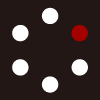
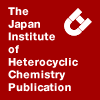
HETEROCYCLES
An International Journal for Reviews and Communications in Heterocyclic ChemistryWeb Edition ISSN: 1881-0942
Published online by The Japan Institute of Heterocyclic Chemistry
e-Journal
Full Text HTML
Received, 22nd June, 2015, Accepted, 14th September, 2015, Published online, 5th October, 2015.
■ Ring Opening Ring Closure Reactions with 3-Substituted Chromones under Nucleophilic Conditions
Magdy A. Ibrahim,* Nasser M. El-Gohary, and Sara Said
Department of Chemistry, Faculty of Education, Ain Shams University, Roxy, Cairo 11711, Egypt
Abstract
This review covers the ring opening ring closure (RORC) reactions of 3-substituted chromones with a variety of nitrogen and carbon nucleophiles. The nucleophilic reagent usually attack 3-substituted chromones at the C-2 position with γ-pyrone ring opening followed by further transformation during the course of the reaction producing a variety of products depending on the substrate at position 3, the nature of nucleophile and the reaction conditions.CONTENTS
1. Introduction
2. RORC reactions with chromone-3-carbonitriles
3. RORC reactions with chromone-3- carboxamides
4. RORC reactions with chromone-3- carboxylic acids
5. RORC reactions with chromone-3- carboxylates
6. RORC reactions with 3-halochromones
7. RORC reactions with 3-nitrochromones
8. Conclusion
9. References
1. INTRODUCTION
Chromones constitute one of the major classes of naturally occurring compounds,1 and they are useful as biologically active agents.2-6 The chromone moiety is an essential pharmacophore of a large number of bioactive molecules.7-9 The biological activity of chromone derivatives include cytotoxic (anticancer).10-13 neuroprotective,14,15 HIV-inhibitory,16,17 antimicrobial,18-20 antifungal,21 anti-inflammatory,22 antiplatelet,23 antidiabetics,24 antitumor,25 antiviral,6 and antioxidant activity.26 Also, chromones possess a broad diversity in treatment of ulcers,27 and schizophrenia.28 Due to their abundance in plants and their low mammalian toxicity, chromone derivatives are present in large amounts in the diet of humans.29,30 3-Substituted chromones are very active substrates toward nucleophilic reagents. The chemical reactivity of 3-substituted chromones is widely different depending on the nature of the functional group present at position 3, nature of nucleophile and the reaction conditions. Among the 3-functionalized chromones, their 3-formyl derivatives are widely used in heterocyclic synthesis. Several reviews in the chemistry of 3-formylchromones are published.31-36 Herein, the present review summarize the ring opening ring closure (RORC) reactions of other 3-substituted chromones with a variety of nitrogen and carbon nucleophiles. Introduction of electron-withdrawing [CN, CONH2, CO2H, CO2R, NO2, X (Cl, Br or I)] group at the 3-position of a chromone system increase significantly the reactivity of the γ-pyrone ring with respect to nucleophiles, and provides a broad synthetic potential for 3-substituted chromones.
2. RORC REACTIONS WITH CHROMONE-3-CARBONITRILES
Chromone-3-carbonitriles 1 being an α,β-unsaturated ketone and possess three electron deficient sites viz. C-2, cyano carbon and carbonyl carbon, the last one having obviously the least electrophilicity compared to the other two carbons.37 An initial addition of the nucleophile to carbonitriles 1 and any subsequent transformations, if possible, of the adducts depend on the nature of the nucleophile as well as the reaction conditions.
Among the diverse transformations of carbonitriles 1, one of the most important, is their conversions on heating with morpholine in an aqueous DMF,38 or with n-propylamine in an aqueous ethanol,39 or with concentrated ammonia,40 or with aqueous NaOH solution,41 into 2-amino-3-formylchromones 2, via the intermediate A (Scheme 1). This transformation was achieved via γ-pyrone ring opening followed by recyclization; in other words, the chromone-3-carbonitriles 1 are 'chemically equivalent' to 2-amino-3-formylchromones 2 under certain reaction conditions.
In marked contrast to the known behavior of chromone-3-carbonitriles 1, 2-trifluoromethyl-chromone-3-carbonitrile (3) under the same basic conditions undergoes facile detrifluoroacetylation giving either 2-aminochromone (4) with morpholine in an aqueous DMF, and salicyloylacetonitrile (5) with aqueous NaOH or DMSO/H2O. The latter readily isomerizes on heating into 2-aminochromone 4 (Scheme 2).42
Chromone-3-carbonitriles 1 when refluxed in ammonium acetate and acetic acid underwent self-condensation, through its tautomeric forms 2, yielding 2-(chromen-3-yl)chromono[2,3-d]pyrimidines 6, via the intermediate B (Scheme 3).39,43
Carbonitrile 1 dissolved in acetic acid reacted with aqueous sodium hypochlorite solution producing 1,2-addition product 7 which upon hydrolysis with aqueous acetic acid in the presence of sodium acetate yielded the rearranged product, 2-amino-3-chlorochromone (8) (Scheme 4).44
Diazomethane undergoes [3+2] cycloaddition with the 2,3-olefinic bond of carbonitrile 1 giving the 1-pyrazoline intermediate 9 that by a concerted electrocyclic elimination of nitrogen molecule and migration of hydrogen yielded 3-cyano-2-methylchromone (10); base catalyzed Michael addition of compound 10 to the α,β-unsaturated keto function of a second molecule of compound 10 gave the dimeric product 11, diazomethane or the pyrazoline 9 act as a base in the latter reaction (Scheme 5).45
Reactions of carbonitrile 1 with primary aromatic amines in boiling benzene gave a mixtures of Z- and E- 3-arylamino-2-(2-hydroxyaroyl)acrylonitriles 12 and 2-amino-3-(aryliminomethyl)chromones 13 (Scheme 6). The latter compounds 13 can easily be obtained in the individual state when the reaction was carried out in the presence of triethylamine, which accelerates the cyclization step via deprotonation of the phenolic hydroxyl group. In the case of primary aliphatic amines, the open chain product 12 immediately undergoes cyclization into 3-alkyliminomethyl-2-aminochromones 13 in good yields.46-48
The reaction of carbonitrile 1 with ethylenediamine in boiling ethanol was firstly studied by Ghosh and Tewari,39 and isolate 1-(2-hydroxyphenyl)-2-imidazolid-2-ylidene)ethanone (14) (15%) together with 2-amino-3-formylchromone 2 (45%); when the reaction was performed in boiling ethanol for 3 h in 1:1 molar ratio (Scheme 7). While, Ghosh et al.49 postulated the formation of bis-chromeno[2,3-b:2',3'-f]- [1,5]diazocine (15) when the reaction was carried in boiling ethanol for 10 min in 2:1 molar ratio. Hydrolysis of compound 15 under acidic conditions afforded compound 2 (Scheme 7), in this reaction ethylenediamine, as aliphatic amine, induced self-condensation of carbonitrile 1.
