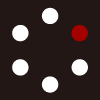
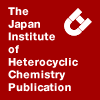
HETEROCYCLES
An International Journal for Reviews and Communications in Heterocyclic ChemistryWeb Edition ISSN: 1881-0942
Published online by The Japan Institute of Heterocyclic Chemistry
e-Journal
Full Text HTML
Received, 11th June, 2015, Accepted, 31st July, 2015, Published online, 6th August, 2015.
■ Regioselective Synthesis of Pyrazoles and Pyrazolo[1,5-a]Pyrimidines: Structural Characterization by HMBC NMR
Abdullah S. Al-Bogami and Tamer S. Saleh*
Department of Green Chemistry, National Research Centre, El-Tahrir Street, Dokki, Giza 12622, Egypt
Abstract
In this study a series of novel pyrazoles and pyrazolo[1,5-a]pyrimidines with a sulfone moiety incorporated the coumarin ring were efficiently synthesized. These pyrazoles and pyrazolo[1,5-a]pyrimidines were synthesized by treating enaminone derivative of β-ketosulfone (3-(2-tosylacetyl)-2H-coumarin) with substituted hydrazines and 5-aminopyrazole derivatives, respectively under microwave irradiation. The structures of the products thus obtained were characterized by 2D NMR (HMBC).Coumarin derivatives form a family of organic compounds that are of interest in contemporary drug discovery.1,2 Coumarin and its derivatives are widely distributed in nature, and they exhibit a broad pharmacological profile.3,4 In addition, well-known drugs such as batoprazine (a serenic or antiaggressive agents, I)5-7 and lamellarin (a selective inhibitor of HIV-I integrase, II)8 contain the coumarin (Figure 1).
Because of their unique pharmacological activities, it is of interest to further derivatize the coumarin backbone using different heterocycles and screen the derivatives thus obtained for their potential as novel therapeutic agents. Meanwhile, the importance of heterocycles such as pyrazoles and pyrazolo[1,5-a]pyrimidines has increased because they have been reported to be extremely useful intermediates for the preparation of new biological materials; they are also present in numerous pharmacologically and agrochemically important compounds.9,10
Moreover, the introduction of an arylsulfonyl moiety into heterocycles has been reported to afford compounds that exhibit high biological activity and selectivity.11,12 In light of their potent activities, a number of studies have been devoted to these nuclei; most of our research has focused on the elaboration of methods for synthesizing aryl sulfonyl-containing compounds.
Microwave chemistry and microwave-assisted organic synthesis (MAOS) are effective tools in medicinal chemistry.13,14 The use of MAOS has been demonstrated to dramatically reduce processing times, increase product yields.
In continuation with our studies on the synthesis of a wide range of heterocyclic systems for biological screening,15-20 herein, we discuss the reactivity of versatile, hitherto unreported E-3-(3-(dimethylamino)-2-tosylacryloyl)-2H-1-benzopyran-2-one (2) toward nitrogen-containing nucleophiles by microwave irradiation and conventional methods. Moreover, this method provides a convenient route for synthesizing novel pyrazoles or pyrazolo[1,5-a]pyrimidines containing a sulfone moiety incorporated the coumarin ring. The structures of the regioisomers obtained were characterized by 2D NMR.
1. SYNTHESIS OF ENAMINONE 2 AND ITS REACTION WITH SUBSTITUTED HYDRAZINES
A reaction of equimolar quantities of 3-(2-tosylacetyl)-2H-1-benzopyran-2-one (1) and dimethylformamide dimethyl acetal (DMF-DMA) was carried out in toluene under microwave irradiation. The key intermediate, E-3-[3-(dimethylamino)-2-tosylacryloyl]-2H-1-benzopyran-2-one (2) was obtained within 10 minو as evidenced by TLC (Scheme 1), while the same reaction was completed in 4 h when refluxed in toluene.
considered versatile, hitherto, unreported, synthetic intermediates that combine the ambident nucleophilicity of enamines with the ambident electrophilicity of enones. Moreover, the synthesis of enaminocarbonyl groups has considerable scope, considering that there are three nucleophilic sites (a, c, and e) and two electrophilic sites (b and d) in the moiety (as shown in Figure 2).23,24
Because of its unique structure of E-3-(3-(dimethylamino)-2-tosylacryloyl)-2H-1-benzopyran-2-one (2) (Figure 2), it undergoes reaction with different nitrogen nucleophiles such as substituted hydrazines or heterocyclic amines; these transformations usually proceed under mild reaction conditions with low regio- and stereoselectivity. This low selectivity can be explained not only by the small difference in the electrophilicity of the carbonyl and β-carbon centers of β-enaminones but also by the nucleophilicity of the hydrazine nitrogen atoms.
