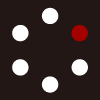
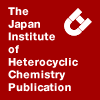
HETEROCYCLES
An International Journal for Reviews and Communications in Heterocyclic ChemistryWeb Edition ISSN: 1881-0942
Published online by The Japan Institute of Heterocyclic Chemistry
e-Journal
Full Text HTML
Received, 24th May, 2015, Accepted, 18th August, 2015, Published online, 24th August, 2015.
■ Metal-Free Synthesis of Benzothiazoles from Disulfides of 2-Aminobenzenethiol and Carboxylic Acid via PCl3-Promoted Tandem Reaction
Guangyan Du, Ning Zhu,* Limin Han, Hailong Hong, and Quanling Suo
Chemical Engineering College, Inner Mongolia University of Technology, Hohhot 010051, China
Abstract
A metal-free process for the synthesis of benzothiazoles via PCl3-promoted cleavage/acylation/ cyclization of disulfides and carboxylic acids has been developed. In addition to acting as the acylating reagent which converted carboxylic acids into acyl chlorides, PCl3 also converted disulfides to thiols, which promoted disulfides of 2-aminobenzenethiol reacted with carboxylic acid to produce benzothiazoles. The developed method is applicable to a wide range of carboxylic acids containing different functional groups.INTORODUCTION
Substituted benzothiazoles are an important class of heterocycles that exhibit valuable biological and therapeutic effects like antitumor, antimicrobial, antidiabetic, anticonvulsant, anti-inflammatory activities.1 Moreover, they can be used as amyloid imaging agent.1b For example, zopolrestat1c (1) has been used for the treatment of diabetes, Phortress (2) and PMX610 (3) display excellent antitumor activity,1a and 18F-labelled 2-(4’-fluorophenyl)-1,3-benzothiazole (4) can be used as amyloid imaging agent (Figure 1).1b In addition, some benzothiazole-based compounds are useful as ratiometric fluorescent pH indicators.2 Therefore, efficient methods to synthesize benzothiazoles and their structural analogues have been explored extensively in recent years.3
Among the many existing methods for the construction of benzothiazoles, the condensations of 2-aminobenzenethiols with aldehydes, carboxylic acids, orthoesters, alkylamines, aryl ketones, β-diketones or nitriles are the most common procedure (Scheme 1a).3a-e,4 However, the starting materials 2-aminobenzenethiols, especially those bearing additional substituents, are readily oxidized to stable disulfides and not always easily available. An alternative approach is the cyclization of thioformanilides, which is carried out by the radical cyclization or transition-metal catalyzed carbon-sulfur bond formation(Scheme 1b).3f,5
However, this method usually exhibits low tolerance for functional groups such as ketone, ester, and amide, because most of the reactions involve the transformation of the amide into the corresponding thioamide by Lawesson’s reagent.5 To overcome this drawback, methods using 2-haloanilides reacting with metal sulfides,3g,3h,6 carbon disulfide,3i,7 sulfur 3j,3k or xanthate salt 8 are developed to synthesize 2-substituted benzothiazoles through copper-catalyzed cross-coupling reaction (Scheme 1c). Recently, 2-substituted benzothiazoles were prepared by the reaction of stable disulfide and aldehyde through the cleavage of the S-S bond via radical, copper-catalyzed, reductive or oxidative method (Scheme 1d).9 In the course of our studies on new methods for the synthesis of benzothiazoles using disulfides as starting materials,10 we found that disulfides reacted with carboxylic acids smoothly to afford benzothiazoles in moderate yields in a one-pot process (Scheme 1e). To the best of our knowledge, this is the first successful example of the synthesis of substituted benzothiazoles from stable and readily available disulfides and carboxylic acids in one-pot tandem reactions.
