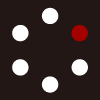
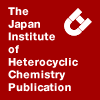
HETEROCYCLES
An International Journal for Reviews and Communications in Heterocyclic ChemistryWeb Edition ISSN: 1881-0942
Published online by The Japan Institute of Heterocyclic Chemistry
e-Journal
Full Text HTML
Received, 18th July, 2015, Accepted, 30th July, 2015, Published online, 11th August, 2015.
■ Synthesis of the Aglycon of Pentalinonside
Nobuhisa Isaka, Saki Kawada, Masaji Ishiguro,* and Minoru Tamiya*
Chemical Biology Laboratory, Department of Applied Life Sciences, Niigata University of Pharmacy and Applied Life Sciences, 265-1, Higashijima, Niigata 956-8603, Japan
Abstract
The aglycon of pentalinonside (2), a novel seco-pregnane with cage-like DEF-rings, was synthesized. Polyoxygenated DEF-rings were constructed using a known 14,20(16),21(15)-triol derivative via 5-exo-cyclization for the F-ring annulation, followed by C14–21(15) oxidative cleavage and intramolecular acetalization.A native Mexican plant called Pentalinon andrieuxii Mueller-Itrgoviensis, which grows in the Yucatan Peninsula, is a Mayan folk medicine for the treatment of cutaneous leishmaniasis lesions. Recent biological studies of extracts of this plant have revealed anti-atherogenic,1 anti-inflammatory,2 anti-leishmania,3 and anti-depressant activities.4 As part of the discovery of naturally occurring anti-leishmania agents from P. andrieuxii, pentalinonside (1, Figure 1), a novel polyoxygenated 14,15-seco-pregnane with a β-diginosyl group at C3 and cage-like DEF-rings, was isolated from the hexane-soluble extract of its roots in 2012.5 Although isolated from bioactive extracts, pentalinonside (1) does not exhibit anti-leishmania activity.
Velutinol A (3), a seco-pregnane with cage-like DEF-rings, was also isolated6 and revealed to be an antagonist of the bradykinin B1 receptor (B1R).7 As part of our efforts to elucidate the mechanism of action of B1R, a preliminary complex structural model of velutinol A (3) bound to the B1R was constructed, which indicated that three oxygen atoms in the cage-like structure of the DEF-rings of velutinol A (3) are important for binding.8 Therefore, we anticipated that seco-pregnane derivatives bearing cage-like DEF-rings might exhibit similar bioactivities. We have also accomplished the syntheses of velutinol A (3)8 and the seco-pregnane derivatives argeloside aglycon (4)9 and illustrol (5),10 which share similar cage-like DEF-rings, to study the structure-activity relationship. Due to its structural similarity to velutinol A (3), our main goal is the synthesis of the pentalinonside aglycon (2), even though the biological activities of pentalinonside (1) have not yet been specified.
Scheme 1 shows our retrosynthetic analysis of pentalinonside aglycon (2), which began with the deacetalization of the target to 14-keto-16(20),21(15)-dihydroxy derivative I. By assuming that ketone I would presumably be obtained from diol II via oxidative cleavage of the C14–C21(15) bond, diol II was established as the precursor. To construct the F-ring in II, we envisioned 5-exo-cyclization via intramolecular SN2 addition of the C15(21) hydroxy group to the C20(16) position of the known α,α-dihydroketone III.8
As shown in the retrosynthetic analysis, the synthesis begins from α,α’-dihydroxyketone 6 (Scheme 2).8 First, we chose a mesylate as the leaving group of C20(16). Thus, after sulfonylation of the C20(16)-hydroxy group using methanesulfonyl chloride (MsCl) in pyridine, the TBS groups of the resulting ketone 7 were removed via nBu4NF, which unfortunately resulted in the formation of an oxatholanedioxide ring to give 8 in 45% yield.11 The structure of 8 was established by heteronuclear multiple bond correlations (HMBC) experiments of diacetate 9, easily accessible from compound 8. Thus, after the protection of the 15(21),16(20)-dihydroxy groups as acetyl groups (86%), the HMBC correlations of the resulting compound 9 were measured (Figure 2).
HMBC correlations from H21’α to C20(16)/C21(15) and from H21’(15’)β to C14/C21(15) indicated the formation of an oxatholanedioxide ring. There was no HMBC correlation from H21’α to C14 nor from H21’β to C20(16) because of the dihedral angles (approximately 90°) of each of the protons and carbon atoms [H21’(15’)α/C14 and H21’(15’)β/C20(16)], as shown in the Figure 2, which is the characteristic conformation of bicycle[3. 3. 0]octane skeletons. The occurrence of the oxatholanedioxide ring is also supported by mass spectrometry.
