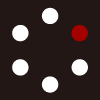
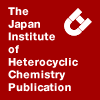
HETEROCYCLES
An International Journal for Reviews and Communications in Heterocyclic ChemistryWeb Edition ISSN: 1881-0942
Published online by The Japan Institute of Heterocyclic Chemistry
e-Journal
Full Text HTML
Received, 4th August, 2015, Accepted, 13th October, 2015, Published online, 27th October, 2015.
DOI: 10.3987/COM-15-13299
■ Microwave Assisted Synthesis of Disubstituted Imidazo[1,2-a]pyridine-3-carboxylic Acid Esters
Lin-hu Li, Zhao-yang Wu, Zhuo-rong Li, Ming-liang Liu,* Hui-yuan Guo, and Qiu-rong Zhang*
Institute of Medicinal Biotechnology, Chinese Academy of Medical Sciences and Peking Union Medical College, Beijing, 100050, China
Abstract
A novel and efficient synthetic method leveraging microwave-assisted organic synthesis (MAOS) to prepare disubstituted imidazo[1,2-a]- pyridine-3-carboxylic acid esters (IPCEs) (3a-z), the key intermediates for a class of novel anti-tuberculosis agents, is reported. Under microwave heating at 120 °C for 20 or 30 min, the condensations of 2-aminopyridines (1a-k) and ethyl 2-halogenated acetoacetates (2a-d) were conveniently performed in ethanol with acceptable yields.INTRODUCTION
Tuberculosis (TB) is a common, communicable and even fatal disease caused by Mycobacteria, mainly by Mycobacterium tuberculosis (MTB).1 The emergence of multi-drug-resistant MTB (MDR-MTB) and extensively-drug-resistant MTB (XDR-MTB) as well as the lethal combination represented by HIV co-infection indicate an urgent need for the development of new TB therapies.2 Encouragingly, a substantial progress has been made in the past decade. Especially, for the first time since 1970s, an ATP synthase inhibitor bedaquiline (TMC207)3 was approved by the US FDA for the treatment of MDR-TB in 2012. However, the clinical use of bedaquiline may be limited due to its serious adverse effects such as cardiac arrhythmias.4 Moreover, several other anti-TB candidates with different mechanisms of action are currently in clinical trials.5 Despite all this, there is still a sense of urgency to develop new and more effective drug candidates for TB treatment.
As a novel class of potent anti-TB agents targeting the QcrB subunit of the menaquinol cytochrome c oxidoreductase (bc1 complex) which is a critical component of the mycobacterial energy metabolism,6 imidazo[1,2-a]pyridine amides (IPAs) have attracted broad attentions in recent years. A number of IPA derivatives have been reported, and several of them were found to be highly active against MTB-resistant and -sensitive strains.7-13 ND-09759 and Q203 (Figure 1), two promising clinical drug candidates, were reported to possess an excellent activity against MTB including MDR-MTB and XDR-MTB as well as remarkable pharmacokinetic properties.9,10 Inspired by those research results, many series of novel IPA derivatives containing two substituent groups at the 2- and 5-, 6-, 7 or 8-positions of the IPA core have been designed and synthesized in our lab, and some of them were discovered to have nanomolar potency against MTB H37Rv and MDR-MTB (not published).
IPAs are conveniently obtained by the amidation of imidazo[1,2-a]pyridine-3-carboxylic acids (IPCAs) with amines. As one of the precursors for the manufacture of IPAs, IPCAs can be prepared by the condensation of ethyl 2-halogenated-3-oxobutanoates with 2-aminopyridines and then hydrolysis of the resulting imidazo[1,2-a]pyridine-3-carboxylic acid esters (IPCEs). Of the two steps, the hydrolytic reaction is easily accomplished in the alkali aqueous solution. Herein, we report the development of a novel synthetic method that can be used to rapidly synthesize IPCEs by microwave (MW) assisted organic synthesis (MAOS).
RESULTS AND DISCUSSION
It was reported that 2,6- and 2,7-disubstituted IPCEs were successfully prepared by the condensation of ethyl 2-chloro/bromo-3-oxobutanoate and 2-aminopyridines in ethanol at reflux overnight9 or in 1,2-dimethoxyethane at reflux for 48 h10 with good yields (50-70%). However, the yields of these compounds were found not to be satisfactory (<50%) in our repeated experiments. Moreover, the preparation of 2, 5- and 2,8-disubstituted IPCEs under the same reaction conditions met with no success, even though various reaction temperatures and durations were investigated. The condensation reaction turned out to be rather complex, and we were not able to obtain the desired products in acceptable yields.
