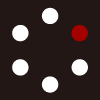
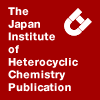
HETEROCYCLES
An International Journal for Reviews and Communications in Heterocyclic ChemistryWeb Edition ISSN: 1881-0942
Published online by The Japan Institute of Heterocyclic Chemistry
e-Journal
Full Text HTML
Received, 27th July, 2015, Accepted, 18th August, 2015, Published online, 27th August, 2015.
■ Design, Synthesis, and Evaluation of Novel 2-Halogenated or Aminated Carbocyclic Oxetanocin a Analogs as Potential Angiogenic Agents
Norikazu Sakakibara,* Junsuke Igarashi, Maki Takata, Ryoji Konishi, Natsuki Suzue, Yoshihisa Kato, Tokumi Maruyama, and Ikuko Tsukamoto
Kagawa School of Pharmaceutical Sciences, Tokushima Bunri University, 1314-1 Shido, Sanuki City, Kagawa 769-2193, Japan
Abstract
We succeeded in the synthesis of four types of carbocyclic oxetanocin A (2-Chloro-C.OXT-A; COA-Cl) analog: 2-halogenated or aminated carbocyclic oxetanocin A: COA-F, COA-Br, COA-I, and COA-NH2, which were synthesized and evaluated using human umbilical vein endothelial cells. Among these compounds, 100 M COA-Br and COA-I had greater angiogenic activity, with relative tube areas of 2.10 0.32 and 2.01 0.61 (mean SD), respectively, which were comparable to that of COA-Cl (2.30 0.59).Angiogenesis, the process in which new capillary vessels are built from preexisting ones, plays an important role in a variety of situations, including wound healing, tumor growth, and metastasis. Angiogenesis promoters are of much potential benefit for therapy of various symptoms arising due to a lack of blood flow, such as Buerger's disease or chronic arteriosclerosis obliterans in diabetic patients. However, the clinical applications of these promoters as medical agents remain minimal compared with those of angiogenesis inhibitors, which are utilized as antitumor therapeutics. The lack of angiogenesis promoters used as therapeutic agents may be due to limited knowledge about them, with the exception of growth factors derived from living systems. For example, vascular endothelial growth factor (VEGF) and fibroblast growth factor (FGF) are macromolecular glycoproteins that are chemically and biologically
unstable. Hence, they are used only in treatments administered by injection or gene transfection approaches.1 Because of this situation, we synthesized the first low-molecular-weight angiogenesis promoter, COA-Cl (1a, racemate) (Figure 1),2 whose molecular weight (MW = 284) is suitable for pharmaceutical use in percutaneous and transmucosal absorption. Therefore, it is expected to have practical applications as a therapeutic for wound healing, Buerger's disease, or peripheral arterial obliterative disease, and could be developed as an adhesive skin patch, lotion, or oral agent. In addition to its angiogenic effect, we found that COA-Cl protects against oxygen-glucose deprivation in primary cortical neurons in a manner sensitive to suramin.2c Because of its utility, COA-Cl is commercially available (chemical name: 2-Cl-C.OXT-A, Wako Co., Ltd., Japan, order number: 032-21541, quantity: 1 mg, price: 15,000 yen). As for mechanistic studies of it, it was revealed that COA-Cl is a novel and potent angiogenic agent that may behave as a partial agonist of the S1P1 receptor.2a,2d Understanding of the utility and unique mechanism of COA-Cl 1a has triggered intensive efforts to discover novel COA-Cl analogs with enhanced angiogenic activity and reduced cytotoxicity.2a,2e,2f However, our previous work resulted in analogs with almost no or less potency than COA-Cl 1a. For example, as shown in Figure 1, the COA-Cl derivative COA-OMe (1b), in which the 2-chloro group at the 2-position in the purine system is substituted with a methoxy group, displayed half the vascularization activity of COA-Cl 1a.2e Furthermore, substitution of an H in place of the Cl (1c: C.OXT-A) or replacement of a cyclobutane moiety with a pentose ring (1d: cladribine) showed no angiogenic potency.2a In a single exceptional case, COA-Cl analog 1e, regioisomer of the two hydroxymethyl groups at the cyclobutane ring of 1a, exhibited angiogenic activity comparable to that of COA-Cl 1a.2f Collectively, these studies indicated that the structure of not only the cyclobutane ring moiety but also the 2-substituted purine skeleton in COA-Cl 1a is essential for its angiogenic activity. In an ongoing effort to discover potential COA-Cl analogs with high angiogenic activity, we were interested in the structure-activity relationship around the functional group at the 2-position of the purine skeleton of COA-Cl. Therefore, here we designed four structurally relevant, novel classes of halogenated or aminated COA-Cl analogs (7, 2b, 2c, and 2ab), by replacing the 2-chloro group of the purine skeleton with appropriate groups (X = F, Br, I, NH2), as shown in Figure 2.