To prevent annulation, we planned to use triol 108 as the starting material and attach the p-toluenesulfonyl (Ts) group to the C20(16) hydroxy group. Thus, the important point of the first step is the regioselective installation of the tosyl group because there are two secondary alcohols at C20(16) and C21(15) in triol 10. We anticipated that the small difference in steric hindrance around the C20(16) and C21(15) hydroxy groups might achieve the regioselective installation of the Ts group on the C20 hydroxy group. Thus, triol 10 was treated with TsCl in the presence of 4-dimethylaminopyridine (DMAP) in CH2Cl2, which, as expected, gave tosylate 11 for F-ring annulation as the sole product in a 93% yield. The structural confirmation of compound 11 was achieved by comparing the 1H NMR values of H20(16) in compounds 10 and 11. The 1H NMR value assigned to H20(16) was shifted from 4.63 ppm to 5.52 ppm after tosylation, confirming the installation of the Ts group at the C20(16) hydroxy group.
For the key 5-exo-cyclization to annulate the F-ring, nBu4NF was used not only to remove the TBS groups but also as a weak base for the intramolecular SN2-type reaction (Table 1, entry 1). Despite quantitative conversion of 11, the major product was the unfavorable ketone 16 (89%), generated by the E2
elimination of TsOH and tautomerization of the resulting enol, along with tetrol 15 (9%). This result suggested that basic conditions would not be adequate for the F-ring annulation of 11 because the basicity of the reaction, even with the distinctly weak base nBu4NF, triggered the E2 elimination of 11. To prevent E2 elimination, diol 11 was treated with 2.5 equivalents of nBu4NF in the presence of 1.3 equivalents of AcOH for 2 d (entry 2). Under these conditions, the desired product 12 was obtained in 35% yield concomitant with the recovery of the starting material in 15% yield. Increasing the equivalents of AcOH from 1.3 to 2.5 increased the recovery (35%, entry 3). The product was obtained in 46% yield when 5.0 equivalents of AcOH were added to the reaction (entry 4). The reaction was ultimately performed at 60 °C for 2 h in the presence of 5.0 equivalents of AcOH to afford 12 in 71% yield (entry 5). We elucidated the structure of compound 12 based on HMBC correlations between H15(21) and C20(16), which indicated the formation of the F-ring.
The C14–21(15) bond of cyclized compound 12 was then oxidatively cleaved using NaIO4 to give keto–aldehyde 13 in 81% yield (Scheme 2). Selective reduction of the C20(16)-oxo group in 13 was achieved via NaBH4 at 0 °C to afford hemi-ketal 14 [71%, confirmed by HMBC correlation between H21(15) and C14]. Treatment of 14 with HClO4 achieved the conversion of the AB rings, detachment of the TBS group, and intramolecular ketalization to give pentalinonside aglycon (2) in 95% yield. The structure of 2 was confirmed by comparing the 1H-NMR and 13C-NMR spectra12 of the synthetic aglycon and authentic pentalinonside.5 As shown in Figure 3, the 13C-NMR peaks of 2 were nearly identical to those of pentalinonside (1), with the exception of those peaks assigned as C2–C4 from the ΔδC value (authentic – synthetic/ppm), which are the positions adjacent to the glycoside bond.
In summary, we have accomplished the concise synthesis of pentalinonside aglycon (2) in 5 steps by utilizing compound 10, the intermediate in velutinol A (3) synthesis. The stereoselective glycosylation of 2,6-dideoxy saccharide for the total synthesis of pentalinonside (1) is now under investigation.
ACKNOWLEDGEMENTS
This project was partially supported by The Science Research Promotion Fund and a Grant-in-Aid for Scientific Research B. Naito Foundation Natural Science Scholarship to M. T. is gratefully acknowledged.