RESULTS AND DISCUSSION
We began our study by examining the reaction of 2,2'-disulfanediyldianiline (1a) with 2-hydroxy-4-methylbenzoic acid (2a) in organic solvent using different condensing agents under various conditions. When 1a reacted with 2.0 equiv. of 2a in the absence of any condensing agents, no desired product 3a was obtained in common organic solvents such as toluene, DMF and 1,4-dioxane as determined by LC-MS analysis (Table 1, entry 1). Furthermore, no or little desired product 3a was obtained in the presence of condensing agents such as SOCl2, POCl3 or Ph3P (entries 2-4). Much to our delight, when 2.4 equiv. of PCl3 was used as the condensing agent in toluene, the anticipated product 3a was obtained in 74% isolated yield (entry 5). When the solvent toluene was replaced with other solvents like DMF or DMSO, no the desired product 3a was found (entries 6-7). The effect of solvent 1,4-dioxane was also investigated, and the products was obtained in a relatively low yield (entry 8). The reaction temperature was another important factor affecting the yield. The reaction yield decreased dramatically to 26% when carried out at 90 °C; when carried out at 110 °C, the yield decreased slightly to 69% (entries 9-10). Moreover, the reaction yield would decrease when the ratio of PCl3 to disulfide was higher or lower than 2.4 (entries 11-14), which showed the ratio of PCl3 to disulfide was also a key factor in determining the yield of desired product. Moderate yield was obtained when the reaction was carried out under an atmosphere of air (entry 15).
A variety of carboxylic acids were examined under the optimized reaction conditions to explore the scope of the substrates. As shown in Table 2, carboxylic acids containing electron-donating groups were employed to afford the corresponding benzothiazoles in moderate to good yields ranging from 54 to 74% (entries 1-8). The relative low yield of 3f and 3g showed that halides substituted group decreased the reactivity of the aromatic carboxylic acids in this one-pot reaction. Additionally, this PCl3-mediated one-pot process exhibited compatibility with several functional groups such as hydroxyl (3a and 3b), methoxyl (3c and 3h), and halides (3f and 3g). However, when carboxylic acids contained electron-withdrawing groups like nitro or nitrile, the corresponding benzothiazoles were obtained in low yields (entries 9-10). Phthalic acid and some aliphatic acids such as acetic acid, trifluoroacetic acid, butyric acid and 2-phenylacetic acid also reacted with disulfide to form the desired products, but the yields were relatively low (entries 11-15).
To expand the scope of this methodology, several different disulfides were employed to react with carboxylic acids under the optimized reaction conditions (entries 16-18). Various functional groups including chloro (3p) and phenoxyl (3q and 3r) were well tolerated under the standard reaction conditions, and the desired products were obtained in moderate to good yields. In addition, the free amino group reacted with carboxylic acid to form the amide product (3q and 3r) under the standard reaction conditions.
The methods used to cleave the sulfur-sulfur bond are the key factor for the synthesis of benzothiazoles from disulfides.4a To understand how benzothiazoles can be prepared from disulfides and carboxylic acids in the presence of PCl3, two possible reaction routes are considered which are illustrated in Scheme 2 using the reaction of 1a with 2d as an example. In route a, intermediate A is formed from disulfide 1a and benzoic acid 2d mediated by PCl3 and then the sulfur-sulfur bond is cleaved and cyclized to form benzothiazole 3d. In route b, the sulfur-sulfur bond of 1a is cleaved by PCl3 first to form 2-aminobenzenethiol B, which reacts with benzoyl chloride produced in situ to form benzothiazole 3d. To test the hypothesis, intermediate A prepared by the reaction of 1a with benzoyl chloride was used to synthesize the benzothiazole 3d under different reaction conditions (Scheme 3). When the intermediate A was heated at 100 °C in toluene without PCl3, no desired product 3d was detected by LC-MS. After adding PCl3 or Ph3P, benzothiazole 3d was obtained in about 27% or 23% yields. These experimental results indicated the sulfur-sulfur bond of intermediate A could be cleaved by PCl3 or Ph3P, but the low yield of 3d indicated that product 3d was not mainly produced by the reaction route a.