The synthesis of COA-Cl analogs 7, 2b, 2c, and 2ab is described in Scheme 1. The intermediate cyclobutyl alcohol 3 was prepared using commercially available bromoacetaldehyde diethyl acetal as starting material in five-step reactions, according to a modified version of a method in a previous report.3 Secondary alcohol 3 was treated with 2-halogenated 6-chloropurine (4a: 6-chloro-2-fluoropurine, 4b: 2-bromo-6-chloropurine, 4c: 6-chloro-2-iodepurine) and underwent the Mitsunobu reaction4 in the presence of PPh3 and DIAD to yield 2-halogenated 9-cyclobutyl-6-chloropurine congeners, 5ac. The yields for Mitsunobu reaction differ considerably depending on the substrate, at 2662% yields; in particular, the coupling of 2-bromo-6-chloropurine 4b and cyclobutanol 3 had a low yield of 26%, which corresponds to a previous report5 that 4b was coupled with the secondary alcohol under almost the same conditions as a Mitsunobu reaction using PPh3, DIAD, and THF. Then, 5b or 5c was treated with methanolic ammonia at 70 C to give the desired 2-brominated or 2-iodinated products of 6-aminopurine analog 2b (COA-Br) and 2c (COA-I) at 78 and 60% yields, respectively. In contrast, for 6-chloro-2-fluoropurine derivative 5a under the same reactive conditions, we observed no objective 2-fluorinated substrate, but the simultaneous production of 2-methoxylated and 2-aminated products of 6-aminopurine analog 2aa (COA-OMe) and 2ab (COA-NH2) occurred at 27 and 45% yields because the fluorine group at the 2-position of the purine skeleton of 5a was substituted under the thermal conditions by a methoxy group or amino group derived from methanol or ammonia in the reaction system, respectively. Subsequently, 2-fluoropurine analog 5a was treated under the alternative mild ammonolysis reaction with liq. NH3/MeOH at 4 C to give 2-fluoro-9-[2,3-bis(benzoyloxymethyl)cyclobutyl]purine derivative 6 at 21% yield. Then, compound 6 was deprotected with Na2CO3/CD3OD condition6 to afford the desired 6-chloro-2-fluoropurine analog 7.
The effects of the four synthesized types of 2-halogenated or aminated COA-Cl analog: COA-F (7), COA-Br (2b), COA-I (2c), and COA-NH2 (2ab), on angiogenesis were examined. We evaluated the analogs using a well-established tube formation assay.7 Typical pictures are shown in Figure 3. VEGF was used as a positive control. The area of the formed tube is presented as a value relative to that of tubes formed in wells without additives (saline). Figure 4 shows the dose-dependent tube formation responses, as well as the solvent control (10% saline) and positive control (VEGF, 10 ng/mL).
As shown in Figures 3 and 4, angiogenic potencies were observed for three prepared compounds, namely, COA-F (7) at a concentration of 100 M, COA-Br (2b) at 10 and 100 M, and COA-I (2c) at 1, 10, and 100 M (Figure 4). Among these, at a concentration of 100 M, 2b and 2c exerted greater angiogenic
activities (2.10 0.32 and 2.01 0.61 [mean SD], respectively), which were comparable to that of COA-Cl 1a (2.30 0.59). In contrast, COA-F (7) at 1 and 10 M, COA-Br (2b) at 1 M, and COA-NH2 (2ab) at all concentrations showed no effect on angiogenic potency (Figure 4). Moreover, as shown in Figure 5, we performed a proliferation assay because angiogenesis is intimately associated with complex cellular processes, including proliferation of endothelial cells,1a,8 which showed that all examined compound (7, 2b, 2c, and 2ab) did not stimulate the proliferation of HUVECs.