Previous studies have demonstrated that the regioselectivity of the cyclocondensation of β-enaminones and substituted hydrazines depends on the electronic and steric structure of β-enaminones, reactivity of hydrazines, and reaction conditions.25-31 Some studies have used β-enaminones for the highly regioselective synthesis of pyrazoles and demonstrated that regioselectivity is related to the β-enaminone structure. 25-28 On the other hand, other studies have reported complex mixtures of isomers, demonstrating that the low regioselectivity is associated the reactivity of the substituted hydrazine, as shown in Scheme 2.29,30 The reaction shown in Scheme 2 between N-substituted hydrazines and β-ketosulfone enaminones 3 may form two regioisomeric pyrazoles A and B through the pathways (Red and Blue paths, respectively) shown. However, to the best of our knowledge, it is difficult to determine the structures of the reaction products (in either case of low or high regioselectivity). Nevertheless, most studies have differentiated between the two isomers after their separation by the following approaches. First involve reaction with butyllithium, where product identification is carried out by taking advantage of the difference in the activity of two isomers (1,4,5-trisubstuted pyrazole A and 1, 3,4-trisubstuted pyrazole B) toward butyllithium,32 however, this is expensive and suffers from high attrition. Second, single crystal X-ray analysis can be employed, considered as a useful tool for the unambiguous structure determination of the obtained products; however, sometimes, it is difficult to obtain a single crystal of the formed product. Recently, our research group employed 2D NMR techniques such as 1H-13C HMBC as a simple analytical tool for elucidating the structure of compounds.33
To the best of our knowledge, 1H-13C HMBC studies of pyrazole derivatives formed from the above-mentioned reaction in Scheme 2 have not been reported.
The reactivity of 2 toward some nitrogen nucleophiles was investigated. For this purpose, compound 2 was treated with phenylhydrazine derivatives in ethanol under microwave irradiation. One isolable product was afforded in each case (as indicated by TLC analysis) in excellent yields and at a reaction time shorter than that observed under conventional conditions (reflux in ethanol, Scheme 3). Table 1 shows the reaction times and product yields.
Scheme 3 shows the formation of the possible products from the reaction between 2 and phenylhydrazine derivatives. As can be observed, either of the isomers 5 or 6 could be formed (on the basis of elemental analysis and spectroscopic data of the isolated products), with the coumarin-2-yl group being located either at position 5 or 3 of the pyrazole ring, respectively. In each case, the 1H NMR spectra of the isolated products, exhibit disappearance of the NMe2 and olefinic proton signals, while a singlet signal appeared in the region δ 8.49-8.52, which corresponds to the pyrazole proton. Furthermore, one carbonyl absorption band was observed in the IR spectra of the isolated products.
Herein, we utilized 2D NMR to unambiguously elucidate the structure of the obtained regioisomer by utilizing long-range C-H connectivities via 1H-13C HMBC. The technique can determine 2J and 3J correlations between 13C and 1H multiple bonds while detecting connectivity with or through nuclei without attached protons. From the full 1H-13C HMBC spectrum of the isolated regioisomer. A signal was observed at δ 158.04 ppm corresponding to the carbonyl carbon of coumarin; it exhibited correlation peak with the singlet signal at δ 8.39 ppm assigned to coumarin H-4. Thus, the singlet signal at δ 8.52 ppm is assigned to the pyrazole proton. Based on the number of correlations between the pyrazole proton and carbons that are two and three bonds away we can easily determine which isomer formed.
The presence of two correlations in the 1H-13C HMBC spectrum of the isolated product is conclusive evidence for the proposed structure of 5c. Hence, the pyrazole isomers 5a-c are assumed to be produced via the Red Path shown in Scheme 2 (c.f. Supporting Information S1).
As shown in Table 1, microwave irradiation decreased the reaction time from several hours to minutes, with improved the product yields (88% to 97%) as compared with those obtained (60% to 72%) under conventional conditions. Although we did not investigate the effect of substituents on the reaction, the presence of electron-withdrawing groups such as CF3 favored the reaction owing to a decrease in the nucleophilicity of the secondary amino group. As can be seen in Table 1, compound 5c was obtained in the highest yield in the shortest reaction time.
2. REACTION OF ENAMINONE 2 WITH 5-AMINOPYRAZOLE DERIVATIVES
Encouraged by the successful characterization of pyrazole derivatives 5a-c, the reaction protocol was further extended to study the reactions of 2 with other nitrogen nucleophiles (such as 5-aminopyrazole derivatives) under microwave irradiation to synthesize novel pyrazolo[1,5-a]pyrimidines derivatives with a sulfone moiety incorporated the coumarin ring. Also to unambiguous structural characterization of the formed pyrazolo[1,5-a]pyrimidine isomers.
For this purpose, enaminone 2 was treated with 5-amino-1H-pyrazole derivatives 7a-i in ethanol and presence of catalytic amount of piperidine under microwave irradiation. In each case, the reaction only afforded one isolable product, as shown by TLC analysis (Scheme 4). Table 2 shows the reaction times and yields.
As shown in Scheme 4, three possible products 9, 10 and 12 can be obtained. The pyrazolo[3,4-b]pyridine derivative 10 (in case of aminopyrazole derivatives 7a-d with no substitution at C-4) was easily ruled out on the basis of the 1H NMR spectra of the reaction products. In each case, the 1H NMR spectrum exhibited a singlet at δ = 6.64 to 6.79 ppm, corresponding to the pyrazole proton.
Notably, products 9, 10, and 12 are possibly formed by the initial attack of the endocyclic nitrogen or the exocyclic amino group on the activated double bond (Figure 3).34
In addition, most of the associated studies have confirmed that the above reaction typically leads to the formation of pyrazolo[1,5-a]pyrimidines similar to 9, as supported by X-ray crystallography.19,20,35,36 In contrast, Shaaban, has recently reported results of an interesting study dealing with the same reaction37 wherein the corresponding pyrazole[1,5-a]pyrimidine had structure similar to that 12 which was also confirmed by X-ray crystallography. The above mentioned findings suggest that there is no general basis for the determination of the preferred isomer; typically, it is difficult to present unequivocal proof for the structure of 9 or 12. Although 1H and 13C NMR can support the formation of pyrazolo[1,5-a]pyrimidine, these techniques cannot differentiate between the two isomers 9 and 12 or exclude either. Meanwhile, it is crucial to rationalize the observed regioselectivity for these aza-Michael addition reactions (Scheme 4).
