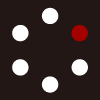
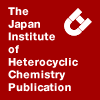
HETEROCYCLES
An International Journal for Reviews and Communications in Heterocyclic ChemistryWeb Edition ISSN: 1881-0942
Published online by The Japan Institute of Heterocyclic Chemistry
e-Journal
Full Text HTML
Received, 5th June, 2015, Accepted, 21st July, 2015, Published online, 23rd July, 2015.
DOI: 10.3987/COM-15-13261
■ Site-Selective Introduction of an Enamido Group at the C(3)-Position of Indoles
Tomoya Miura,* Qiang Zhao, Yuuta Funakoshi, and Masahiro Murakami*
Department of Synthetic Chemistry and Biological Chemistry, Graduate School of Engineering, Kyoto University, youdai-katsura, Nishikyo, Kyoto 615-8510, Japan
Abstract
An enamido group is introduced site-selectively at the C(3)-position of indoles by the rhodium(II)-catalyzed reaction with N-sulfonyl-1,2,3-triazoles. Formally, an α-imino rhodium carbene complex is inserted into the C(3)-H bond of an indole.Indoles are privileged structural motifs found in a myriad of natural products and pharmaceuticals.1 Numerous methods have been developed for the functionalization of indole skeletons.2 Among them, a C-H bond functionalization using metal carbene complexes has received much attention in recent years.3 On the other hand, N-sulfonyl-1,2,3-triazoles have emerged as convenient precursors for the generation of α-imino metal carbene complexes.4 The complexes contain an electrophilic carbene carbon and a nucleophilic imino nitrogen in the molecule. They can trigger a variety of synthetically useful transformations, including not only typical carbene reactions such as cyclopropanation5 and X-H bond insertion (X = carbon,6 oxygen,7 nitrogen8) but also carbene-induced reactions such as [3+2] annulation,9 ring expansion,10 and others.11 Davies and co-workers have demonstrated an excellent method for the functionalization of indole skeletons using α-imino rhodium carbene complexes generated from N-sulfonyl-1,2,3-triazoles; 1,3-disubstituted indoles undergo [3+2] annulation reaction in an enantioselective manner to afford pyrroloindolines (Figure 1(a)).12,13 This fascinating result led us to
examine the rhodium(II)-catalyzed reaction of indole derivatives having C(3)-H bonds. Now, we report a rhodium(II)-catalyzed reaction of 1,2-disubstituted indoles and 1-monosubstituted indoles with N-sulfonyl-1,2,3-triazoles. The presence of the C(3)-H bond shifts the [3+2] annulation pathway to 1,3-insertion of α-imino rhodium carbene complexes into the C(3)-H bond, leading to the formation of α-(3-indolyl)sulfonylenamides with the high (Z)-selectivities (Figure 1(b)).
Initially, 4-phenyl-1-tosyl-1,2,3-triazole (1a) was prepared from phenylacetylene and tosyl azide according to the authentic procedure using a copper(I) catalyst.14 When the triazole 1a (0.2 mmol) was treated with 1,2-dimethyl-1H-indole (2a, 0.3 mmol) in the presence of (tBuCO2)4Rh2 (1 mol%) in chloroform (2 mL) at 80 °C, 1a was completely consumed within 8 hours. Chromatographic purification afforded β-(3-indolyl)sulfonylenamide 3a in 97% isolated yield with the Z/E ratio of >95:5 (Scheme 1). The configuration of the double bond of 3a was confirmed as Z by an NOE study. Unlike the cases with 1,3-disubstituted indoles, no annulation product was formed.15 We propose the following mechanism for the production of 3a. α-Diazo imine 1a’ is generated from the triazole 1a by a ring-chain tautomerization through equilibrium. The transient 1a’ is trapped by a rhodium(II) catalyst to furnish α-imino carbene complex A. Nucleophilic addition of the indole 2a at the C(3)-position to the electrophilic carbene of A gives the zwitterionic intermediate B. The anionic rhodium of B releases an electron pair, which flows onto the imino nitrogen. Since there is a hydrogen atom at the C(3)-position, the imino nitrogen acts as a base to pick up the hydrogen rather than undergoes nucleophilic attack onto the C(2)-carbon atom, furnishing (Z)-isomer of 3a.