In conclusion, we designed four structurally relevant COA-Cl analogs: COA-F, COA-Br, COA-I, and COA-NH2 (7, 2b, 2c and 2ab), which of 2b and 2c had greater angiogenic activity at 100 M, with relative tube areas of 2.10 0.32, and 2.01 0.61, respectively. This was comparable with the tube area induced by COA-Cl (1a, 2.30 0.59). Overall, our data will be utilized in the future in preliminary structure–activity relationship studies and molecular modeling analyses using COA-Cl or its corresponding analogs as a ligand, performed to explore the major interactions between the S1P1 receptor and COA-Cl (1a), as well as COA-Cl derivatives.
EXPERIMENTAL
Instrumentation
1H NMR and 13C NMR spectra were taken with a UltrashieldTM 400 Plus FT NMR System (BRUKER). Chemical shifts and coupling constants (J) were given in and Hz, respectively. Melting points were determined on a Yanaco MP-500D. Elementary analyses were determined by a Perkin Elmer Series II CHNS/O Analyzer 2400. High-resolution mass spectrometry was performed on a APEX IV mass spectrometer (BRUKER) with electrospray ionization mass spectroscopy (ESI-MS).
General procedure for the synthesis of 5ac
A solution of compound 3 (374.4 mg, 1.10 mmol), triphenylphosphine (288.5 mg, 1.10 mmol), 4ac (1.00 mmol) and DIAD (diisopropyl azodicarboxylate, 216.6 L, 1.10 mmol) in THF (5.5 mL) was stirred at 50 C. After 217 h stirring, the residual solution was purified by silica gel column chromatography (60% AcOEt in hexane) to give 5ac.
6-Chloro-2-fluoro-9-[trans-trans-2,3-bis(benzoyloxymethyl)cyclobutyl]purine (5a)
Yield 39%; oil; 1H NMR (400 MHz, CDCl3): δ 8.17 (1 H, s, H-8), 7.98-8.12 (3 H, m, 2-OBz and 3-OBz), 7.82-7.88 (2 H, m, 2-OBz and 3-OBz), 7.53-7.63 (3 H, m, 2-OBz and 3-OBz), 7.34-7.50 (2 H, m, 2-OBz and 3-OBz), 4.82 (1 H, dd, J 17.6 and 8.4, H-1), 4.45-4.61 (4 H, m, 2-CH2 and 3-CH2), 3.45-3.53 (1 H, m, H-2), 2.71-2.81 (2 H, m, H-4), 2.58-2.66 (1 H, m, H-3); 13C NMR (100 MHz, CDCl3): 166.5, 166.3, 156.9 (d, J 219), 156.9 (d, J 19), 153.5 (d, J 17), 144.9 (d, J 3), 133.4, 133.5, 130.8 (d, J 5), 129.7, 129.5, 128.7, 128.5, 118.0 (d, J 4), 65.9, 64.5, 49.2, 45.4, 31.2, 29.3; HRMS (ESI) Calcd for C25H20ClFN4NaO4 [MNa]: 517.10493. Found: 517.10443.
2-Bromo-6-chloro-9-[trans-trans-2,3-bis(benzoyloxymethyl)cyclobutyl]purine (5b)
Yield 26%; oil; 1H NMR (400 MHz, CDCl3): δ 8.17 (1 H, s, H-8), 8.04-8.09 (2 H, m, 2-OBz and 3-OBz), 7.79-7.84 (2 H, m, 2-OBz and 3-OBz), 7.52-7.59 (2 H, m, 2-OBz and 3-OBz), 7.44-7.50 (2 H, m, 2-OBz, 3-OBz), 7.36-7.41 (2 H, m, 2-OBz, 3-OBz), 4.91 (1 H, dd, J 17.2 and 9.2, H-1), 4.49-4.62 (4 H, m, 2-CH2 and 3-CH2), 3.35-3.44 (1 H, m, H-2), 2.74-2.83 (1 H, m, H-4), 2.59-2.72 (2 H, m, H-3 and H-4); 13C NMR (100 MHz, CDCl3): 166.4, 152.9, 151.6, 144.2, 143.0, 133.4, 129.7, 129.4, 128.6, 66.1, 64.5, 49.5, 31.3, 29.5, 22.0; HRMS (ESI) Calcd for C25H20BrClN4NaO4 [MNa]: 577.02487. Found: 577.02336.