Elemental analyses and spectroscopic data of the isolated products show the possible occurrence of two isomeric structures, 9a-i or 12a-i. The mass spectrum of the obtained product for the reaction of 2 with aminopyrazole derivative 7e revealed a molecular ion peak at m/z 507. In its 1H NMR spectrum, four singlets were observed at δ 2.39, 2.52, 8.30, and 9.16 ppm, which correspond to two methyl, coumarin H-4, and pyrimidine protons, respectively. In addition, aromatic proton signals were observed as multiplet between δ 7.39 and 7.86 ppm. The positions of coumarin H-4 and the pyrimidine proton were supported by 1H-13C HMBC. from the full 1H-13C HMBC spectrum of the formed regioisomer. A signal was observed at δ 157.19 ppm, which corresponds to the carbonyl carbon of coumarin; it exhibited correlation peak with a singlet signal at δ 8.30 ppm, assigned to coumarin H-4. Thus, the singlet signal at δ 9.16 ppm is assigned to the pyrimidine proton (cf. Supporting Information S2).
In this section, the structures of the obtained regioisomers were unambiguously elucidate by advanced 1H-15N HMBC techniques, where all connectivities were observed for the assignment the nitrogen chemical shifts. Using the product obtained from the reaction of 2 with the aminopyrazole derivative 7e as representative examples (Figure 4), the chemical shifts of the nitrogen atoms are N-4 (280.40 ppm), N-1 (275.45 ppm) and N-7a (216.12 ppm), consistent with those reported in literature.38,39
The 1H–15N HMBC spectrum of the obtained product show that pyrimidine nitrogen atom 4 (indicated by purple) was key in differentiating between the two possible isomers. Connectivity was observed between the pyrimidine proton and N-4 (2JH3-N4) (cf. Supporting Information S3). Therefore, the results confirmed the existence of isomer 9e over the alternative structure 12e.
In addition to the other observed connectivities in the 1H–15N HMBC spectrum, connectivity was observed between the methyl proton (position 2) and N-1. Furthermore, N-7a can be used to differentiate between the two isomers, however, no connectivity was observed between pyrimidine-H and N-7a. Therefore, this lack of connectivity served as additional evidence for the selective formation of 9e (cf. Supporting Information S3).
As shown in Scheme 4, novel pyrazolo[1,5-a]pyrimidine derivatives 9a-i were assumed to form via the initial Michael addition of the exocyclic amino group in the 5-aminopyrazole derivative 7a-i to the α,β-unsaturated moiety in enaminone 2, affording the corresponding acyclic non-isolable intermediates 8; the acyclic intermediates then undergo intramolecular cyclization and aromatization via the loss of dimethylamine and water to form the final product 9 (Scheme 4).
Notably, most previous studies support an alternative synthesis for some of the isomers.19 For example, 9a has been well established as the product of an alternative synthesis of 5-aminopyrazole derivative 7a with DMF–DMA and subsequent condensation of the obtained amidine.36 Furthermore, 5-N-(N,N-dimethylamino-methylene)amino-3-methyl-1H-pyrazole (13) with 3-(2-tosylacetyl)-2H-1-benzopyran-2-one (1) under microwave irradiation afforded a product identical in all respects (mp, mixed mp, and spectra) to 9a (Scheme 5).
On the other hand, to investigate the advantage of microwave irradiation, the previously mentioned reactions were carried out using conventional methods (reflux in ethanol with a catalytic amount of piperidine, Table 2). From the results, the microwave protocol afforded the products in shorter time and with a higher yield. Hence, microwave irradiation was also observed to have a beneficial effect on the synthesis of pyrazolo[1,5-a]pyrimidine derivatives 9a-i: the reaction time decreased substantially from 6 to 12 h with conventional heating to less than 50 min. In addition, the yields of products were noticeably improved.
In conclusion, we present a mild, rapid, and efficient method for the highly regioselective synthesis of a new series of pyrazoles and pyrazolo[1,5-a]pyrimidines with the sulfone moiety incorporated into the coumarin ring under microwave irradiation. The products thus obtained were not only synthesized in a shorter reaction time but also had yields higher than those obtained by conventional heating. The structures of the obtained regioisomers were unambiguously determined by 1H-13C HMBC (in case of pyrazoles) and 1H-15N HMBC (in case of pyrazolo[1,5-a]pyrimidines).
EXPERIMENTAL
All organic solvents were purchased from commercial sources and used as received unless otherwise stated. All other chemicals were purchased from Merck, Aldrich or Acros and used without further purification. Thin-layer chromatography (TLC) was performed on pre-coated Merck 60 GF254 silica gel plates using a fluorescent indicator, and detection was carried out using UV light at 254 and 360 nm. Melting points were measured on a Stuart melting point apparatus and were uncorrected. IR spectra were recorded on Nicolet iS10 FT-IR spectrometer with Smart iTR, which is an ultra-high-performance, versatile attenuated total reflectance (ATR) sampling accessory. The NMR spectra were recorded on a Bruker Avance III 400 (9.4 T, 400.13 MHz for 1H, 100.62 MHz for 13C, 376.25 MHz for 19F and 40.56 MHz for 15N) spectrometer with a 5-mm BBFO probe, at 298 K. Chemical shifts (δ in ppm) are given relative to the internal solvent: DMSO-d6 2.50 for 1H, 39.50 for 13C, and for 15N NMR, nitromethane (0.0) was used as an external standard. The standard Bruker pulse sequence hmbcgplpndqf was used for 1H–15N HMBC experiments. Mass spectra were recorded on a Thermo ISQ single quadrupole gas chromatography-mass spectrometer. Elemental analyses were conducted on a EuroVector instrument C, H, N, S analyzer EA3000 Series.