The previous reaction was next studied by Sosnovskikh et al.50 and isolate N`,N`-ethylene-bis(2-amino-3-iminomethylchromones) 16, when the reaction was performed in boiling ethanol for 10 min in 1:1 molar ratio. Depending on the time of refluxing in acetic acid, the later compound gave either 2-amino-3-formylchromones 2 or the products of their dimerization, 2-(chromen-3-yl)-5H-chromeno[2,3-d]- pyrimidin-5-ones 6 (Scheme 8).50
Also, there is contradictory information in literature regarding the structure of the product obtained from the reaction between carbonitrile 1 and o-phenylenediamine. Hence, Ghosh and Tewari,39 postulate the formation of chromeno[2,3-b][1,5]benzodiazepines 17. While, Risitano and his coworkers 51 repeated the reaction and postulate the formation of benzodiazepines 18. Next, the reaction was reinvestigated and the product was expected to be 3-(benzimidazol-2-yl)chromones 19 (Scheme 9).52
Similar to the above behavior, reaction of carbonitriles 1 (R=H, CH3) with 1,6-diaminopyridones 20 in boiling DMF containing triethylamine produced pyridotriazepine derivatives 21,53,54 these products were assumed to be the Schiff bases 22 as published by Sosnovskikh and Moshkin (Scheme 10).55
Recently, the chemical transformations 6-methylchromone-3-carbonitrile (1) was studied towards a variety of heterocyclic nitrogen binucleophiles. Thus, treatment of carbonitrile 1 (R= Me) with 3-amino-1,2,4-triazole in absolute ethanol under reflux afforded 5-amino-6-(2-hydroxy-5-methylbenzoyl)- [1,2,4]triazolo[1,5-a]pyrimidine (23), via intermediate C, as depicted in Scheme 11. Similarly, reaction of carbonitrile 1 (R=Me) with 2-aminobenzimidazole in boiling ethanol yielded pyrimido[1,2-a]- benzimidazole derivative 24. These reactions take place via γ-pyrone ring opening followed by intramolecular cycloaddition onto the nitrile function. (Scheme 11).56
Previously, the reactions of carbonitriles 1 with hydrazine hydrate and phenylhydrazine were believed to occur via 1,2-addition at the cyano group of carbonitrile 1. Hydrazine undergoes 1,2-addition to the nitrile function in carbonitriles 1 in ethanol under reflux and the resultant iminohydrazine intermediate 25 (R=H; non-isolable) cyclized to the fused pyrazole 26.57 In case of phenylhydrazine, under similar conditions the iminiohydrazine 25 (R=Ph; isolable) was obtained and underwent, upon further heating in ethanol, intramolecular γ-pyrone ring opening producing benzoylpyrazole derivative 27 (Scheme 12).57
The previous reactions were reinvestigated by Sosnovskikh et al.58 and different products were isolated depending on the reaction conditions. Reactions of carbonitriles 1 with phenylhydrazine gave the corresponding 5-amino-4-salicyloyl-1-phenylpyrazoles 28 (in ethanol), 2-aminochromone-3-carboxaldehyde-N-phenyl-hydrazones 29 (in benzene/TEA), and 1-phenylchromeno[4,3-c]pyrazol-4(1H)-ones 30 (in acetic acid), (Scheme 13).
Carbonitriles 1 reacted differently with methylhydrazine giving 3-(2-hydroxyaryl)-1-methylpyrazole-4-carbonitriles 31 (in benzene) and 2-methylchromeno[4,3-c]pyrazol-4(2H)-ones 32 (in acetic acid). Boiling pyrazole 31 in acetic acid produced compound 32 (Scheme 14).58
Treating carbonitrile 1 with 7-chloro-4-hydrazinoquinoline and 3-hydrazino-5,6-diphenyl-1,2,4-triazine in refluxing ethanol led to the quinolinylpyrazole 33 and 1,2,4-triazinylpyrazole 34, respectively (Scheme 15).56
Previously, reaction of carbonitrile 1 and hydroxylamine hydrochloride in boiling ethanol was believed to occur via 1,2-addition onto the nitrile function to produce the 1,2-adduct 35 which undergoes no further transformation.57 A Polish group59,60 reported the formation of oxime 36 and pyrazolinone 37 by reaction of carbonitrile 1 with an aqueous solution of hydroxylamine hydrochloride and sodium hydroxide (Scheme 16). The reaction of carbonitrile 1 with hydroxylamine hydrochloride was repeated by Sosnovskikh et al.61 and found the product was, in fact, 2-amino-3-carbamoylchromone (38) (Scheme 16).
Reaction of carbonitriles 1 with guanidine carbonate in boiling ethanol afforded 2-aminochromeno[4,3-d]pyrimidin-4(4H)-ones 39 (Scheme 17).50
Heteroannulated chromones showed significant biological activity including pharmacological, anti-inflammatory and antiplatelet activities.62 Chromone-3-carbonitriles 1 are useful intermediates in the synthesis of chromeno[2,3-b]pyridines 40 (trivial name: azaxanthones) with anti-inflammatory activity.63 Reactions of carbonitriles 1 with some active methyl and methylene compounds were studied and a variety of chromeno[2,3-b]pyridines and related compounds were efficiently synthesized.64 Condensation reaction of carbonitrile 1 with some acetyl heterocycles namely; 2-acetylthiophene, 3-acetylpyridine, 5-acetyl-4-hydroxy-2H-1,3-thiazine-2,6(3H)-dione and 3-acetyl-4-hydroxy-6-methyl-2H-pyran-2-one, in absolute ethanol containing few drops of 1,8-diazabicyclo[5.4.0]undec-7-ene (DBU) as a basic catalyst, afforded 2-heteroaryl-5-oxo-5H-chromeno[2,3-b]pyridines 40, via the non isolable intermediate D, as described by Ibrahim (Scheme 18).64 The transformation of carbonitrile 1 into chromeno[2,3-b]pyridines 40 can be regarded as a domino "Michael/retro-Michael/nitrile-addition/cyclocondensation".
In a similar manner, condensation of carbonitriles 1 with 4,6-diacetylresorcinol in 1:1 and 2:1 molar ratios gave chromeno[2,3-b]pyridines 41 and 42, respectively. 4,6-Bis(5-oxo-5H-chromeno[2,3-b]pyridin-2-yl)- resorcinols 42 were also obtained from the interaction of compounds 41 with carbonitriles 1 under the same basic conditions (Scheme 19).64,65
Various 2-amino-5-oxo-5H-chromeno[2,3-b]pyridines 43 bearing different substituents at position 3 were synthesized from the reaction of carbonitrile 1 with some active methylenenitriles (-CH2CN) namely: malononitrile, cyanoacetamide, N-benzyl-2-cyanoacetamide, N-phenyl-2-cyanoacetamide, ethyl cyanoacetate, phenylacetonitrile, and phenylthioacetonitrile in ethanol containing few drops of DBU (Scheme 20).65,66
Chromeno[2,3-b]pyridine 43 (R`= CN) was used as a good precursor to synthesize a novel series of heteroannulated chromones identified as chromeno[3',2':5,6]pyrido[2,3-d]pyrimidines.66 Consequently, condensation of compound 43 (R`= CN) with formamide, formic acid and acetic anhydride under fusion conditions afforded 8-methyl-6-oxo-6H-chromeno[3',2':5,6]pyrido[2,3-d] pyrimidines 44-46, respectively (Scheme 21).