6-Chloro-2-iodo-9-[trans-trans-2,3-bis(benzoyloxymethyl)cyclobutyl]purine (5c)
Yield 62%; oil; 1H NMR (400 MHz, CDCl3): δ 8.11 (1 H, s, H-8), 8.02-8.09 (2 H, m, 2-OBz and 3-OBz), 7.79-7.84 (2 H, m, 2-OBz and 3-OBz), 7.51-7.62 (2 H, m, 2-OBz and 3-OBz), 7.43-7.50 (2 H, m, 2-OBz and 3-OBz), 7.34-7.41 (2 H, m, 2-OBz and 3-OBz), 4.90 (1 H, dd, J 17.2 and 8.8, H-1), 4.50-4.62 (4 H, m, 2-CH2 and 3-CH2), 3.22-3.41 (1 H, m, H-2), 2.74-2.82 (1 H, m, H-4), 2.59-2.70 (2 H, m, H-3, H-4); 13C NMR (100 MHz, CDCl3): 171.1, 166.4, 152.5, 150.5, 143.7, 133.4, 129.6, 128.6, 128.5, 66.2, 64.6, 49.4, 46.1, 31.2, 29.5;HRMS (ESI) Calcd for C25H20IClN4NaO4 [MNa]: 625.0112. Found: 625.01033.
General procedure for the synthesis of 2aa, 2ab, 2b and 2c
Compound 5ac (0.16 mmol) was dissolved in NH3 (14.0 mL)/MeOH (3.0 mL), and then sealed and stirred for several hours at 70 C. The mixture was evaporated, and the residue was purified by silica gel column chromatography (2030% MeOH in CH2Cl2) to afford 2aa, 2ab, 2b and 2c.
6-Amino-2-methoxy-9-[trans-trans-2,3-bis(hydroxymethyl)cyclobutyl]purine (2aa)
Yield 27%; pale yellow oil; 1H NMR (400 MHz, CD3OD); δ 7.91 (1 H, s, H-8), 4.52 (1 H, dd, J 17.2 and 8.4, H-1), 3.85 (3 H, s, 2-OCH3), 3.53-3.63 (4 H, m, 2-CH2 and 3-CH2), 2.77-2.84 (1 H, m, H-2), 2.41-2.50 (1 H, m, H-4), 2.29-2.37 (1 H, m, H-4), 2.07-2.17 (1 H, m, H-3); 13C NMR (100 MHz, CDCl3): 162.1, 156.7, 151.2, 138.6, 115.2, 64.0, 62.2, 53.7, 33.4, 28.1, 13.1; HRMS (ESI) Calcd for C12H17N5NaO3 [MNa]: 302.12236. Found: 302.12229.
2,6-Diamino-9-[trans-trans-2,3-bis(hydroxymethyl)cyclobutyl]purine (2ab)
Yield 45%; pale yellow crystals; 1H NMR (400 MHz, CD3OD); δ 7.91 (1 H, s, H-8), 4.51 (1 H, dd, J 17.2 and 8.8, H-1), 3.63-3.77 (4 H, m, 2-CH2, 3-CH2), 2.72-2.81 (1 H, m, H-3), 2.53-2.61 (1 H, m, H-4), 2.28-2.38 (1 H, m, H-4), 2.13-2.24 (1 H, m, H-2); 13C NMR (100 MHz, CDCl3): 168.2, 160.0, 156.2, 136.7, 113.1, 63.7, 62.5, 33.4, 28.0; HRMS (ESI) Calcd for C11H16N6NaO2 [MNa]: 287.12269. Found: 287.12246; mp 179.3 C.