Microwave experiments were conducted on a CEM Discover & Explorer SP microwave apparatus (300 W), utilizing 35 mL capped glass reaction vessels with automated power control based on temperature feedback.
Starting Materials. 3-(2-Tosylacetyl)-2H-1-benzopyran-2-one (1),22 5-amino-1H-pyrazole derivatives 5a-i40,41 and 5-N-(N,N-dimethylaminomethylene)amino-3-methyl-1H-pyrazole (13)36 were prepared by the appropriate reported procedure. All other chemicals used in this study were commercially available.
Synthesis of E-3-(3-(dimethylamino)-2-tosylacryloyl)-2H-1-benzopyran-2-one (2)
Method A (Conventional Method). To a solution of 3-(2-tosylacetyl)benzopyran-2-one (1) (3.42 g, 10 mmol) in toluene (30 mL), dimethylformamide dimethyl acetal (DMF-DMA) (2.62 mL, 20 mmol) was added and the mixture was refluxed for 4 h (until no starting materials showed in TLC). The excess toluene and DMF-DMA were distilled off under reduced pressure. The residual solid was taken up in Et2O (20 mL) and the resulting crystals were collected by filtration, washed thoroughly with Et2O, dried, and finally recrystallized from absolute EtOH to afford enaminone 2 (2.21 g, 55.7% yield).
Method B (Microwave Method). This process was performed under microwave irradiation (300 W, 100 oC) on the same scale as that described above. Here the reactants were dissolved in toluene and subjected to microwave irradiation for 10 min until the starting materials could no longer detected by TLC. The product was obtained and separated (3.66 g, 92.2% yield) in the same manner as that described above in the conventional reaction.
Yellowish white solid, mp 221-223 oC; IR (KBr) v/cm-1: 1733,1705 (2CO), 1379, 1130 (SO2); 1H NMR (DMSO-d6): δ 2.33 (s, 3H, CH3), 2.90 (s, 3H, CH3), 2.98 (s, 3H, CH3),7.21-8.10 (m, 8H, ArH), 8.27 (s, 1H, =CH), 8.71 (s, 1H, coumarin H-4); 13C NMR (DMSO-d6): δ 21.52, 36.54, 116.66, 118.09, 125.36, 127.09, 128.51,128.99, 130.59, 132.86, 135.29, 136.51, 140.25, 148.93, 154.28, 159.17, 162.57, 185.88; MS: m/z 397 (M+); Anal. Calcd for C21H19NO5S (397.44): C, 63.46; H, 4.28; N, 3.52; S, 8.07. Found: C, 63.73; H, 4.76; N, 3.42; S, 7.96%.
Typical procedure for synthesis of pyrazole derivatives 5a-c
Method A (Conventional Method). A mixture of enaminone 2 (0.397g, 1 mmol) and the appropriate substituted hydrazine derivatives 4a-c (1.5 mmol) in absolute EtOH (15 mL) was refluxed for an appropriate time (Table 1). The progress of the reaction was monitored by TLC (eluent; EtOAc: CHCl31:10). Upon completion of the reaction, the solvent was evaporated under reduced pressure to obtain the solid product which was crystallized from EtOH to afford the corresponding pyrazole derivatives 5a–c.
Method B (Microwave Method). This process was performed on the same scale as that described above. Here the reactants were dissolved in toluene and subjected to microwave irradiation for an appropriate time (Table 1) until the starting materials could no longer detected by TLC. The product was obtained in excellent yield and high purity than the described above in the conventional reaction.
3-(1-Phenyl-4-tosyl-1H-pyrazol-5-yl)-1-benzopyran-2-one (5a): White solid, mp 236-238 oC; IR (KBr) v/cm-1: 1705 (CO), 1601(C=N), 1345, 1136 (SO2); 1H NMR (DMSO-d6): δ 2.31 (s, 3H, CH3), 7.23-7.74 (m, 13H, ArH), 8.33 (s, 1H, coumarin H-4), 8.49 (s, 1H, pyrazole-H); 13C NMR (DMSO-d6): δ 21.43, 115.12, 116.54, 119.57, 122.45, 126.35, 127.09, 127.38, 127.98, 129.11, 129.65, 130.08, 130.27, 134.26, 137.96 139.54, 141.64, 141.96, 149.84, 154.19, 158.95; MS: m/z 442 (M+); Anal. Calcd for C25H18N2O4S (442.49): C, 67.86; H, 4.10; N, 6.33; S, 7.25. Found: C, 68.15; H, 3.98; N, 6.24; S, 7.17%.
