Wang, D. Zhao, X. Guo, J. Correa, B. L. Riehl, and W. R. Heineman, Anal. Chem., 2014, 86, 4354. CrossRef
10. Y.-S. Huang and G.-W. Wang, J. Mol. Struct.: THEOCHEM, 2008, 860, 24. CrossRef
11. M. Zhang, N. T. Alvarez, D. Zhao, L. Zhang, M. R. Haase, R. Malik, C. Katuscak, T. Wang, and V. Shanov, Nano LIFE, 2014, 4, 1441019. CrossRef
12. Y. Huang and X. Chen, Nano LIFE, 2014, 4, 1441006. CrossRef
13. D. Zhao, X. Guo, T. Wang, N. Alvarez, V. N. Shanov, and W. R. Heineman, Electroanalysis, 2014, 26, 488. CrossRef
14. P. Wu, X. Chen, J. Li, and Y. Huang, Comput. Theor. Chem., 2014, 1030, 67. CrossRef
15. D. Zhao, X. Guo, T. Wang, Y. Zheng, L. Wang, and Q. Yang, Nano LIFE, 2014, 4, 1441008. CrossRef
16. T. Wang, H. D. Manamperi, W. Yue, B. L. Riehl, B. D. Riehl, J. M. Johnson, and W. R. Heineman, Electroanalysis, 2013, 25, 983. CrossRef
17. Y. Huang, J. Li, X. Chen, and X. Wang, RSC Adv., 2014, 4, 62160. CrossRef
18. J. Barluenga, A. Fernández, F. Rodríguez, and F. J. Fañanás, J. Organomet. Chem., 2009, 694, 546. CrossRef
19. Y. Huang, M. A. Shaw, E. S. Mullins, T. L. Kirley, and N. Ayres, Biomacromolecules, 2014, 15, 4455. CrossRef
20. G. Bartoli, R. Dalpozzo, and M. Nardi, Chem. Soc. Rev., 2014, 43, 4728. CrossRef
21. B. Robinson, Chem. Rev., 1963, 63, 373. CrossRef
22. D. F. Taber and P. K. Tirunahari, Tetrahedron, 2011, 67, 7195. CrossRef
23. M. Inman and C. J. Moody, Chem. Sci., 2013, 4, 29. CrossRef
24. T. Guo, F. Huang, L. Yu, and Z. Yu, Tetrahedron Lett., 2015, 56, 296. CrossRef
25. R. Vicente, Org. Biomol. Chem., 2011, 9, 6469. CrossRef
26. Y. Jin, A. Zhang, Y. Huang, and W. Zhang, Chem. Commun., 2010, 46, 8258. CrossRef
27. J. Dash, R. Nath Das, N. Hegde, G. D. Pantoş, P. S. Shirude, and S. Balasubramanian, Chem. Eur. J., 2012, 18, 554. CrossRef
28. Z. Li, Z. Shi, and C. He, J. Organomet. Chem., 2005, 690, 5049. CrossRef
29. X. Li, J.-Y. Wang, W. Yu, and L.-M. Wu, Tetrahedron, 2009, 65, 1140. CrossRef
30. M. S. Kutubi and T. Kitamura, Tetrahedron, 2011, 67, 8140. CrossRef
31. H. Singh and K. Singh, Tetrahedron, 1988, 44, 5897. CrossRef
32. X. Zhu and Y. Wei, J. Chem. Res., 2012, 36, 363. CrossRef
33. X. Zhu and Y. Wei, J. Chem. Res., 2013, 37, 119. CrossRef
34. X. Zhu, D. S. Xiang, and W. Y. Shi, J. Struct. Chem., 2013, 54, 1107. CrossRef
35. X. Zhu, W. Ge, and Y. Wei, Polycyclic Aromat. Compd., 2013, 33, 467. CrossRef