6-Amino-2-bromo-9-[trans-trans-2,3-bis(hydroxymethyl)cyclobutyl]purine (2b)
Yield 78%; pale yellow crystals; 1H NMR (400 MHz, CD3OD); δ 8.08 (1 H, s, H-8), 4.56 (1 H, dd, J 17.2 and 8.4, H-1), 3.55-3.63 (4 H, m, 2-CH2 and 3-CH2), 2.73-2.81 (1 H, m, H-2), 2.45-2.53 (1 H, m, H-4), 2.07-2.18 (1 H, m, H-4); 13C NMR (100 MHz, MeOD): 156.4, 150.4, 144.2, 140.0, 118.2, 63.8, 62.3, 33.3, 28.4, 27.5; HRMS (ESI) Calcd for C11H14BrN5O2 [MH]: 328.04036. Found: 328.04041; mp 146.7148.0 C.
6-Amino-2-iodo-9-[trans-trans-2,3-bis(hydroxymethyl)cyclobutyl]purine (2c)
Yield 60%; pale yellow crystals; 1H NMR (400 MHz, CD3OD); δ 8.01 (1 H, s, H-8), 4.55 (1 H, dd, J 17.2 and 8.4, H-1), 3.56-3.63 (4 H, m, 2-CH2 and 3-CH2), 2.72-2.82 (1 H, m, H-2), 2.43-2.52 (1 H, m, H-4), 2.20-2.30 (1 H, m, H-4), 2.06-2.17 (1 H m, H-3); 13C NMR (100 MHz, MeOD): 155.6, 149.8, 139.6, 118.9, 63.9, 62.4, 48.5, 33.4, 28.4, 20.7; HRMS (ESI) Calcd for C11H14IN5NaO2 [MNa]: 398.00844. Found: 398.00860; mp 188.9190.6 C.
6-Amino-2-fluoro-9-[trans-trans-2,3-bis(benzoyloxymethyl)cyclobutyl]purine (6)
Compound 5a (33.0 mg, 0.067 mmol) was dissolved in NH3 (14.0 mL)/MeOH (2.0 mL), and then sealed and stirred for 10 h at 4 C. The mixture was evaporated, and the residue was purified by silica gel column chromatography (AcOEt) to give 6 as pale yellow oil (6.8 mg, 0.041 mmol, 21%). 1H NMR (400 MHz, CDCl3): δ 7.98-8.14 (2 H, m, 2-OBz and 3-OBz), 7.86-7.90 (2 H, m, 2-OBz and 3-OBz), 7.82 (1 H, s, H-8), 7.36-7.62 (6 H, m, 2-OBz and 3-OBz), 5.99 (2 H, brs, NH2), 4.75 (1 H, dd, J 18.0 and 9.2, H-1), 4.48-4.60 (4 H, m, 2-CH2 and 3-CH2), 3.42-3.50 (1 H, m, H-2), 2.67-2.77 (1 H, m, H-4), 2.52-2.62 (1 H, m, H-3); 13C NMR (100 MHz, CDCl3): 166.6, 166.4, 158.7 (d, J 207), 157.0 (d, J 20), 151.7 (d, J 20), 139.7 (d, J 3), 133.3, 129.7, 129.5, 128.6, 128.4, 118.0 (d, J 4), 65.9, 64.5, 49.2, 45.4, 31.2, 29.3; HRMS (ESI) Calcd for C25H22FN5NaO4 [MNa]: 498.15480. Found: 498.15010.