3-(1-(4-Tolyl)-4-tosyl-1H-pyrazol-5-yl)-1-benzopyran-2-one (5b): Buff crystal, mp 228-230 oC; IR (KBr) v/cm-1: 1713 (CO), 1600(C=N), 1321, 1144 (SO2); 1H NMR (DMSO-d6): δ 2.29 (s, 3H, CH3), 2.33 (s, 3H, CH3), 7.26-7.71 (m, 12H, ArH), 8.27 (s, 1H, coumarin H-4), 8.51 (s, 1H, pyrazole-H); 13C NMR (DMSO-d6): δ 21.22, 21.29, 114.53, 118.78, 120.86, 125.31, 126.01, 126.94, 127.13, 128.11, 128.53, 130.00, 130.14, 132.89, 136.01, 136.51, 140.78, 140.95, 142.32, 148.58, 152.21, 158.17; MS: m/z 456 (M+); Anal. Calcd for C26H20N2O4S (456.51): C, 68.41; H, 4.42; N, 6.14; S, 7.02. Found: C, 68.65; H, 4.34; N, 6.04; S, 6.96%.
3-(1-(4-Trifluoromethylphenyl)-4-tosyl-1H-pyrazol-5-yl)-1-benzopyran-2-one (5c): White crystal, mp 209-211 oC; IR (KBr) v/cm-1: 1705 (CO), 1603 (C=N), 1332, 1128 (SO2); 1H NMR (DMSO-d6): δ 2.36 (s, 3H, CH3), 7.35-7.86 (m, 12H, ArH), 8.39 (s, 1H, coumarin H-4), 8.52 (s, 1H, pyrazole-H); 13C NMR (DMSO-d6): δ 21.49, 114.47, 117.08, 118.24, 122.77, 124.23, 125.47, 125.76, 125.89, 127.25, 127.29, 127.39, 130.07, 130.45, 134.42, 137.63, 139.15, 141.50, 141.97, 144.98, 149.25, 154.16, 158.04; 19F NMR (DMSO-d6): δ -61.57; MS: m/z 510 (M+); Anal. Calcd for C26H17F3N2O4S (510.48): C, 61.17; H, 3.36; N, 5.49; S, 6.28. Found: C, 61.42; H, 3.31; N, 5.37; S, 6.20%.
Typical procedure for synthesis of pyrazolo[1,5-a]pyrimidine derivatives 9a-i
Method A (Conventional Method). A mixture of enaminone 2 (0.397g, 1 mmol), and the appropriate heterocyclic amine (5-amino-1H-pyrazole derivatives 5a-i, in EtOH (25 mL) and a catalytic amount of piperidine (1-2 drops) was heated under reflux for the required time to complete the reaction, which was monitored by TLC (Table 2), and then left to cool. The solid was collected by filtration, washed with EtOH, dried and finally recrystallized from EtOH/DMF to afford the corresponding pyrazolo[1,5-a]pyrimidines 9a-i.
Method B (Microwave Method). This process was performed using microwave irradiation on the same scale as that described above. In this method, first, the reactants were added to EtOH (5 mL), and next, the total mixture was placed in a process vial in the microwave oven and was irradiated with a power of 300 W to reach a reaction temperature of 100 oC under auto-generated pressure. The vial was exposed to microwave irradiation for the appropriate time (Table 2) until the starting materials could no longer detected by TLC (eluent EtOAC/CHCl3 1:10). Upon completion of the reaction, the solvent was evaporated under reduced pressure. The obtained solid product was separated as described above in the conventional reaction to afford the corresponding the corresponding pure pyrazolo[1,5-a]pyrimidines 9a-i.
Method C (Alternative Synthetic Pathway). A mixture of 3-(2-tosylacetyl)coumarin (1) (3.42 g, 10 mmol) and an equivalent molar ratio of 5-N-(N,N-dimethylamino-methylene)amino-3-methyl-1H-pyrazole (13) in EtOH (5 mL) with 2 drops of piperidine was subjected to microwave irradiation for 50 min, as evidenced by TLC. The solid was taken and collected by filtration, washed with EtOH, dried, and finally recrystallized from EtOH/DMF to afford a product that is identical in all aspects (mp, mixed mp, TLC, IR, and mass spectra) to 9a.
The physical and spectral data of the synthesized compounds 9a-i are listed below:
3-(2-Methyl-6-tosylpyrazolo[1,5-a]pyrimidin-7-yl)-1-benzopyran-2-one (9a): Yellow crystals, mp 282-284 oC; IR (KBr) v/cm-1: 1711 (CO), 1593 (C=N), 1325, 1123 (SO2); 1H NMR (DMSO-d6): δ 2.39 (s, 3H, CH3), 2.57 (s, 3H, CH3), 6.79 (s, 1H, pyrazole H-3), 7.01-7.71 (m, 8H, ArH), 8.26 (s, 1H, coumarin H-4), 9.11 (s, 1H, pyrimidine H-5); 13C NMR (DMSO-d6): δ 14.14, 22.31, 96.01, 114.13, 119.56, 123.74, 125.67, 127.60, 127.94, 128.95, 129.00, 129.89, 137.25, 138.90, 144.01, 144.25, 145.59, 149.22, 151.03, 156.78, 158.97; MS: m/z 431 (M+); Anal. Calcd for C23H17N3O4S (431.46): C, 64.03; H, 3.97; N, 9.74; S, 7.43. Found: C, 64.31; H, 3.88; N, 9.62; S, 7.36%.