Considering that MAOS has advantages over traditional heating methods in some cases, the synthetic methods of 2,6-dimethyl IPCE (3a) from 2-amino-5-methylpyridine (1a, 10 mmol) and ethyl 2-chloroacetoacetate (2a, 10 mmol) by MAOS under different conditions [solvents (ethanol, acetonitrile, DMF, 20 mL), temperatures (100 °C, 120 °C, 150 °C), durations (10 min, 20 min, 40 min)] were firstly investigated, and the results are shown in Table 1.
First, the condensation of 1a and 2a was performed in ethanol at 100 °C in a sealed vessel under MAOS conditions. The yield of product 3a was found to increase from 53% to 61% as the reaction time prolonged from 10 min to 20 min (Table 1, entry 1 vs 2), but a further prolonging time to 40 min failed to improve the yield (Table 1, entry 2 vs 3). Next, the reaction temperature was optimized (Table 1, entry 2 vs 4, 5). The yield (75%) significantly improved with the increase of temperature from 100 °C to 120 °C. However, a slight decrease of the yield (70%) was observed when the reaction was carried out at 150 °C. Because of this, there seemed no need to further investigate the experiment under higher temperatures (>150 °C) for safety considerations. Unfortunately, the desired product 3a was obtained only in poor yields (32% and 10%, respectively) when ethanol was replaced by acetonitrile or N,N-dimethyl- formamide (DMF) (Table 1, entry 4 vs 6, 7).
Herein, the preparation of 3a by MAOS was also compared to traditional heating methods. The reaction of 1a and 2a was performed in ethanol at reflux overnight by following well-established procedures or MW heating at 120 °C for 20 min. In the latter case, after the reaction mixture was poured into the cold water, 3a precipitated out in a high purity with a good yield (75%) comparing to the purification with chromatography in the former one (45%). Our results indicate that MAOS has evident advantages over traditional methods.
Under the above optimized condition, the substrate scope of this reaction was examined using readily available starting materials 2-aminopyridines (1a-k) and ethyl 2-chloroacetoacetate (2a) as well as commercially unavailable ethyl 2-bromoacetoacetate (2b-d), which were prepared by the bromination of corresponding ethyl acetoacetates with N-bromosuccinimide (NBS) in the presence of ammonium acetate in diethyl ether. At the beginning, we made a search for the 2-aminopyridine substrate scope using 2a as a model substrate (Table 2, entries 1-11). It was found that the position of the substituent of 1a-k showed significant impact on the yield: the best one was 5-, next 4-, then 3- or 6-position, which was consistent with the distance from the reaction region of the substrates (Table 2, entry 1 vs 6 vs 8, 4 vs 7 vs 9 vs 11). On the other hand, an electron-donating group at 5- or 4-position favored this reaction more than an electron-withdrawing one (Table 2, entry 1 vs 2-5 vs 10, 6 vs 7). Subsequently, halogenated aliphatic ethyl esters substrates were investigated using 1a as a model substrate (Table 2, entries 1, 12-14). The results suggested that the yield seemed to decrease with the increase of the size of the alkyl group R2 (Table 2, entry 1 vs 12 vs 13 vs 14) mainly due to the steric hindrance of the reaction. Finally, other necessary IPCEs (Table 2, entries 15-26) for our IPA derivatives were also prepared by MAOS in good yields (59-73%) except the entries 18 and 19 (36% and 46%, respectively). The correlations between the substrates and yields were considered to be similar to that of the mentioned above. The data shown in Table 2 exhibited the generality of MAOS for synthesis of IPCEs with respect to a range of 2-aminopyridine and ethyl 2-halogenated acetoacetate substrates.
In general, the reaction occurred at a very fast speed relative to the traditional heating methods. In fact, it only took 20 min and 30 min for 4- / 5-substituted 2-aminopyridines and 3- / 6-substituted 2-aminopyridines, respectively. In some cases, high-purity products could precipitate out after the reaction mixture was poured into the cold water. But in other cases, products must be purified by chromatography. The structural elucidation of the products 3a-z was determined from their 1H NMR, 13C NMR, and MS spectra.
Finally, it’s important to note that a resemble method for the synthesis of IPCEs by MAOS has been reported14: the advantages of this procedure are its thermal condition (55 °C, within 30 min, and without solvent), microwave irradiation (100 W, with Al2O3, and without solvent) and high yields (58-95%), but complex post treatment process seems to be inevitable. In comparison, many of the IPCEs in our work can precipitate out after the treatment of the reaction mixture with water, although others must be purified by chromatography. What is more, all of our IPCEs were designed specifically for the further synthesis of IPAs with potent activity against MDR-MTB. In short, the method developed in our lab is to be a good alternative option for the preparation of IPCEs.