6-Amino-2-fluoro-9-[trans-trans-2,3-bis(hydroxymethyl)cyclobutyl]purine (7)
A solution of compound 6 (15.6 mg, 0.033 mmol) and Na2CO3 (35.0 mg, 0.33 mmol), in CD3OD (0.45 mL) was stirred at ambient temperature. After 6 h stirring, the mixture was evaporated in vacuo and the residue was filtered through sintered glass, concentrated, and subjected to silica gel column chromatography (20% MeOH in CH2Cl2) to give a pale yellow solid 7 (4.8 mg, 0.018 mmol, 55%). 1H NMR (400 MHz, CD3OD): δ 8.08 (1 H, s, H-8), 4.54 (1 H, dd, J 17.6 and 8.8, H-1), 3.55-3.63 (4 H, m, 2-CH2 and 3-CH2), 2.73-2.82 (1 H, m, H-2), 2.42-2.51 (2 H, m, H-4a), 2.20-2.29 (2 H, m, H-4b), 2.08-2.16 (1 H, m, H-3); 13C NMR (100 MHz, CD3OD): 160.4 (d, J 207), 159.0 (d, J 20), 152.2 (d, J 19), 141.5 (d, J 3), 118.6 (d, J 4), 65.3, 63.6, 55.1, 37.9, 34.8, 29.8; HRMS (ESI) Calcd for C11H14FN5NaO2 [MNa]: 29.010237. Found: 290.10194; mp 180.0181.5 C.
Assay 7
Human umbilical vein endothelial cells (HUVEC), normal human dermal fibroblasts (NHDF), endothelial cell growth medium (EGM-2), endothelial cell basal medium (EBM-2) and fibroblast growth medium (FGM-2) were purchased from Lonza Japan (Tokyo, Japan). Vascular endothelial growth factor-A (VEGF) was from Kurabo Co. (Osaka, Japan). Cell Counting Kit 8 was supplied by Dojindo Molecular Technologies (Kumamoto, Japan). Anti-CD31 monoclonal antibody Human, Mouse from Dako Japan (Tokyo, Japan) and anti-Mouse IgG antibody conjugated with Alexa Fluor 488, Gout, from Life Technologies (Carlsbad, CA, USA) were used for the immuno staining. HUVEC and NHDF were cultured in EGM-2 and FGM-2, respectively.
Tube formation assay 7
HUVEC and NHDF were tripsinized and suspended separately, and were then mixed at 20,000 of HUVEC and 140,000 of NHDF in 500 μL of culture medium and seeded on gelatin-coated 24-well plates. After seeding, the plates were incubated for 24 h to permit anchorage, and then, the medium was changed with the assay medium, consisting of 450 μL of EBM-2 with 2% heat-inactivated FBS and 50 μL of saline containing additives 7, 2b, 2c and 2ab. The contents of EBM-2 are almost identical to those of EGM-2, except for the fact that the former does not include FBS, growth factors, or antibiotics. After 96 h, HUVEC was stained with first antibody against CD31 and second antibody conjugated with Alexa fluor 488. The area of the formed tube was measured by the ImageJ program. Two pictures from each well were provided for the estimation. VEGF (10 ng/mL) was used as a positive control.
Proliferation assay 2a,8
HUVECs were seeded on gelatin-coated 96-well plates, typically at 3000 cells/well in 100 μL of EGM-2. After seeding, the plates were incubated for 24 h to permit anchorage, and then, the culture medium was changed with the assay medium, consisting of 90 μL of EBM-2 with 2% heat-inactivated FBS and 10 μL of saline containing additives. The proliferation assay was performed using a Cell Counting Kit-8 48 h after the addition of compounds 7, 2b, 2c and 2ab.
ACKNOWLEDGEMENT
This research was partially supported by a Grant-in-Aid for Young Scientists (B), No. 24790123, from the Japan Society for the Promotion of Science (JSPS).