Heterocyclization of compound 43 (R`=CONHCH2Ph) with acetic anhydride afforded 3-benzyl-2,8-dimethyl-4H,6H-chromeno[3',2':5,6]pyrido[2,3-d]pyrimidine-4,6-dione (47) (Scheme 22).66
On the other hand, reaction of carbonitrile 1 (R=H) with N`-[(4-chlorophenyl)methylidene]-2-cyanoacetohydrazide (48) in boiling ethanol containing DBU yielded the expected 2-amino-5-oxo-5H-chromeno[2,3-b]pyridine-3-carbohydrazide (49), via intermediates E and F.64 While, the unexpected benzoxocine derivatives 50 were obtained from the reaction of carbonitrile 1 (R=CH3) with N`-[(4-chloro/methoxyphenyl)methylidene]-2-cyanoacetohydrazide (48) under the same reaction conditions (Scheme 23).56,66 Formation of benzoxocine derivatives 50 may occur via the formation of intermediate E followed by an intramolecular nucleophilic addition of the hydroxyl group onto the nitrile function as shown in Scheme 23.
Treatment of carbonitrile 1 (R= Me) with malononitrile dimer (2-aminoprop-1-ene-1,1,3-tricarbonitrile) in boiling ethanol containing DBU gave (3-cyano-9-methyl-5-oxo-1,5-dihydro-2H-chromeno[4,3-b]- pyridin-2-ylidene)-propanedinitrile (51).67 Another expected product, 2,4-diamino-8-methyl-6-oxo-6H-chromeno[2,3-b][1,8]naphthyridine-3-carbonitrile (52) was ruled out (Scheme 24). Compound 52 was efficiently synthesized using an alternative pathway via the reaction of 2-amino-6-methylchromone-3-carboxaldehyde (2) with malononitrile dimer in boiling ethanol containing few drops of DBU.
Reaction of carbonitrile 1 (R=H) with benzimidazol-2-ylacetonitrile (53) in boiling ethanol containing few drops of triethylamine, gave the angular heteroannulated chromone; chromeno[2,3:6,5]pyrido[1,2-a]benzimidazole-6-carbonitrile 54 (Scheme 25). While, the reaction of 6-methylchromone-3-carbonitrile (1; R=Me) with benzimidazol-2-ylacetonitrile (53) showed different behavior and the reaction proceeds in a different mechanism producing 2-amino-3-(1H-benzimidazol-2-yl)-7-methyl-5H-chromeno[2,3-b]- pyridin-5-one (55) (Scheme 25).68
Reaction of carbonitriles 1 with some symmetrical and unsymmetrical active methylene ketones namely; acetylacetone, dibenzoylmethane, deoxybenzoin, ethyl acetocetate, ethyl benzoylacetone, diethyl malonate, acetoacetanilide, dimethyl β-ketoglutarate and diethyl β-ketoadipate afforded 2,3-disubstituted-5-oxo-5H-chromeno[2,3-b]pyridines 56 (Scheme 26).64,66,69-75 Recently, some chromeno[2,3-b]pyridines 56, with antioxidant activity, were synthesized in high yields (78-86%) using ultra sound irradiation.66
Oxazine 57 was obtained, from reaction of carbonitrile 1 with Z-isomer of dimethyl β-keto-α-oximino-glutarate, in boiling ethanol containing triethylamine (Scheme 27).71
Cyclic α-methylene ketones and cyclic 1,3-diketones also undergo smooth and efficient ring opening and ring closure (RORC) for carbonitrile 1 yielding heteroannulated chromone derivatives.64 Thus, reaction of carbonitrile 1 with cyclopentanone, 2-phenyliminothiazolidin-4-one, pyrazoline-3,5-dione, 5,5-dimethylcyclohexane-1,3-dione, barbituric and thiobarbituric acids afforded a series of tetracyclic systems 58-62, respectively (Scheme 28).64
The reaction of carbonitrile 1 with N-benzoylglycine, "hippuric acid", in acetic anhydride was reported to chromenopyridine derivative 63 as assigned by Ghosh and Tewari,70 while the same reaction produced chromenopyridine derivative 64 as suggested by Ibrahim64 (Scheme 29). On the other hand, boiling carbonitrile 1 with N-acetylglycine, "aceturic acid", and N-benzoylglycine, "hippuric acid", in acetic anhydride containing in the presence of freshly fused sodium acetate, the tetracyclic systems 65 was obtained (Scheme 29).39, 64,70
Treating carbonitrile 1 with 3-nitroacetylquinolinone 66 in boiling DMF containing DBU as a catalyst afforded quinolinone derivative bearing chromeno[3,2-b]pyridine 67 (Scheme 30).75
Moreover, treating carbonitrile 1 (R=H, 8-allyl)) with β-ketoacid 68 in boiling DMF containing DBU afforded pyranoquinoline derivatives 69 (Scheme 31).76
Lactone 70 was obtained (30% yield) by reacting carbonitrile 1 with 4-hydroxycoumarin, in refluxing ethanol containing piperidine (Scheme 32).77 Schurreit78 has reported without giving any mechanism the formation of compound 71 (18% yield) by refluxing three molecules of carbonitrile 1 and 4-hydroxycoumarin under the same reaction conditions; 4-hydroxycoumarin having no role in the formation of compound 71. Compound 71 when refluxed with 30% hydrogen peroxide in trifluoroacetic acid is converted into the lactone 70 (Scheme 32).
The chemical reactivity of chromone-3-carbonitrile (1) towards 1,2-, 1,3- and 1,4-cyclohexanediones was studied under different molar ratios. Three isomeric products of angular bis[1]chromenophenanthrolines were isolated.79 Thus, base catalyzed reaction of carbonitrile 1 with 1,2-cyclohexanedione in 1:1 and 2:1 molar ratio resulted in the formation of the angular heptacyclic system, 7,8-dihydro-5H,10H-bis[1]chromeno[2,3-b:3`,2`-J][1,10]phenanthroline-5,10-dione (73). Isolation of chromeno[2,3-b]- quinolinedione derivative 72 was failed (Scheme 33).