3-(2-Phenyl-6-tosylpyrazolo[1,5-a]pyrimidin-7-yl)-1-benzopyran-2-one (9b): Yellow crystals, mp 291-293 oC; IR (KBr) v/cm-1: 1703 (CO), 1599 (C=N), 1343, 1137 (SO2); 1H NMR (DMSO-d6): δ 2.31 (s, 3H, CH3), 6.79 (s, 1H, pyrazole H-3), 6.99-7.89 (m, 13H, ArH), 8.17 (s, 1H, coumarin H-4), 9.32 (s, 1H, pyrimidine H-5); 13C NMR (DMSO-d6): δ 21.85, 98.12, 114.47, 117.52, 117.89, 119.78, 121.40, 124.03, 125.18, 127.20, 128.00, 128.49, 128.91, 129.10, 130.45, 133.89, 137.37, 138.96, 144.00, 145.85, 149.13, 151.22, 154.70, 158.13; MS: m/z 493 (M+); Anal. Calcd for C28H19N3O4S (493.53): C, 68.14; H, 3.88; N, 8.51; S, 6.50. Found: C, 68.41; H, 3.79; N, 8.40; S, 6.43%.
3-(2-(4-Tolyl)-6-tosylpyrazolo[1,5-a]pyrimidin-7-yl)-1-benzopyran-2-one (9c): Yellow crystals, mp 275-277 oC; IR (KBr) v/cm-1: 1712 (CO), 1604 (C=N), 1334, 1151 (SO2); 1H NMR (DMSO-d6): δ 2.31 (s, 3H, CH3), 2.47 (s, 3H, CH3), 6.64 (s, 1H, pyrazole H-3), 7.09-7.86 (m, 12H, ArH), 8.23 (s, 1H, coumarin H-4), 9.21 (s, 1H, pyrimidine H-5); 13C NMR (DMSO-d6): δ 21.36, 21.45, 98.13, 114.03, 116.19, 118.63, 119.00, 121.53, 124.49, 125.15, 127.58, 128.00, 128.16, 128.96, 129.98, 130.11, 133.63, 137.48, 138.96, 144.00, 145.98, 149.22, 152.07, 154.97, 158.65; MS: m/z 507 (M+); Anal. Calcd for C29H21N3O4S (507.56): C, 68.62; H, 4.17; N, 8.28; S, 6.32. Found: C, 68.88; H, 4.10; N, 8.16; S, 6.25%.
3-(2-(4-Methoxyphenyl)-6-tosylpyrazolo[1,5-a]pyrimidin-7-yl)-1-benzopyran-2-one (9d): Yellow crystals, mp 286-288 oC; IR (KBr) v/cm-1: 1702 (CO), 1601 (C=N), 1333, 1149 (SO2); 1H NMR (DMSO-d6): δ 2.36 (s, 3H, CH3), 3.81 (s, 3H, OCH3), 6.71 (s, 1H, pyrazole H-3), 7.20-7.89 (m, 12H, ArH), 8.27 (s, 1H, coumarin H-4), 9.30 (s, 1H, pyrimidine H-5); 13C NMR (DMSO-d6): δ 21.29, 54.13, 99.11, 114.16, 117.45, 118.14, 118.88, 121.23, 124.75, 125.76, 127.94, 128.64, 128.84, 128.97, 129.01, 130.22, 133.51, 137.03, 138.74, 145.51, 149.00, 152.17, 154.97, 158.65, 159.46; MS: m/z 523 (M+); Anal. Calcd for C29H21N3O5S (523.56): C, 66.53; H, 4.04; N, 8.03; S, 6.12. Found: C, 66.77; H, 3.95; N, 7.93; S, 6.07%.
3-(2-Methyl-3-phenyl-6-tosylpyrazolo[1,5-a]pyrimidin-7-yl)-1-benzopyran-2-one (9e): Yellow crystals, mp 277-279 oC; IR (KBr) v/cm-1: 1709 (CO), 1599 (C=N), 1342, 1149 (SO2); 1H NMR (DMSO-d6): δ 2.39 (s, 3H, CH3), 2.52 (s, 3H, CH3), 7.39-7.86 (m, 13H, ArH), 8.30 (s, 1H, coumarin H-4), 9.16 (s, 1H, pyrimidine H-5); 13C NMR (DMSO-d6): δ 14.69, 21.57, 111.16, 117.05, 117.23, 118.00, 122.80, 126.07, 127.56, 127.97, 129.07, 129.29, 130.04, 130.56, 130.91, 134.54, 138.03, 142.42, 145.65, 145.89, 146.81, 147.85, 154.14, 156.52, 157.19; MS: m/z 507 (M+); Anal. Calcd for C29H21N3O4S (507.56): C, 68.62; H, 4.17; N, 8.28; S, 6.32. Found: C, 68.89; H, 4.07; N, 8.16; S, 6.27%.
3-(2-Amino-3-(phenyldiazenyl)-6-tosylpyrazolo[1,5-a]pyrimidin-7-yl)-1-benzopyran-2-one (9f): Golden yellow solid, mp > 300 °C; IR (KBr) ν/cm-1: 3417, 3314 (NH2), 1602(C=N), 1352, 1142 (SO2); 1H NMR (DMSO-d6): δ 2.33 (s, 3H, CH3), 6.72-7.77 (m, 13H, ArH), 7.64 (s, 2H, NH2, D2O-exchangeable), 8.26 (s, 1H, coumarin H-4), 9.13 (s, 1H, pyrimidine H-5); 13C NMR (DMSO-d6): δ 21.15, 108.25, 115.18, 115.23, 116.02, 120.10, 122.52, 125.43, 126.79, 126.94, 128.44, 128.96, 129.88, 129.94, 130.36, 139.12, 141.90, 142.30, 144.78, 148.52, 150.52, 151.07, 156.30, 158.95; MS: m/z 536 (M+); Anal. Calcd for C28H20N6O4S (536.56): C, 62.68; H, 3.76; N, 15.66; S, 5.98. Found C, 62.92; H, 3.70; N, 15.54; S, 5.92%.