Overman, Bach and co-coworkers11 provided some examples that S-S bond could be broken by Ph3P to form thiol, which promoted us to think that PCl3 could also break S-S bond under our experimental conditions. To prove PCl3 could break S-S bond, the reaction of 2,2'-disulfanediyldianiline 1a with PCl3 in toluene was analyzed by LC-MS (Figure 2). Most of disulfide 1a was converted into 2-aminobenzenethiol B after 1a reacted with PCl3 and minimal water in toluene for one hour (Figure 2a-b), which fully show PCl3 could break the S-S bond of the disulfide to form thiol (Scheme 4, Eq. 1). After adding benzoyl chloride to the reaction, the desired product benzothiazole 3d was isolated in 68% and another product D in 17% yield was also obtained (Eq. 2), which agreed with the literature report.12 When 1a and 2d reacted in the presence of PCl3, the same product D was obtained in 11% yield in addition to the desired benzothiazole 3d in 67% yield. Product D was determined to be S-2-benzamidophenyl benzothioate by NMR, MS and single crystal X-ray diffraction analysis (Eq. 3 and Figure 3). However, D was not converted to benzothiazole 3d under the same reaction conditions (Eq. 4), which showed D was a byproduct, not a reaction intermediate. The similar diacylated products like D were also obtained in the reaction of 1a and other carboxylic acids under the same reaction conditions.
Therefore, we propose a tentative reaction mechanism for the formation of 2-substituted benzothiazoles from disulfides as shown in Scheme 5. Carboxylic acid reacts with PCl3 to form acyl chloride first. Through the nucleophilic attack on disulfide 1a by PCl3, 2-aminobenzenethiol B and an intermediate phosphonium cation-thiolate anion salt C are formed, and subsequently thiolate anion C reacts with water which produced from the formation of 3d in the last step to form 2-aminobenzenethiol B.11a,11b At last, the intermediate B reacts with the in-situ produced acyl chloride to give the desired product 3d and byproduct D. In the reaction process, PCl3 acts not only as an acylating reagent to convert carboxylic acid into acyl chloride, but also as a sulfur-sulfur bond reducing reagent to convert disulfide into thiol. Because SOCl2 and POCl3 only act as acylating agents and Ph3P only as a sulfur-sulfur bond reducing reagent, SOCl2, POCl3 and Ph3P couldn’t efficiently facilitate the reaction of disulfide and carboxylic acid (Table 1, entries 2-3).
In summary, we have developed a metal-free method for the synthesis of 2-substituted benzothiazoles from disulfides and carboxylic acids mediated by PCl3 through a sulfur-sulfur bond cleavage/acylation/ cyclization tandem reaction. Both the disulfides and carboxylic acids are stable and readily commercially available. In the reaction process, PCl3 acted as an acylating reagent and a disulfide reducing reagent, which promoted disulfides of 2-aminobenzenethiol reacted with carboxylic acid to produce benzothiazoles. The new synthetic method offers a convenient alternative approach for the construction of biologically important compounds containing a benzothiazole structure.
EXPERIMENTAL
All reagents were purchased from different commercial sources and used without further purification. Solvents were dried over sodium and distilled prior to use. All reactions were performed under a dry argon atmosphere in oven-dried glassware. Reactions were monitored by analytical thin-layer chromatography on silica gel GF254 precoated on glass plates. Column chromatography separations were carried out on silica gel (200-300 mesh). Melting points were measured with SGC X-4 microscopic melting point meter and are uncorrected. Molecular weights were determined by a low-resolution mass spectrometer (ESI) using automatic sampler to inject sample solution. The 1H and 13C NMR spectra were obtained in CDCl3 on a 500 MHz spectrometer and referenced to the residual protonic solvent or TMS. The NMR results were processed by software MestReNova. Elemental analyses were recorded on an Elementar var III-type analyzer. The starting material 1a was purchased from Aladdin Reagent Company. 1b was prepared from 2-amino-4-chlorobenzenethiol13 and 1c was prepared from 1,5-difluoro-2,4-dinitrobenzene (DFDNB) by literature method.10a
General procedure for the synthesis of benzothiazole derivatives 3a-3p, D. 2,2'-Disulfanediyldianiline 1a or 6,6'-disulfanediylbis(3-chloroaniline) 1b (0.5 mmol) and carboxylic acid (1.0 mmol, except 0.5 mmol for 2k) were added to a three-neck flask under an atmosphere of Ar, and 5 mL dry toluene was added, then the mixture was stirred for 2.5 h at 70 C to allow the solid to dissolve in toluene solvent completely. After the reaction solution was cooled down to 30 C, PCl3 (1.2 mmol, 0.165 g) was added dropwise. When the PCl3 was added completely, the reaction mixture was further stirred for 4 - 6 h at 100 C until no 2,2'-disulfanediyldianiline or 6,6'-disulfanediylbis(3-chloroaniline) was detected by TLC analysis. The reaction solution was washed with saturated aqueous sodium bicarbonate solution and extracted with CH2Cl2. The collected organic layers were dried over anhydrous MgSO4. After filtered to remove the MgSO4 and the solvent was removed under reduced pressure. The crude product was purified by flash chromatography on silica gel using PE / EtOAc.