On the other hand, condensation of carbonitrile 1 with 1,3-cyclohexanedione, in absolute ethanol containing DBU, in 1:1 molar ratio resulted in the formation of chromeno[2,3-b]quinolinedione derivative 74 (Scheme 34). When the latter reaction occurs in 2:1 (carbonitrile 1: 1,3-cyclohexanedione) molar ratio resulted in the formation of the angular heptafused system 75 containing two chromeno[2,3-b]pyridine moieties. Compound 75 was also obtained authentically from the condensation of compound 74 with carbonitrile 1.79
Next, condensation of carbonitrile 1 with 1,4-cyclohexanedione in 1:1 and 2:1 molar ratio resulted in the formation of the angular heptacyclic system, 7,8-dihydro-15H,18H-bis[1]chromeno[3,2-b:2`,3`-J][4,7]- phenanthroline-15,18-dione (76), via the non-isolable chromeno[2,3-b]quinolinedione derivative (Scheme 35).79
3. RORC REACTIONS WITH CHROMONE-3-CARBOXAMIDES
Chromone-3-carboxamide (77) has three electron deficient centers, C-2, C-4, and the amidic carbon at position 3. The nucleophilic reagent usually attack at the C-2 position with γ-pyrone ring opening followed by further transformation during the course of the reaction producing a variety of products depending on the nucleophile used. The chemistry of chromone-3-carboxamide (77) was studied in details by Ibrahim.80,81 Reactions of chromone-3-carboxamide (77) with different nucleophiles resulted in the conversion of γ-pyrone ring into α-pyrone; on other words conversion of chromone into coumarin.
Treatment of carboxamide 77 with aqueous 1 M NaOH solution resulted in the facile rearrangement to 4-hydroxycoumarin-3-carboxaldehyde (78), while its reaction with concentrated ammonium hydroxide solution produced 3-aminomethylene-2H-chroman-2,4-dione (79) via ring opening followed by ring closing (RORC) reactions (Scheme 36).80
Treatment of carboxamide 77 with some primary aliphatic and aromatic amines namely; methylamine, ethylamine, n-propylamine, benzylamine, p-bromoaniline and p-toluidine, resulted in ring transformation via γ-pyrone ring opening followed by lactonization with loss of ammonia to afford the corresponding geometrical isomeric chromane-2,4-diones 80 (Scheme 37).80,81 The ratio of Z:E isomers depend on the type of amine used.
Moreover, the chemical reactivity of carboxamide 77 was studied towards a variety of 1,2-binucleophiles. Condensation of carboxamide 77 with hydroxylamine hydrochloride in refluxing DMF produced chromeno[3,4-d]isoxazol-4(4H)-one (81) (Scheme 38).81
Condensation of carboxamide 77 with hydrazine hydrate and phenylhydrazine in refluxing ethanol achieved ring transformation of carboxamide 77 producing chromeno[4,3-c]pyrazoles 82 (R=H, Ph). While, reaction of carboxamide 77 with 7-chloro-4-hydrazinoquinoline in boiling ethanol afforded the hydrazone derivative 83 (Scheme 39).81
Condensation of carboxamide 77 with guanidine hydrochloride, cyanoguanidine and thiourea in ethanolic potassium hydroxide solution produced chromeno[4,3-d]pyrimidine derivatives 84 (X= NH, N-CN, S) (Scheme 40).81
Treatment of carboxamide 77 with o-phenylenedimine and o-aminophenol in refluxing DMF afforded chromane-2,4-dione derivatives 85 (X= NH, O), as Z isomers. While, treatment of carboxamide 77 with o-aminothiophenol in refluxing DMF achieved ring transformation producing benzothiazolylcoumarin derivative 86 (Scheme 41).81
Ethylenediamine showed different behavior when reacted with carboxamide 77 producing the bis- enaminone derivative 87 (Scheme 42).81
Furthermore, the chemical reactivity of carboxamide 77 was studied towards a variety of carbon nucleophiles. Treatment of carboxamide 77 with malononitrile and cyanoacetamide in boiling ethanol containing piperidine afforded the pyridine derivatives 88 as depicted in Scheme 43.81
The reaction of carboxamide 77 with acetophenone and thiobarbituric acid in ethanolic potassium hydroxide solution gave the corresponding α,β-unsaturated ketone 89 and pyrimidine derivative 90, respectively (Scheme 44).81
Treating chromone-3-carboxamides 77 (R=H, Me) with 1H-benzimidazol-2-ylacetonitrile, in boiling ethanol containing triethylamine, produced pyrido[1,2-a]benzimidazoles 91 (Scheme 45).68
Heteroannulated chromone, namely, 3-(2-hydroxybenzoyl)-2H-chromeno[2,3-b]pyridine-2,5(1H)-dione (92) was obtained from dimerization of carboxamide 77 with sodium methoxide solution (Scheme 46).80
4. RORC REACTIONS WITH CHROMONE-3-CARBOXYLIC ACIDS
The chemistry of nucleophilic reactions involving RORC of chromone-3-carboxylic acid (93) attracted attentions due to rare literature reports. Also, in these very few reports we face some encountering results.82-84 The product obtained from the reaction of carboxylic acid 93 with phenylhydrazine had been established to be 4-oxo-1-phenylchromeno[4,3-c]pyrazole (30),82 not the corresponding isomer 2-phenyl-chromeno[4,3-c]pyrazol-3(2H)-one (94) as previously reported by Ghosh and Mukhopadhyay (Scheme 47).83 Also, reaction of carboxylic acid 93 with hydroxylamine hydrochloride was reported to give 5-oxo-4,5-dihydro-1,4-benzoxazepine-3-carboxylic acid (95).83 However, Chantegrel et al. found the same reaction gave 5-(2-hydroxyphenyl)isoxazole (96).84 Reaction of carboxylic acid 93 with guanidine carbonate gave 2-aminochromeno[4,3-d]pyrimidin-4(4H)-one (39) not the corresponding isomer 97 (Scheme 47) as published by Chantegrel et al.84
The chemistry of chromone-3-carboxylic acid (93) was studied in details by Ibrahim.85 Carboxylic acid 93 in the presence of sodium hydroxide solution undergoes different reactions depending on the concentration of sodium hydroxide and reaction conditions. Treatment of carboxylic acid 93 with 0.025 M sodium hydroxide solution in refluxing ethanol afforded ω-formyl-2-hydroxyacetophenone (98), while when the reaction carried out using 0.05 M aqueous sodium hydroxide solution at 70 ºC afforded 1-(3-chromonyl)-2-(2-hydroxybenzoyl)ethene (99) (Scheme 48). Reaction of carboxylic acid 93 with benzaldehyde in ethanolic sodium hydroxide solution (0.025 M) afforded 3-(α-hydroxybenzyl)chromone (100) (Scheme 48).85
Reaction of carboxylic acid 93 with primary and secondary amines in ethanol afforded the enaminones 101, when carboxylic acid 93 was allowed to react with aniline and n-propylamine in glacial acetic acid produced 3-(phenyl/n-propyl)aminomethylenechromane-2,4-diones 102 as stereoisomeric (Z and E) mixtures (Scheme 49).85 These results confirm the loss of CO2 molecule after opening of pyrone ring in ethanol but no decarboxylation occurred in acetic acid medium.