References
1. J. Jiu, Lloydia, 1966, 29, 250.
2. C. M. Lezama-Dávila and A. P. Isaac-Márquez, Divulge Bioméd., 1994, 2, 13.
3. C. M. Lezama-Dávila, A. P. Isaac-Márquez, P. Zamora-Crescencio, M. R. Úc-Encalada, S. Y. Justiniano-Apolinar, R. Angel-Robles, A. R. Satoskar, and L. Hernández-Rivero, Fitoterapia, 2007, 78, 255. CrossRef
4. M. J. Chan-Bacab, E. Balanza, E. Deharo, V. Muñoz, R. Durán-García, and L. M. Peña-Rodríguez, J. Ethnopharmacol., 2003, 86, 243. CrossRef
5. L. Pan, C. M. Lezama-Dávila, A. P. Isaac-Márquez, E. P. Calomeni, J. R. Fuchs, A. R. Satoskar, and A. D. Kinghorn, Phytochemistry, 2012, 82, 128. CrossRef
6. R. A. Yunes, M. G. Pizzolatti, A. E. G. Sant'Ana, G. E. Hawkes, and J. B. Calixto, Phytochem. Anal., 1993, 4, 76; CrossRef E. S. Bento, J. B. Calixto, G. E. Hawkes, M. G. Pizzolatti, A. E. G. Sant’Ana, and R. A. Yunes, J. Chem. Soc., Perkin Trans. 2, 1996, 1359. CrossRef
7. W. M. Mattos, M. M. Campos, E. S. Fernandes, G. P. Richetti, R. Niero, R. A. Yunes, and J. B. Calixto, Regul. Pept., 2006, 136, 98; CrossRef W. M. Mattos, J. Ferreira, G. P. Richetti, R. Niero, R. A. Yunes, and J. B. Calixto, Neuropeptides, 2006, 40, 125. CrossRef
8. N. Isaka, M. Tamiya, A. Hasegawa, and M. Ishiguro, Eur. J. Org. Chem., 2012, 665. CrossRef
9. M. Tamiya, N. Isaka, T. Kitazawa, and M. Ishiguro, Asian J. Org. Chem., 2014, 3, 264. CrossRef
10. M. Tamiya, N. Isaka, K. Ishizawa, M. Ikeda, and M. Ishiguro, Chem. Lett., 2014, 43, 1704. CrossRef
11. X. Creary, J. Org. Chem., 1980, 45, 2419. CrossRef
12. 1. Chemical data of 2: solid, mp 206.2–206.4 °C; [α]D20 –37.19 (c 0.32, MeOH); 1H NMR (500 MHz, pyridine-d5): δ = 5.49–5.45 (m, 1H, H-6), 4.57 (t, 1H, J = 4.6 Hz, H-16), 4.49 (ddd, 1H, J = 8.0, 5.8, 1.2 Hz, H-20), 4.23 (d, 1H, J = 10.1 Hz, H-15b), 4.06 (dd, 1H, J = 12.6, 5.8 Hz, H-21a), 4.03 (dd, 1H, J = 10.1, 4.6 Hz, H-15a), 3.89–3.81 (m, 1H, H-3), 3.80 (dd, 1H, J = 12.6, 1.2 Hz, H-21b), 2.66–2.60 (m, 1H, H-7), 2.63–2.58 (m, 1H, H-4), 2.61 (dd, 1H, J = 8.0, 4.6 Hz, H-17), 2.52–2.44 (m, 1H, H-7), 2.13–2.06 (m, 1H, H-2a), 1.90–1.83 (m, 1H, H-1b), 1.89–1.84 (m, 1H, H-8), 1.83–1.73 (m, 1H, H-2b), 1.52–1.46 (m, 1H, H-11), 1.49–1.43 (m, 1H, H-12b), 1.45–1.40 (m, 1H, H-9), 1.38–1.32 (m, 1H, H-12a), 1.34–1.28 (m, 1H, H-11), 1.18–1.10 (m, 1H, H-1a), 1.1 (s, 3H, H-18), 1.02 (s, 3H, H-19) ppm; 13C NMR (125 MHz, pyridine-d5): δ = 140.4 (C-5), 121.8 (C-6), 108.1 (C-14), 80.7 (C-16), 76.2 (C-20), 74.2 (C-15), 71.2 (C-3), 66.1 (C-21), 54.5 (C-17), 46.0 (C-9), 43.4 (C-4), 41.3 (C-10), 37.7 (C-1), 36.8 (C-13), 36.2 (C-8), 34.8 (C-12), 32.5 (C-2), 25.4 (C-79, 20.5 (C-11), 19.6 (C-19), 17.2 (C-18) ppm; IR (ATR): v = 3309, 2927, 1439, 1381, 1364, 1269, 1202, 1169, 1133, 1101, 1052, 1019, 986, 972, 954 cm–1; HRMS (EI): m/z calcd for C21H30O4 [M+H]: 302.2217, found: 302.2223.