General procedure for the synthesis of benzothiazole derivatives 3q and 3r. 6,6'-Disulfanediylbis[4-(3,5 -dimethylphenoxy)benzene-1,3-diamine] (1c) (0.2 mmol) and carboxylic acid (0.8 mmol) were added to a three-neck flask under an atmosphere of Ar, and 5 mL dry toluene was added, then the mixture was stirred for 2.5 h at 70 C to allow the solid to dissolve in toluene solvent completely. After the reaction solution was cooled down to 30 C, PCl3 (0.96 mmol, 0.132 g) was added dropwise. When the PCl3 was added completely, the reaction mixture was further stirred for 4-6 h at 100 C until no starting material 1c was detected by TLC analysis. The reaction solution was washed with saturated sodium bicarbonate solution and extracted with CH2Cl2. The collected organic layers were dried over anhydrous MgSO4. After filtered to remove the MgSO4 and the solvent was removed under reduced pressure. The crude product was purified by flash chromatography on silica gel.
ACKNOWLEDGEMENTS
This work was supported by the National Natural Science Foundation of China (21362019) and the Inner Monlia Natural Science Foundation of China (2012MS0204).
SUPPLEMENTARY MaTERIALS
Experimental procedures, characterization data, and NMR spectra of all compounds could be obtained in Supplementary Materials.
References
1. (a) K. Ahmed, S. Yellamelli Valli Venkata, N. A. K. Mohammed, F. Sultana, and K. R. Methuku, Expert Opin. Investig. Drugs, 2012, 21, 619; CrossRef (b) K. Serdons, T. Verduyckt, D. Vanderghinste, J. Cleynhens, P. Borghgraef, P. Vermaelen, C. Terwinghe, F. Van Leuven, K. Van Laere, and H. Kung, Bioorg. Med. Chem. Lett., 2009, 19, 602; CrossRef (c) B. L. Mylari, E. R. Larson, T. A. Beyer, W. J. Zembrowski, C. E. Aldinger, M. F. Dee, T. W. Siegel, and D. H. Singleton, J. Med. Chem., 1991, 34, 108. CrossRef
2. J. Han and K. Burgess, Chem. Rev., 2009, 110, 2709. CrossRef
3. (a) G. Bastug, C. Eviolitte, and I. E. Markó, Org. Lett., 2012, 14, 3502; CrossRef (b) G. Naresh, R. Kant, and T. Narender, J. Org. Chem., 2014, 79, 3821; CrossRef (c) M. S. Mayo, X. Yu, X. Zhou, X. Feng, Y. Yamamoto, and M. Bao, Org. Lett., 2014, 16, 764; CrossRef (d) Y. Sun, H. Jiang, W. Wu, W. Zeng, and X. Wu, Org. Lett., 2013, 15, 1598; CrossRef (e) V. N. Bochatay, P. J. Boissarie, J. A. Murphy, C. J. Suckling, and S. Lang, J. Org. Chem., 2013, 78, 1471; CrossRef (f) H. Wang, L. Wang, J. Shang, X. Li, H. Wang, J. Gui, and A. Lei, Chem. Commun., 2012, 48, 76; CrossRef (g) X. Zhang, W. Zeng, Y. Yang, H. Huang, and Y. Liang, Org. Lett., 2014, 16, 876; CrossRef (h) J. Liu, Q. Gui, Z. Yang, Z. Tan, R. Guo, and J.