3-[N`-(7-Chloroquinolin-4-yl)hydrazino]-1-(2-hydroxyphenyl)-prop-2-en-1-one (103) and 1-(7-chloroquinolin-4-yl)chromeno[4,3-c]pyrazol-4(1H)-one (104) were obtained from the reaction of carboxylic acid 93 with 7-chloro-4-hydrazinoquinoline in ethanol and acetic acid, respectively. Cyclization of compound 103 in DMF afforded the pyrazole derivative 105 (Scheme 50).85
Reaction of carboxylic acid 93 with cyanoacetohydrazide in ethanol and/or acetic acid gave compounds 106 and 30, respectively. When the later reaction took place in sodium ethoxide, the diazepine derivative 107 was isolated (Scheme 51). Compounds 106, 30 and 107 are produced during the ring transformation because cyanoacetohydrazide thereby acts as an ambient nucleophile, that is, as both N- and C-nucleophile.85 Refluxing compound 106 in dimethylformamide afforded the well known 3-(2-hydroxyphenyl)-1H-pyrazole (108).43 Compounds 30 and 108 were also obtained from the reaction of carboxylic acid 93 with hydrazine hydrate in acetic acid and ethanol, respectively (Scheme 51).85
Reaction of chromone-3-carboxylic acid (93) with some carbon nucleophiles was studied. Reaction of carboxylic acid 93 with some acyclic active methylene nitriles namely; malononitrile, ethyl cyanoacetate, chloroacetonitrile and benzyl cyanide in absolute ethanol containing few drops of triethylamine led to the expansion of γ-pyrone ring in chromone-3-carboxylic acid (93) affording 2-amino-3-substituted-6H-benzoxocin-6-ones 110–113, respectively (Scheme 52).85,86 This transformation occurred by way a domino "Michael /retro-Micheal/ring opening/ decarboxylation/cycloaddition" reaction.
On the other hand, reaction of carboxylic acid 93 with ethyl cyanoacetate in absolute ethanol containing few drops of piperidine and morpholine afforded 2-amino-3-(piperidin/morpholin-1-ylcarbonyl)-6H-1-benzoxocin-6-ones 114 and 115, respectively (Scheme 53). Compounds 114 and 115 were also synthesized from the reaction of ethyl ester 111 with equivalent amounts of piperidine and morpholine in boiling ethanol.86
Treatment of compound 93 with cyanoacetamide in ethanol containing few drops of triethylamine afforded 6-(2-hydroxyphenyl)-2-oxo-1,2-dihydropyridine-3-carbonitrile (116) (Scheme 54).85 Under similar conditions, treatment of carboxylic acids 93 (R=H, Me) with benzimidazol-2-ylacetonitrile, in boiling ethanol containing few drops of triethylamine as a basic catalyst, afforded the pyrido[1,2-a]- benzimidazole-4-carbonitriles 117 (Scheme 54).68
The chemical behavior of chromone-3-carboxylic acid (93) towards some acyclic active methylene ketones was studied. Boiling carboxylic acid 93 with acetylacetone and ethyl acetoacetate in absolute ethanol containing few drops of triethylamine afforded the corresponding 2-methyl-3-substituted-6H-1-benzoxocin-6-ones 118 and 119, respectively (Scheme 55).86
Interestingly, it was found that chromone-3-carboxylic acid (93) showed unexpected behavior towards diethyl malonate than the previous acylic active methylene compounds. Refluxing an equimolar amounts of carboxylic acid 93 with diethyl malonate in absolute ethanol containing few drops of triethylamine produced 2-(2-hydroxyphenyl)-4H,5H-pyrano[2,3-b]chromen-5-one (118) (Scheme 56). The expected benzoxocinone derivative 119 was excluded.86
In most of the previous mentioned reactions, the -pyrone ring in chromone-3-carboxylic acid (93) was expanded to oxocinone ring upon its reaction with acyclic active methylene compounds to produce 6H-benzoxocin-6-one derivatives.
Then, the chemical behavior of chromone-3-carboxylic acid (93) towards some cyclic active methylene compounds. Thus, treatment of carboxylic acid 93 with dimedone produced 2-(2-hydroxyphenyl)-7,7-dimethyl-6,7-dihydrochromen-5-one (120) and not the ring expanded product, 2,2-dimethyl-2H-dibenzo[b,g]oxocine-4,7(1H,3H)-dione (121) as depicted in Scheme 57.86
The reaction between carboxylic acid 93 and some heterocycles containing active methylene group was studied. Surprisingly, heating an ethanolic solution of the carboxylic acid 93 with 1,3-diphenyl-1H-pyrazol-5(4H)-one and thiobarbituric acid under reflux produced the novel unexpected products identified as 1,3-diphenyl-5-ethoxy-5H-chromeno[3`,4`:5,6]pyrano[2,3-c]pyrazol-4 (1H)-one (122) and 6-ethoxy-2-thioxo-2H,6H-chromeno[3`,4`:5,6]pyrano[2,3-d]pyrimidine-4,5-(1H,3H)-dione (123), respectively (Scheme 58).86
5. RORC REACTIONS WITH CHROMONE-3-CARBOXYLATES
The chemical reactions of chromone-3-carboxylates with nucleophilic reagents are rare. Basic rearrangement of ethyl chromone-3-carboxylates 124 in 1 N aqueous sodium hydroxide solution at room temperature produced 3-substituted-4-hydroxycoumarins 125 in good yield (78-86%) (Scheme 59).87
An analogous transformation was observed when ethyl chromone-3-carboxylate (96) was heated with concentrated ammonium hydroxide solution, giving rise 3-(formimidoyl)-4-hydroxycoumarin (126) (Scheme 60).87
Similarly, heating ethyl 2-methylchromone-3-carboxylate (124) with aqueous ammonia solution afforded 3-acetimidoyl-4-hydroxycoumarin (127) via ring opening ring closure reaction (Scheme 61).88
Boiling chromone-3-carboxylate derivative 128 in aqueous ammonia solution yielded 3-acetimidoyl-4-hydroxy-5-methoxy-6,7,8-trifluorocoumarin (129) which upon heating with sulfuric acid afforded 3-acetylcoumarin analog 130 (Scheme 62).89
Also, reaction of chromone-3-carboxylate 124 with methylhydrazine produced substituted pyrazole 131 and a mixture of chromeno[4,3-c]pyrazoles 132 and 133 (Scheme 63).90
On the other hand, methyl 5-(2-hydroxyphenyl)-1-(pyridin-2-yl)-1H-pyrazole-4-carboxylate (134) was synthesized in good yield (61%) from the reaction of chromone-3-carboxylate 124 with 2-hydrazinopyridine in boiling ethanol. Compound 134 underwent an intramolecular lactonization under the influence of methylhydrazine producing chromeno[4,3-c]pyrazol-4-one 135 (Scheme 64).91
Treatment of methyl ester 124 with phosphorhydrazides afforded chromane-2,4-diones 136 via γ-pyrone ring opening followed by lactonization (Scheme 65).92
6. RORC REACTIONS WITH 3-HALOCHROMONES
Reaction of 3-bromochromone (137, X=Br) with equimolar amount of dimethyl acetonedicarboxylate in tetrahydrofuran (THF) in the presence of DBU as a basic catalyst afforded methyl furoate derivative 138 in 19% yield (expected product) along with furylcyclopropylchromene derivative 139 in 42% yield (unexpected product) (Scheme 66).93
Gammill and his coworkers94 described the reaction of 3-bromochromone (137, X=Br) with amines and found that; the primary amines gave the ring contraction products 140, while secondary amines gave 3-aminochromone (141). However, Huang and his coworkers95 found that secondary amines gave also the ring contraction products (Scheme 67).