1,2-Dimethyl-1H-indole (2a) was reacted with various triazoles 1 (Table 1). Triazoles 1b−e possessing aryl and heteroaryl groups at the C(4)-position all reacted well to afford the corresponding products 3b−e in yields ranging from 90% to 95% with high (Z)-selectivities (entries 1–4). The reaction of the alkyl-substituted triazoles 1f−h gave the products 3f−h, albeit in moderate yields (entries 5–7).7 Aryl group as well as alkyl group were compatible for the R2 substituent on the sulfonyl group to afford the products 3i−m in high yields (entries 8–12).
Next, the use of 1-methyl-1H-indole and its derivatives was examined. The C-H bond functionalization took place site-selectively at the C(3)-position, and the corresponding products 3n−s were obtained in excellent yields (Table 2). In these cases, however, the lower (Z)-selectivities (Z:E = 82:18~88:12) were observed except 3s, which was ascribed to isomerization of the initially formed (Z)-isomer to the (E)-isomer occurring during the course of the reaction. In fact, when the reaction of 1a with 1-methyl-1H-indole was monitored by 1H NMR, the gradual isomerization from (Z)-3n to (E)-3n was observed [96:4 (1 h), 83:17 (4 h), 80:20 (8 h)].
Fused indole 2b with N(1)-to-C(2) was also suitable substrate, giving the product 3t in 95% yield with the Z/E ratio of 87:13 (Eq 1).
An all-in-one-pot procedure was also carried out to demonstrate the practical convenience of the present method (Eq 2). Phenylacetylene (4), tosyl azide, 1,2-dimethyl-1H-indole (2a), copper(I) and rhodium(II) catalysts, and chloroform were put in a reaction vessel, and the mixture was stirred at room temperature. After 3 hours, 4 and tosyl azide were both consumed to generate the triazole 1a. Then, the reaction mixture was stirred at 80 °C for 8 hours, and the following chromatographic purification afforded the product 3a in 79% yield.
The synthetic utility of the products 3 was exemplified by further transformations. The carbon–carbon double bond of 3a, 3n, and 3q was successfully reduced, giving tryptamine derivatives 5, 6, and 7 in good yields when a simple hydrogenation reaction using palladium on charcoal was applied (Eq 3). Furthermore, treatment of 6 with iodine (1.0 equiv) caused electrophilic cyclization to afford fused indole derivative 8 in 77% yield (Eq 4).16
In summary, a regioselective C-H bond functionalization of 1,2-disubstituted- and 1-monosubstituted indoles by α-imino rhodium carbene complexes is reported. The product selectivity is significantly affected by the substitution pattern at the C(3)-position of the indoles. The resulting products are useful intermediates for the synthesis of tryptamine derivatives.
ACKNOWLEDGEMENTS
This work was supported in part by a Grant-in-Aid for Scientific Research (S) from MEXT and the ACT–C program of the JST. We are grateful to the JSPS for Young Scientists for a Research Fellowship to Y. F. We also thank Dr. Y. Nagata (Kyoto University) for kind help in an X-ray analysis.