-C. Shi, Synthesis, 2013, 45, 943; CrossRef (i) G. Satish, K. H. V. Reddy, K. Ramesh, K. Karnakar, and Y. V. D. Nageswar, Tetrahedron Lett., 2012, 53, 2518; CrossRef (j) H. Deng, Z. Li, F. Ke, and X. Zhou, Chem. Eur. J., 2012, 18, 4840; CrossRef (k) R. Wang, Y.-l. Ding, H. Liu, S. Peng, J. Ren, and L. Li, Tetrahedron Lett., 2014, 55, 945; CrossRef (l) W. Zhang, Y. Yue, D. Yu, L. Song, Y.-Y. Xu, Y.-J. Tian, and Y.-J. Guo, Adv. Synth. Catal., 2012, 354, 2283; CrossRef (m) S. Liu, R. Chen, H. Chen, and G.-J. Deng, Tetrahedron Lett., 2013, 54, 3838; CrossRef (n) M. Zhang, W.-T. Lu, W. Ruan, H.-J. Zhang, and T.-B. Wen, Tetrahedron Lett., 2014, 55, 1806; CrossRef (o) A. B. Khemnar and B. M. Bhanage, RSC Adv., 2014, 4, 8939; (p) Y. Gao, Q. Song, G. Cheng, and X. Cui, Org. Biomol. Chem., 2014, 12, 1044. CrossRef
4. (a) N. Zhu, Z. Zhang, M. Gao, L. Han, Q. Suo, and H. Hong, Chin. J. Org. Chem., 2013, 33, 1423; CrossRef (b) K. Bahrami, M. M. Khodaei, and A. Nejati, Green Chem., 2010, 12, 1237; CrossRef (c) A. K. Chakraborti, S. Rudrawar, and A. Kondaskar, Synthesis, 2005, 2521. CrossRef
5. (a) D. S. Bose and M. Idrees, J. Org. Chem., 2006, 71, 8261; CrossRef (b) K. Inamoto, C. Hasegawa, J. Kawasaki, K. Hiroya, and T. Doi, Adv. Synth. Catal., 2010, 352, 2643. CrossRef
6. D. Ma, S. Xie, P. Xue, X. Zhang, J. Dong, and Y. Jiang, Angew. Chem. Int. Ed., 2009, 48, 4222. CrossRef
7. (a) S. Murru, H. Ghosh, S. K. Sahoo, and B. K. Patel, Org. Lett., 2009, 11, 4254; CrossRef (b) F. Wang, S. Cai, Z. Wang, and C. Xi, Org. Lett., 2011, 13, 3202; CrossRef (c) D. Ma, X. Lu, L. Shi, H. Zhang, Y. Jiang, and X. Liu, Angew. Chem. Int. Ed., 2011, 50, 1118. CrossRef
8. L. Zhu and M. Zhang, J. Org. Chem., 2004, 69, 7371. CrossRef
9. (a) Z. Wang, R. Tang, and Q. Xiao, Chin. J. Chem., 2011, 29, 314; CrossRef (b) J. Srogl, J. Hývl, Á. Révész, and D. Schröder, Chem. Commun., 2009, 3463; CrossRef (c) A. A. Weekes, M. C. Bagley, and A. D. Westwell, Tetrahedron, 2011, 67, 7743. CrossRef
10. (a) N. Zhu, F. Zhang, and G. Liu, J. Comb. Chem., 2010, 12, 531; CrossRef (b) G. Du, N. Zhu, H. Hong, L. Han, and Q. Suo, Phosphorus, Sulfur Silicon Relat. Elem., 2015, 190, 1154. CrossRef
11. (a) L. E. Overman, D. Matzinger, E. M. O'Connor, and J. D. Overman, J. Am. Chem. Soc., 1974, 96, 6081; CrossRef (b) L. E. Overman and S. T. Petty, J. Org. Chem., 1975, 40, 2779; CrossRef (c) O. Dmitrenko, C. Thorpe, and R. D. Bach, J. Org. Chem., 2007, 72, 8298. CrossRef
12. V. N. Drozd, Zh. Prikl. Khim., 1987, 23, 355.
13. W. E. Fristad and J. R. Peterson, Synth. Commun., 1985, 15, 1.