Reaction of 3-bromochromone (137, X=Br) with ethyl acetoacetate in basic medium resulted in rupture of the γ-pyrone ring producing furan derivative 142 (Scheme 68).94
Addition of nitroethane to 3-bromochromone (137, X=Br) in acetonitrile in the presence of DBU as a base gave 3-hydroxy-2-[1-(hydroxyimino)methyl]chromone (143) in 71% yield. Treatment of compound 143 with N,N-dimethylformamide dimethyl acetal (DMF-DMA) afforded the isoxazole derivative 144 in 76% yield (Scheme 69).96
Treatment of 3-iodochromone (137, X=I) with 2-mercaptobenzimidazole in the presence of potassium carbonate in DMF at room temperature gave a mixture of 3-(1H-benzimidazol-2-yl-thio)chromone (145) and benzimidazo[2,1-b]thiazole derivative 146 (Scheme 70).97
Reaction of 3-chloro-2-(polyfluoroalkyl)chromones 147 with hydrazine hydrate hydrochloride in boiling ethanol afforded 4-chloro-3-(2-hydroxyphenyl)-pyrazoles 148 (Scheme 71).98
On the other hand, reaction of 3-chloro-2-(polyfluoroalkyl)chromones 147 with hydroxylamine hydrochloride in boiling ethanol in the presence of sodium acetate produced benzofuran derivative 149 in high yield (58-91%) instead of isoxazoles 150 (Scheme 72).99
A highly efficient synthesis of furo[3,2-c]coumarins 152 was achieved via base catalyzed reaction of 3-halochromones 124 (X=Br, Cl) with 4-hydroxycoumarin100 or 2-aminochromone (151) (Scheme 73).101
7. RORC REACTIONS WITH 3-NITROCHROMONES
Reaction of 3-nitrochromone (153) with phenylhydrazine gave 5-(2-hydroxyphenyl)-4-nitro-1-phenylpyrazole (154),102 while its reaction with methylhydrazine in boiling ethanol afforded the isomeric pyrazole derivatives 155 and 156 (Scheme 74).103 Also, treatment of compound 153 with benzimidine in boiling ethanol containing triethylamine gave 6-(2-hydroxyphenyl)-5-nitro-2-phenylpyrimidine (157) (Scheme 74).102
Treatment of 3-nitrochromone (153) with some cyclic enamines namely 3-amino-2-cyclohexen-1-one, 5-amino-3-(4-pyridyl)isoxazole) and 3-amino-2-isopropyl-5-methylpyrazole afforded nitro derivatives of quinolinone 158, isoxazolo[5,4-b] pyridine 159 and pyrazolo[3,4-b]pyridine 160, respectively, via γ-pyrone ring opening followed by ring closure (Scheme 75).102
Also, 3-nitrochromone (153) as 1,3-dielectrophile reacted with some electron rich aminoheterocycles (as 1,3-dinucleophiles) giving a variety of hetero(carbo)annulated 3-nitropyridines 161-166 (Scheme 76).104 The reaction involve [3+3] cyclocondensation and proceeds in high yields and no influence greatly by the nature of the 1,3-C,N-dinucleophiles.104
Reaction of 3-nitrochromone (153) with ethyl glycinate hydrochloride in sodium ethoxide produced 1-nitropyrrolo[2,3-c]coumarin-4-one (167). Also, treating compound 153 with acetone dicarboxylic acid diethylester in pyridine gave ethyl 7-hydroxy-10-nitro-6-oxo-dibenzo[b.d]pyran-8-carboxylate (168) (Scheme 77).102
2-Methyl-3-nitrochromone (169) reacted with acid hydrazides, hydroxyl amine and ethyl aminoethanoate to give the nitro derivatives of pyrazole 170, isoxazole 171 and pyrrolo[2,3-c]coumarin 172, respectively (Scheme 78).105
Reaction of 2-methyl-3-nitrochromone (169) with guanidine, acetamidine, benzamidine and S-methylisothiourea, in sodium ethoxide, afforded the corresponding 2-substituted-6-(2-hydroxyphenyl)-4-methyl-5-nitropyrimidine 173-176 in good yields (Scheme 79).105,106
8. CONCLUSION
In conclusion, the ring opening ring closure (RORC) reactions of 3-substituted chromones with nucleophilic reagents proceed preferentially at C-2 position through 1,4-Michael addition with γ-pyrone ring opening followed by new heterocyclization reaction leading to a variety of products depending on the substrate at position 3, nature of nucleophile used and the reaction conditions.
References
1. S. K. Jash and G. Brahmachari, J. Org. Biomol. Chem., 2013, 1, 65.
2. R. S. Keri, S. Budagumpi, R. K. Pai, and R. G. Balakrishna, Eur. J. Med. Chem., 2014, 78, 340. CrossRef
3. M. Singh, M. Kaur, and O. Silakari, Eur. J. Med. Chem., 2014, 84, 206. CrossRef
4. M. Parveen, A. M. Malla, Z. Yaseen, A. Ali, and M. Alam, J. Photochem. Photobiol. B; Biology, 2014, 130, 179.
5. M. A. Ibrahim, N. M. El-Gohary, and M. A. Abd-Hamed, J. Braz. Chem. Soc., 2011, 22, 1130. CrossRef
6. R. Kaur, N. Taheam, A. K. Sharma, and R. Kharb, Res. J. Pharm., Biol. Chem. Sci., 2013, 4, 79.
7. K. S. Marles, Molecules, 2012, 17, 191.
8. J. A. Chemler, Y. Yan, E. Leonard, and M. A. Koffas, Org. Lett., 2007, 9, 1855. CrossRef
9. J. B. Harborne and C. A. Williams, Photochem., 2000, 55, 481. CrossRef
10. S. Bhatnogar, S. Sahi, P. Kackar, S. Kaushik, M. K. Dave, A. Shukla, and A. Goel, Bioorg. Med. Chem. Lett., 2010, 20, 4945. CrossRef
11. L.-C. Lim, Y.-C. Kuo, and C.-J. Chou, J. Nat. Prod., 2000, 63, 627. CrossRef
12. Y. Q. Shi, T. Fukai, H. Sakagami, W.-J. Chang, P.-Q. Yang, F.-P. Wang, and T. Nomura, J. Nat. Prod., 2001, 64, 181. CrossRef
13. J. A. Beutler, E. Hamel, A. J. Vlietinck, A. Hamers, P. Rajan, J. N. Roitman, J. H. Cardellina, and M. R. Boyd, J. Med. Chem., 1988, 41, 2333. CrossRef