References
1. Reviews: A. J. Kochanowska-Karamyan and M. T. Hamann, Chem. Rev., 2010, 110, 4489; CrossRef P. M. Dewick, ‘Medicinal Natural Products: A Biosynthetic Approach, 3rd Ed.’, John Wiley & Sons Inc., Chichester, 2009, pp. 311-420. CrossRef
2. Reviews: S. Cacchi and G. Fabrizi, Chem. Rev., 2005, 105, 2873; CrossRef G. Bartoli, G. Bencivenni, and R. Dalpozzo, Chem. Soc. Rev., 2010, 39, 4449; CrossRef M. Bandini and A. Eichholzer, Angew. Chem. Int. Ed., 2009, 48, 9608; CrossRef C. C. J. Loh and D. Enders, Angew. Chem. Int. Ed., 2012, 51, 46. CrossRef
3. Y. Lian and H. M. L. Davies, J. Am. Chem. Soc., 2010, 132, 440; CrossRef A. DeAngelis, V. W. Shurtleff, O. Dmitrenko, and J. M. Fox, J. Am. Chem. Soc., 2011, 133, 1650; CrossRef T. Goto, Y. Natori, K. Takeda, H. Nambu, and S. Hashimoto, Tetrahedron: Asymmetry, 2011, 22, 907; CrossRef Y. Cai, S.-F. Zhu, G.-P. Wang, and Q.-L. Zhou, Adv. Synth. Catal., 2011, 353, 2939; CrossRef H. Qiu, M. Li, L.-Q. Jiang, F.-P. Lv, L. Zan, C.-W. Zhai, M. P. Doyle, and W.-H. Hu, Nature Chem., 2012, 4, 733; CrossRef M. Delgado-Rebollo, A. Prieto, and P. J. Pérez, ChemCatChem, 2014, 6, 2047; CrossRef L. Li, C. Shu, B. Zhou, Y.-F. Yu, X.-Y. Xiao, and L.-W. Ye, Chem. Sci., 2014, 5, 4057; CrossRef L.-Y. Mei, X.-Y. Tang, and M. Shi, Org. Biomol. Chem., 2014, 12, 1149; CrossRef Y. Xing, G. Sheng, J. Wang, P. Lu, and Y. Wang, Org. Lett., 2014, 16, 1244. CrossRef
4. Reviews: A. V. Gulevich and V. Gevorgyan, Angew. Chem. Int. Ed., 2013, 52, 1371; CrossRef H. M. L. Davies and J. S. Alford, Chem. Soc. Rev., 2014, 43, 5151; CrossRef P. Anbarasan, D. Yadagiri, and S. Rajasekar, Synthesis, 2014, 46, 3004. CrossRef
5. S. Chuprakov, S. W. Kwok, L. Zhang, L. Lercher, and V. V. Fokin, J. Am. Chem. Soc., 2009, 131, 18034; CrossRef T. Miura, T. Nakamuro, C.-J. Liang, and M. Murakami, J. Am. Chem. Soc., 2014, 136, 15905. CrossRef
6. S. Chuprakov, J. A. Malik, M. Zibinsky, and V. V. Fokin, J. Am. Chem. Soc., 2011, 133, 10352; CrossRef D. Yadagiri and P. Anbarasan, Org. Lett., 2014, 16, 2510; CrossRef S. Park, W.-S. Yong, S. Kim, and P. H. Lee, Org. Lett., 2014, 16, 4468. CrossRef
7. T. Miura, T. Biyajima, T. Fujii, and M. Murakami, J. Am. Chem. Soc., 2012, 134, 194; CrossRef T. Miura, T. Tanaka, T. Biyajima, A. Yada, and M. Murakami, Angew. Chem. Int. Ed., 2013, 52, 3883. CrossRef
8. S. Chuprakov, B. T. Worrell, N. Selander, R. K. Sit, and V. V. Fokin, J. Am. Chem. Soc., 2014, 136, 195; CrossRef D. J. Lee and E. J. Yoo, Org. Lett., 2015, 17, 1830. CrossRef