References
1. (a) V. Bobek, O. Taltynov, D. Pinterova, and K. Kolostova, Vascular Pharmacol., 2006, 44, 395; CrossRef (b) D. J. Collinson and R. Donnelly, Eur. J. Vasc. Endovasc. Surg., 2004, 28, 9; CrossRef (c) G. O'Toole, D. MacKenzie, M. F. Buckley, R. Lindeman, and M. Poole, Br. J. Plast. Surg., 2001, 54, 1. CrossRef
2. (a) I. Tsukamoto, N. Sakakibara, T. Maruyama, J. Igarashi, H. Kosaka, Y. Kubota, M. Tokuda, H. Ashino, K. Hattori, S. Tanaka, M. Kawata, and R. Konishi, Biochem. Biophys. Res. Commun., 2010, 399, 699; CrossRef (b) I. Tsukamoto, R. Konishi, M. Tokuda, Y. Kubota, T. Maruyama, H. Kosaka, and J. Igarashi, PCT Int. Appl., 2010, 95; (c) N. Okabe, E. Nakamura, N. Himi, K. Narita, I. Tsukamoto, T. Maruyama, N. Sakakibara, T. Nakamura, T. Itano, and O. Miyamoto, Brain Research, 2013, 1506, 115; CrossRef (d) J. Igarashi, T. Hashimoto, Y. Kubota, K. Shoji, T. Maruyama, N. Sakakibara, Y. Takuwa, Y. Ujihara, Y. Katanosaka, S. Mohri, K. Naruse, T. Yamashita, R. Okamoto, K. Hirano, H. Kasaka, M. Takata, R. Konishi, and I. Tsukamoto, Pharmacol. Res. Perspect., 2014, 2, e00068; CrossRef (e) N. Sakakibara, I. Tsukamoto, T. Tsurura, M. Takata, R. Konishi, and T. Maruyama, Heterocycles, 2012, 85, 1105; CrossRef (f) N. Sakakibara, J. Igarashi, M. Takata, Y. Demizu, T. Misawa, M. Kurihara, R. Konishi, Y. Kato, T. Maruyama, and I. Tsukamoto, Chem. Pharm. Bull., 2015, in press.
3. (a) G. S. Bisacchi, A. Braitman, C. W. Cianci, J. M. Clark, A. K. Field, M. E. Hagen, D. R. Hockstein, M. F. Malley, T. Mitt, W. A. Slusarchyk, J. E. Sundeen, B. J. Terry, A. V. Tuomari, E. R. Weaver, M. G. Young, and R. Zahler, J. Med. Chem., 1991, 34, 1415; CrossRef (b) G. R. Geen, T. J. Grinter, P. M. Kincey, and R. L. Jarvest, Tetrahedron, 1990, 46, 6903; CrossRef (c) W. A. Slusarchyk, M. G. Young, G. S. Bisacchi, D. R. Hockstein, and R. Zahler, Tetrahedron Lett., 1989, 30, 6453. CrossRef
4. (a) N. Sakakibara, T. Hamasaki, M. Baba, Y. Demizu, M. Kurihara, K. Irie, M. Iwai, E. Asada, Y. Kato, and T. Maruyama, Bioorg. Med. Chem., 2013, 21, 5900; CrossRef (b) N. Sakakibara, M. Baba, M. Okamoto, M. Yoyama, Y. Demizu, T. Misawa, M. Kurihara, K. Irie, Y. Kato, and T. Maruyama, Antivir. Chem. Chemother., 2015, 24, 3. CrossRef
5. H. S. Kim, M. Ohno, B. Xu, H. O. Kim, Y. Choi, X. D. Ji, S. Maddileti, V. E. Marquez, T. K. Harden, and K. A. Jacobson, J. Med. Chem., 2003, 46, 4974. CrossRef
6. S. Lee, C. Uttamapinant, and G. L. Verdine, Org. Lett., 2007, 9, 5007. CrossRef
7. (a) S. Sekiya, T. Shimizu, M. Yamato, A. Kikuchi, and T. Okano, Biochem. Biophys. Res. Commun., 2006, 341, 573; CrossRef (b) N. Asakawa, T. Shimizu, Y. Tsuda, S. Sekiya, T. Sasagawa, M. Yamato, F. Fukai, and T. Okano, Biomaterials, 2010, 31, 3903; CrossRef (c) T. Sasagawa, T. Shimizu, M. Yamato, and T. Okano, Biomaterials, 2014, 35, 206. CrossRef
8. (a) K. Yonesu, Y. Kawase, T. Inoue, N. Takagi, J. Tsuchida, Y. Takuwa, S. Kumakura, and F. Nara, Biochem. Pharmacol., 2009, 77, 1011; CrossRef (b) M. L. H. Wong, A. Prawira, A. H. Kaye, and C. M. Hovens, J. Clin. Neurosci., 2009, 16, 1119 CrossRef