3-(2-Amino-3-[(4-tolyl)diazenyl]-6-tosylpyrazolo[1,5-a]pyrimidin-7-yl)-1-benzopyran-2-one (9g): Orange solid, mp > 300 °C; IR (KBr) ν/cm-1: 3426, 3311 (NH2), 1602(C=N), 1357, 1139 (SO2); 1H NMR (DMSO-d6): δ 2.37 (s, 3H, CH3), 2.44 (s, 3H, CH3), 7.06-7.89 (m, 12H, ArH), 7.87 (s, 2H, NH2, D2O-exchangeable), 8.29 (s, 1H, coumarin H-4), 9.12 (s, 1H, pyrimidine H-5); 13C NMR (DMSO-d6): δ 21.15, 21.56, 108.18, 114.98, 115.27, 116.85, 120.45, 123.41, 125.03, 127.71, 127.99, 128.50, 128.97, 129.04, 129.51, 131.00, 139.89, 140.85, 142.37, 145.54, 149.51, 150.52, 151.00, 157.69, 158.91; MS: m/z 550 (M+); Anal. Calcd for C29H22N6O4S (550.59): C, 63.26; H, 4.03; N, 15.26; S, 5.82. Found C, 63.53; H, 3.94; N, 15.11; S, 5.79%.
3-(2-Amino-3-[(3-trifluoromethylphenyl)diazenyl]-6-tosylpyrazolo[1,5-a]pyrimidin-7-yl)-1-benzopyran-2-one (9h): Orange solid, mp > 300 °C; IR (KBr) ν/cm-1: 3427, 3317 (NH2), 1604(C=N), 1367, 1130 (SO2); 1H NMR (DMSO-d6): δ 2.35 (s, 3H, CH3), 7.11-7.94 (m, 12H, ArH), 7.71 (s, 2H, NH2, D2O-exchangeable), 8.23 (s, 1H, coumarin H-4), 9.31 (s, 1H, pyrimidine H-5); 13C NMR (DMSO-d6): δ 21.19, 109.22, 115.14, 115.25, 118.07, 122.98, 123.45, 124.29, 127.75, 127.89, 128.98, 129.24, 129.51, 130.89, 131.00, 131.76, 139.89, 140.01, 140.85, 142.61, 145.44, 149.51, 150.87, 151.05, 157.19, 158.85; 19F NMR (DMSO-d6): δ -62.20; MS: m/z 604 (M+); Anal. Calcd for C29H19F3N6O4S (604.56): C, 57.61; H, 3.17; N, 13.90; S, 5.30. Found C, 57.90; H, 3.12; N, 13.74; S, 5.22%.
3-(2-Amino-3-((3-nitrophenyl)diazenyl)-6-tosylpyrazolo[1,5-a]pyrimidin-7-yl)-1-benzopyran-2-one (9i): Dark yellow solid, mp > 300 °C; IR (KBr) ν/cm-1: 3417, 3334 (NH2), 1602 (C=N), 1353, 1128 (SO2); 1H NMR (DMSO-d6): δ 2.31 (s, 3H, CH3), 6.95-7.94 (m, 12H, ArH), 7.89 (s, 2H, NH2, D2O-exchangeable), 8.23 (s, 1H, coumarin H-4), 9.34 (s, 1H, pyrimidine H-5); 13C NMR (DMSO-d6): δ 21.19, 108.16, 115.25, 116.11, 118.49, 122.14, 123.90, 124.45, 127.47, 127.95, 128.62, 129.02, 129.74, 130.56, 131.78, 131.90, 139.00, 140.07, 140.66, 142.23, 147.58, 149.54, 150.78, 151.40, 156.00, 158.81; MS: m/z 581 (M+); Anal. Calcd for C28H19N7O6S (581.56): C, 57.83; H, 3.29; N, 16.86; S, 5.51. Found C, 58.07; H, 3.22; N, 16.76; S, 5.44%.
ACKNOWLEDGEMENTS
This project was funded by the Deanship of Scientific Research (DSR), King Abdulaziz University, Jeddah, under grant No. (420-965-1433). The authors, therefore, acknowledge with thanks DSR technical and financial support.