9. T. Horneff, S. Chuprakov, N. Chernyak, V. Gevorgyan, and V. V. Fokin, J. Am. Chem. Soc., 2008, 130, 14972; CrossRef T. Miura, M. Yamauchi, and M. Murakami, Chem. Commun., 2009, 1470; CrossRef B. Chattopadhyay and V. Gevorgyan, Org. Lett., 2011, 13, 3746; CrossRef M. Zibinsky and V. V. Fokin, Angew. Chem. Int. Ed., 2013, 52, 1507; CrossRef S. Chuprakov, S. W. Kwok, and V. V. Fokin, J. Am. Chem. Soc., 2013, 135, 4652; CrossRef T. Miura, Y. Funakoshi, and M. Murakami, J. Am. Chem. Soc., 2014, 136, 2272. CrossRef
10. T. Miura, Y. Funakoshi, M. Morimoto, T. Biyajima, and M. Murakami, J. Am. Chem. Soc., 2012, 134, 17440; CrossRef N. Selander, B. T. Worrell, and V. V. Fokin, Angew. Chem. Int. Ed., 2012, 51, 13054; CrossRef R. Liu, M. Zhang, G. Winston-McPherson, and W. Tang, Chem. Commun., 2013, 49, 4376; CrossRef F. Medina, C. Besnard, and J. Lacour, Org. Lett., 2014, 16, 3232. CrossRef
11. E. E. Schultz and R. Sarpong, J. Am. Chem. Soc., 2013, 135, 4696; CrossRef B. T. Parr, S. A. Green, and H. M. L. Davies, J. Am. Chem. Soc., 2013, 135, 4716; CrossRef J. S. Alford, J. E. Spangler, and H. M. L. Davies, J. Am. Chem. Soc., 2013, 135, 11712; CrossRef T. Miura, T. Tanaka, K. Hiraga, S. G. Stewart, and M. Murakami, J. Am. Chem. Soc., 2013, 135, 13652; CrossRef J.-M. Yang, C.-Z. Zhu, X.-Y. Tang, and M. Shi, Angew. Chem. Int. Ed., 2014, 53, 5142; CrossRef H. Shang, Y. Wang, Y. Tian, J. Feng, and Y. Tang, Angew. Chem. Int. Ed., 2014, 53, 5662; CrossRef K. Chen, Z.-Z. Zhu, Y.-S. Zhang, X.-Y. Tang, and M. Shi, Angew. Chem. Int. Ed., 2014, 53, 6645; CrossRef E. E. Schultz, V. N. G. Lindsay, and R. Sarpong, Angew. Chem. Int. Ed., 2014, 53, 9904; CrossRef D. J. Lee, H. S. Han, J. Shin, and E. J. Yoo, J. Am. Chem. Soc., 2014, 136, 11606; CrossRef D. J. Jung, H. J. Jeon, J. H. Kim, Y. Kim, and S. Lee, Org. Lett., 2014, 16, 2208; CrossRef A. Boyer, Org. Lett., 2014, 16, 5878; CrossRef J. Fu, H. Shen, Y. Chang, C. Li, J. Gong, and Z. Yang, Chem. Eur. J., 2014, 20, 12881; CrossRef H.-D. Xu, Z.-H. Jia, K. Xu, H. Zhou, and M.-H. Shen, Org. Lett., 2015, 17, 66. CrossRef
12. J. E. Spangler and H. M. L. Davies, J. Am. Chem. Soc., 2013, 135, 6802. CrossRef
13. For a thermal reaction of 4-alkoxy-1,2,3-triazoles with indoles, see: J. S. Alford and H. M. L. Davies, J. Am. Chem. Soc., 2014, 136, 10266. CrossRef
14. J. Raushel and V. V. Fokin, Org. Lett., 2010, 12, 4952. CrossRef
15. The reaction of 1a with 2a was carried out under the Davies’s conditions (1 mol% (S-PTAD)4Rh2, cyclohexane, 65 °C, 4 h).12 However, no annulation product was formed, and 3a was obtained in 88% yield.
16. Y.-X. Li, H.-X. Wang, S. Ali, X.-F. Xia, and Y.-M. Liang, Chem. Commun., 2012, 48, 2343. CrossRef