14. R. Larget, B. Lockhart, P. Renard, and M. Largeron, Bioorg. Med. Chem. Lett., 2000, 10, 835. CrossRef
15. J. S. Yoon, M. K. Lee, S. H. Sung, and Y. C. Kim, J. Nat. Prod., 2006, 96, 290. CrossRef
16. A. Groweiss, J. H. Cardellins, and M. R. Boyd, J. Nat. Prod., 2000, 63, 1537. CrossRef
17. D. Yu, C. H. Chen, A. Brossi, and K. H. Lee, J. Med. Chem., 2004, 16 , 47, 4072.
18. Y. Deng, J. P. Lee, M. T. Ramamonjy, J. K. Synder, S. A. Des Etages, D. Kanada, M. P. Synder, and C. J. Turner, J. Nat. Prod., 2000, 63, 1082. CrossRef
19. I. A. Khan, M. A. Avery, C. L. Burandt, D. K. Goins, J. R. Mikell, T. E. Nash, A. Azadega, and L. A. Walker, J. Nat. Prod., 2000, 63, 1414. CrossRef
20. K. Mori, G. Audran, and H. Monti, Synlett, 1998, 259. CrossRef
21. W. G. Ma, N. Fuzzati, S. L. Lu, D. S. Gu, and K. Hostettmann, Photochem., 1996, 43, 1339. CrossRef
22. J. A. Hutter, M. Salman, W. B. Stavinoha, N. Satsangi, R. F. Williams, R. T. Streeper, and S. T. Weintraub, J. Nat. Prod., 1966, 59, 541. CrossRef
23. M. Mazzei, A. Balbi, G. Roma, M. Braccio, G. Leoncini, E. Buzzi, and M. Maresca, Eur. J. Med. Chem., 1988, 23, 237. CrossRef
24. U. M. Ceylan, E. J. Verspohi, and R. Ertan, J. Enzyme Inhib. Med. Chem., 2010, 25, 784. CrossRef
25. C. Kanadaswami, L. T. Lee, P. Lee, J. Hwang, F. C. Ke, Y. T. Huang, and M. T. Lee, In Vivo. 2005, 19, 895.
26. P. G. Pietta, J. Nat. Prod., 2000, 63, 1035. CrossRef
27. M. I. Hegab, A. S. M. Abdel-fattah, N. M. Yousef, H. F. Nour, A. M. Mostafa, and M. Elithey, Arch. Pharm. Chem. Life Sci., 2007, 340, 396. CrossRef
28. P. C. Unangst, T. Capiris, D. T. Connor, T. G. Heffner, R. G. Mackenzie, S. R. Miller, T. A. Pugsley, and L. D. Wise, J. Med. Chem., 1997, 40, 2688. CrossRef
29. G. R. Beecher, J. Nutr., 2003, 133, 3248.
30. J. R. S. Hoult, M. A. Moroney, and M. Paya, Methods Enzymol., 1994, 234, 443. CrossRef
31. N. Sepay and S. P. Dey, J. Heterocycl. Chem., 2014, doi: 10.1002/jhet.2001. CrossRef
32. M. A. Ibrahim, T. E. Ali, N. M. El-Gohary, and A. M. El-Kazak, Eur. J. Chem., 2013, 4, 311. CrossRef
33. A. S. Plaskon, O. O. Grygorenko, and S. V. Ryabukhin, Tetrahedron, 2012, 68, 2743. CrossRef
34. T. E. Ali, M. A. Ibrahim, and S. M. Abdel-Kariem, Phosphorus, Sulfur Silicon Relat. Elem., 2009, 184, 2358. CrossRef
35. R. Gasparová and M. Lácova, Molecules, 2005, 10, 937. CrossRef
36. G. Sabitha, Aldrichim. Acta, 1996, 29, 15.
37. C. K. Ghosh and S. K. Karak, J. Heterocycl. Chem., 2005, 42, 1035. CrossRef
38. A. Nohara, T. Ishiguro, K. Ukawa, H. Sugihara, Y. Maki, and Y. Sanno, J. Med. Chem.,1985, 28, 559. CrossRef
39. C. K. Ghosh and N. Tewari, J. Org. Chem., 1980, 45, 1964. CrossRef
40. C. K. Ghosh and N. Tewari, J. Org. Chem., 1980, 45, 1964. CrossRef
41. U. Petersen and H. Heitzer, Liebigs Ann. Chem., 1976, 9, 1659. CrossRef
42. V. Ya Sosnovskikh, V. S. Moshkin, and M. I. Kodess, Tetrahedron, 2008, 64, 7877. CrossRef
43. (a) V. Ya. Sosnovskikh, D. V. Sevenard, V. S. Moshkin, and O. S. Elʹtsov, Russ. Chem. Bull., 2010, 59, 2155; CrossRef (b) J. Dusemund and T. Schurreit, Arch. Pharm. (Weinheim), 1984, 317, 377. CrossRef
44. A. Nohara, K. Ukawa, and Y. Sanno, Tetrahedron, 1974, 30, 3563. CrossRef
45. F. M. Dean and R. S. Johnson, J. Chem. Soc., Perkin Trans.1, 1981, 224. CrossRef
46. V. Ya. Sosnovskikh, V. S. Moshkin, and M. I. Kodess, Tetrahedron Lett., 2009, 50, 6515. CrossRef
47. V. Ya. Sosnovskikh, V. S. Moshkin, and M. I. Kodess, Russ. Chem. Bull., 2010, 59. 615. CrossRef
48. C. K. Ghosh, C. Ghosh, and A. Patra, Indian J. Chem., 1998, 37B, 387.
49. C. K. Ghosh, N. Tewari, and C. Bandyopadhyay, Indian J. Chem., 1983, 22B, 1200.
50. V. Ya. Sosnovskikh, V. S. Moshkin, and O. S. Elʹtsov, Russ. Chem. Bull., 2010, 59. 2151. CrossRef
51. F. Risitano, G. Grassi, and F. Foti, J. Heterocycl. Chem., 2001, 38, 1083. CrossRef
52. (a) V. Ya. Sosnovskikh, D. V. Sevenard, V. S. Moshkin, V.O. Iaroshenko, and P. Langer, Tetrahedron, 2010, 66, 7322; CrossRef (b) I. Sigg, G Haas, and T. Winkler, Helv. Chim. Acta, 1982, 65, 275; CrossRef (c) G. Rihs, I. Sigg, G. Haas, and T. Winkler, Helv. Chim. Acta, 1985, 68, 1933. CrossRef
53. M. Abdel-Megid, Chem. Heterocycl. Compd., 2009, 45, 1523. CrossRef
54. M. Abdel-Megid, M. A. Ibrahim, Y. Gabr, N. M. El-Gohary, and E. A. Mohamed, J. Heterocycl. Chem., 2013, 50, 615. CrossRef