References
1. M. Basanagouda, V. B. Jambagi, N. N. Barigidad, S. S. Laxmeshwar, and D. Devaru, Eur. J. Med. Chem., 2014, 74, 225. CrossRef
2. Y. Chen, Y. Lan, S. Wang, H. Zhang, X. Xu, X. Liu, M. Yu, B. Liu, and G. Zhang, Eur. J. Med. Chem., 2014, 74, 427. CrossRef
3. T. H. V. Huynh, B. Abrahamsen, K. K. Madsen, A. Gonzalez-Franquesa, A. A. Jensen, and L. Bunch, Bioorg. Med. Chem., 2012, 20, 6831. CrossRef
4. R. Aggarwal, S. Kumar, P. Kaushik, D. Kaushik, and G. K. Gupta, Eur. J. Med. Chem., 2013, 62, 508. CrossRef
5. B. Olivier, Ann. N.Y. Acad. Sci., 2004, 1036, 382. CrossRef
6. B. Olivier and R. Van Oorschot, Eur. J. Pharmacol., 2005, 526, 207. CrossRef
7. J. Gommans, T. H. Hijzen, R. A. Maes, and B. Olivier, Life Sci., 1997, 61, 11. CrossRef
8. S. Ruchirawat and T. Mutarapat, Tetrahedron Lett., 2001, 42, 1205. CrossRef
9. S. Lober, H. Hüber, and P. Gmeiner, Bioorg. Med. Chem. Lett., 2006, 16, 2955. CrossRef
10. A. Balbi, M. Anzaldi, C. Macciò, C. Aiello, M. Mazzei, R. Gangemi, P. Castagnola, M. Miele, C. Rosano, and M. Viale, Eur. J. Med. Chem., 2011, 46, 5293. CrossRef
11. R. Ragno, M. Artico, G. De Martino, G. La Regina, A. Coluccia, A. Di Pasquali and R. Silvestri, J. Med. Chem., 2005, 48, 213. CrossRef
12. R. Silvestri, M. Artico, G. La Regina, G. De Martino, M. La Colla, R. Loddo, and P. La Colla, Il Farmaco, 2004, 59, 201. CrossRef
13. C. O. Kappe and A. Stadler, Microwaves in Organic and Medicinal Chemistry; Weinheim: Wiley-VCH, 2005. CrossRef
14. C. O. Kappe, D. Dallinger, and S. S. Murphree, Practical Microwave Synthesis for Organic Chemists; Weinheim: Wiley-VCH, 2009.
15. M. R. Shaaban, T. S. Saleh, and A. M. Farag, Heterocycles, 2007, 71, 1765. CrossRef
16. M. R. Shaaban, T. S. Saleh, and A. M. Farag, Heterocycles, 2009, 78, 151. CrossRef
17. M. R. Shaaban, T. S. Saleh, and A. M. Farag, Heterocycles, 2009, 78, 699. CrossRef
18. T. S. Saleh, N. M. Abd-El-Rahman, and R. S. A. Assaker, Green Chem. Lett. Rev., 2012, 5, 315. CrossRef
19. M. R. Shaaban, T. S. Saleh, A. S. Mayhoub, and A. M. Farag, Eur. J. Med. Chem., 2011, 46, 3690. CrossRef
20. H. A. Abdel-Aziz, T. S. Saleh, and H. S. A. El-Zahabi, Arch. Pharm., 2010, 343, 24.
21. B. Stanovnik and J. Svete, Chem. Rev., 2004, 104, 2433. CrossRef
22. G. Nalajam and R. V. Rajeswar, Phosphorus, Sulfur Silicon Relat. Elem., 2010, 185, 361. CrossRef
23. P. Lue and J. V. Greenhill, Adv. Heterocycl. Chem., 1997, 67, 207. CrossRef
24. C. M. Kascheres, J. Braz. Chem. Soc., 2003, 14, 945. CrossRef
25. F. A. Rosa, P. Machado, P. S. Vargas, H. G. Bonacorso, N. Zanatta, and M. A. P. Martins, Synlett, 2008, 1673.
26. M. Calle, L. A. Calvo, A. Gonzalez-Ortega, and A. M. Gonzalez-Nogal, Tetrahedron, 2006, 62, 611. CrossRef
27. L. A. Calvo, A. M. Gonzlaez-Nogal, A. Gonzalez-Ortega, and M. C. Saӧudo, Tetrahedron Lett., 2001, 42, 8981. CrossRef
28. A. Touzot, M. Soufyane, H. Berber, L. Toupet, and C. Mirand, J. Fluorine Chem., 2004, 125, 1299. CrossRef
29. K. I. Pashkevich, V. I. Filyakova, and O. A. Kuznetsova, Russ. Chem. Bull., 1996, 45, 2868. CrossRef
30. S. G. Hegde and C. R. Jones, J. Heterocycl. Chem., 1993, 30, 1501. CrossRef
31. C. P. Frizzo, M. R. P. Marzari, L. Buriol, D. N. Moreira, F. A. Rosa, P. S. Vargas, N. Zanatta, H. G. Bonacorso, and M. A. P. Martins, Catal. Commun., 2009, 10, 1967. CrossRef
32. M. Takahashi, T. Mamiya, H. Hasegawa, T. Nagai, and H. Wakiya, J. Heterocycl. Chem., 1986, 23, 1363. CrossRef
33. A. E. M. Mekky, T. S. Saleh, and A. S. Al-Bogami, Tetrahedron, 2013, 69, 6787. CrossRef
34. H. F. Anwar and M. H. Elnagdi, ARKIVOC, 2009, i, 198.
35. H. Behbehani, H. M. Ibrahim, and S. Makhseed, ARKIVOC, 2010, ii, 267.
36. K. M. Al-Zaydi, M. A. Al-Shiek, and E. A. Hafez, J. Chem. Res., 2000, 13. CrossRef
37. M. R. Shaaban, J. Fluorine Chem., 2008, 129, 1156. CrossRef
38. D. Sanz, R. M. Claramunt, A. Saini, V. Kumar, R. Aggarwal, S. P. Singh, I. Alkorta, and J. Elguero, Magn. Reson. Chem., 2007, 45, 513. CrossRef
39. M. Elyashberg, K. Blinov, and A. Williams, Magn. Reson. Chem., 2009, 47, 371. CrossRef
40. A. Takamizawa and Y. Hamashima, Yakugaku Zasshi, 1964, 84, 1113.
41. M. H. Elnagdi, H. A. Elfahham, S. A. S. Ghozlan, and G. E. Elgemeie, J. Chem. Soc., Perkin Trans.1, 1982, 2663