55. V. Ya Sosnovskikh and V. S. Moshkin, Chem. Heterocycl. Compd., 2012, 48, 139. CrossRef
56. M. A. Ibrahim and N. M. El-Gohary, J. Heterocycl. Chem., 2015, doi: 10.1002/jhet.2355. CrossRef
57. C. Ghosh, D. K. SinhaRoy, and K. K. Mukhopadhyay, J. Chem. Soc., Perkin Trans. 1, 1979, 1964.
58. V. Ya Sosnovskikh, V. S. Moshkin, and M. I. Kodess, J. Heterocycl. Chem., 2010, 47, 629.
59. Z. Jerzmanowska, W. Basiński, and L. Zieliniska, Pol. J. Chem., 1980, 54, 383.
60. W. Basiński and Z. Jerzmanowska, Pol. J. Chem., 1983, 57, 471.
61. V. Ya. Sosnovskikh, V. S. Moshkin, and M. I. Kodess, Tetrahedron Lett., 2008, 49, 6856. CrossRef
62. K. Kubo, K. Urania, S. Kuzuna, and A. Nohara, Chem. Pharm. Bull., 1986, 36, 1108. CrossRef
63. G. Singh, R. Singh, N. K. Girdhar, and M. P. S. Ishar, Tetrahedron, 2002, 58, 2471. CrossRef
64. M. A. Ibrahim, Synth. Commun., 2009, 39, 3527. CrossRef
65. M. A. Ibrahim, Eur. J. Chem., 2010, 1, 124. CrossRef
66. M. A. Ibrahim, N. M. El-Gohary, S. S. Ibrahim, and S. Said, Chem. Heterocycl. Compd., 2015, 50, 1624. CrossRef
67. M. A. Ibrahim, A. A. M. Farag, and N. M. El-Gohary, Synth. Met., 2015, 203, 91. CrossRef
68. M. A. Ibrahim, Tetrahedron, 2013, 69, 6861. CrossRef
69. C. K. Ghosh, Synth. Commun., 1978, 487. CrossRef
70. C. K. Ghosh and N. Tewari, Indian J. Chem., 1982, 21B, 881.
71. C. K. Ghosh, C. Bandyopadhyay, and J. Maitti, Heterocycles, 1987, 26, 1623. CrossRef
72. A. H. Abdel-Rahman, M. M. Girges, A. S. El-Ahl, and L. M. Sallem, Heteroat. Chem., 2006, 17, 2. CrossRef
73. C. K. Ghosh and C. Ghosh, J. Indian Chem. Soc., 1999, 76, 537.
74. E. Dimitriadou, M. Raftopoulou, P. M. Kasapidou, C. A. Tsoleridis, J. Stephanidou-Stephanatou, D. J. Hadjipavlou-Litina, C. Kontogiorgis, A.Pritsa, and A. Papadopoulos, ARKIVOC, 2014, iv, 372.
75. M. A. Ibrahim, H. M. Hassanin, Y. A. Gabr, and Y. A. Alnamer, J. Braz. Chem. Soc., 2012, 5, 905. CrossRef
76. M. A. Ibrahim, H. M. Hassanin, Y. A. Gabr, and Y. A. Alnamer, Eur. J. Chem., 2010, 1, 195. CrossRef
77. C. Bandyopadhyay, K. R. Sur, R. Patra, and S. Banerjee, J. Chem. Res. (s) 2003, 459; (m) 2003, 847.
78. T. Schurreit, Arch. Pharm. (Weinheim), 1987, 320, 500. CrossRef
79. M. A. Ibrahim and N.M. El-Gohary, J. Heterocycl. Chem., 2015, doi: 10.1002/jhet.2368.
80. M. A. Ibrahim, Tetrahedron, 2009, 65, 7687. CrossRef
81. M. A. Ibrahim, J. Braz. Chem. Soc., 2013, 24, 1754.
82. B. Chantegrel, A. Nadi, and S. Gelin, Tetrahedron Lett., 1983, 24, 381. CrossRef
83. C. K. Ghosh and K. K. Mukhopadhyay, Synthesis, 1978, 779. CrossRef
84. B. Chantegrel, A. Nadi, and S. Gelin, J. Org. Chem., 1984, 49, 4419. CrossRef
85. M. A. Ibrahim, ARKIVOC, 2008, xvii, 192.
86. M. A. Ibrahim and T.E. El-Sayed, Turk. J. Chem., 2015, 39, 412. CrossRef
87. S. Klutchko, J. Shavel Jr, and M. von Strandtmann, J. Org. Chem., 1974, 39, 2436. CrossRef
88. L. Bazyi, S. Kisil, S. Frolov, Y. Burgart, and V. Saloutin, Russ. Chem. Bull., 1999, 48, 1537. CrossRef
89. S. Kisil, Y. Burgart, and V. Saloutin, Russ. J. Org. Chem., 2001, 37, 1455. CrossRef
90. E. Budzisz, M. Malecka, and B. Nawrot, Tetrahedron, 2004, 60, 1749. CrossRef
91. A. Kufelnicki, M. Woźniczka, L. Chęcińska, M. Miernicka, and E. Budzisz, Polyhedron, 2007, 26, 2589. CrossRef
92. J. Nawrot-Modranka, E. Nawrot, and J. Graczyk, Eur. J. Med. Chem., 2006, 41, 1301. CrossRef
93. M. A. Terzidis, C. A. Tsoleridis, J. Stephanidou-Stephanatou, A. Terzis, C. P. Raptopoulou, and V. Psycharis, Tetrahedron, 2008, 64, 11611. CrossRef
94. R. B. Gammill, S. A. Nash, and S. A. Mizsak, Tetrahedron Lett., 1983, 24, 3435. CrossRef
95. W. Huang, M.-Z. Liu, Y. Li, Y. Tan, and G.-F. Yang, Bioorg. Med. Chem., 2007, 15, 5191.
96. R. B. Gammill, S. A. Nash, L. T. Bell, and W. Watt, Tetrahedron Lett., 1992, 33, 993. CrossRef
97. Y. Sugita, S. Yin, and I. Yokoe, Heterocycles, 2000, 53, 2191. CrossRef
98. V. Ya Sosnovskikh, B. I. Usachev, and A. Yu. Sizov, Russ. Chem. Bull., 2003, 52, 508. CrossRef
99. V. Ya Sosnovskikh, B. I. Usachev, and A. Yu. Sizov, Russ. Chem. Bull., 2003, 52, 984. CrossRef
100. S. Maiti, J. Ghosh, T. Sarkar, and C. Bandyopadhyay, J. Indian Chem. Soc., 2013, 90, 1497.
101. P. Biswas, J. Ghosh, T. Sarkar, S. Maiti, and C. Bandyopadhyaya, J. Chem. Res., 2012, 36, 623. CrossRef
102. G. Haas, J. L. Stanton, and T. Winkler, J. Heterocycl. Chem., 1981, 18, 61.
103. M. J. Nawrot and K. Kostka, Pol. J. Chem., 1989, 63, 103.
104. V. O. Iaroshenko, S. Mkrtchyan, A. Gevorgyan, M. Vilches-Herrera, D. V. Sevenard, A. Villinger, T. V. Ghochikyan, A. Saghiyan, V. Ya Sosnovskikh, and P. Langer, Tetrahedron, 2012, 68, 2532. CrossRef
105. K. Takagi, M. Tanaka, Y. Murakami, K. Ogura, K. Ishii, H. Morita, and T. Aotsuka, J. Heterocycl. Chem., 1987, 24, 1003. CrossRef
106. M. Tanaka, Y. Murakami, H. Morita, and K. Takagi, Chem. Pharm. Bull., 1985, 33, 2129 CrossRef