CONCLUSION
In summary, we have developed an efficient method to prepare various IPCEs including twelve new ones which were not reported before (Table 2), key intermediates for IPA derivatives, from 2-aminopyridines and ethyl 2-halogenated acetoacetates by MAOS, although some of them cannot be obtained by traditional heating methods. Undoubtedly, this MW assisted reaction lays the foundation for synthesizing 2,5-, 2,6-, 2,7- and 2,8-disubstituted IPAs. In fact, many series of IPAs had been obtained in our lab and some of them were found to have an excellent activity against MTB H37Rv and MDR-MTB. We believe this strategy may be of value to those groups aiming to seek novel IPAs with a potent activity against MDR-MTB and remarkable pharmacokinetic properties.
EXPERIMENTAL
Unless otherwise noted, all reagents and solvents were purchased from commercial suppliers without further purification. All reactions were carried out with a focused microwave unit (CEM Discover SP). Each reaction was run in a 35-mL glass pressure vial. The classical heating was performed in glassware heated in an oil bath. Melting points were determined on an x-4 digital display microscope apparatus. 1H NMR (500 MHz or 400 MHz) and 13C NMR (126 MHz) spectra were recorded on Mercury-400 spectrometer or INOVA-500 spectrometer using TMS as an internal standard. NMR signals are described as follows: s (singlet), d (doublet), t (triplet), q (quartet), m (multiplet). Reactions were monitored by thin layer chromatography and was carried out on Merck silica gel 60F plates. ESI-MS spectra were measured on Advion expression CMSTM instruments.
Typical procedure for the synthesis of 3a: A 35-mL microwave process vial was charged with 1a (1.08 g, 10 mmol), 2a (1.64 g, 10 mmol) and EtOH (20 mL). The reaction mixture was stirred for 20 min at 120 °C. After cooling to room temperature, the mixture was poured into cold water (100 mL) and stirred for another 1 h. The precipitate was filtered out and dried in vacuum to give the title compound 3a (1.64 g, 75%). For 7-11, 13, 18, 19, 22 and 24, after the reaction completed, the mixture was concentrated to dryness under reduced pressure and the residue was purified by silica gel column chromatography (eluted with petroleum ether : EtOAc = 4:1) to get the corresponding title compounds.
Ethyl 2,6-dimethylimidazo[1,2-a]pyridine-3-carboxylate (3a): White solid, mp 73-75 °C. 1H NMR (400 MHz, CDCl3) δ 9.15 (d, J = 7.1 Hz, 1H), 7.36 (s, 1H), 6.79 (dd, J = 7.1, 1.6 Hz, 1H), 4.41 (q, J = 7.1 Hz, 2H), 2.69 (s, 3H), 2.43 (s, 3H), 1.42 (t, J = 7.1 Hz, 3H). 13C NMR (126 MHz, CDCl3) δ 161.28, 158.98, 146.96, 134.22, 128.35, 115.97, 115.12, 112.17, 60.60, 23.65, 14.50, 13.59. MS-ESI (m/z): 219.1 (M + H)+.
Ethyl 2-methyl-6-nitroimidazo[1,2-a]pyridine-3-carboxylate (3b): Light yellow solid, mp 99-101 °C. 1H NMR (500 MHz, CDCl3) δ 10.38 (d, J = 1.9 Hz, 1H), 8.14 (dd, J = 9.8, 2.1 Hz, 1H), 7.66 (d, J = 9.8 Hz, 1H), 4.48 (q, J = 7.1 Hz, 2H), 2.77 (s, 1H), 1.46 (t, J = 7.1 Hz, 3H). 13C NMR (126 MHz, CDCl3) δ 160.89, 155.94, 146.88, 138.04, 127.87, 121.74, 116.35, 107.20, 61.33, 16.85, 14.51. MS-ESI (m/z): 219.1 (M + H)+.
Ethyl 6-fluoro-2-methylimidazo[1,2-a]pyridine-3-carboxylate (3c): Light yellow, mp 94-96 °C. 1H NMR (500 MHz, CDCl3) δ 9.28 (dd, J = 4.7, 2.4 Hz, 1H), 7.56 (dd, J = 9.7, 5.2 Hz, 1H), 7.28 (td, J = 9.8, 7.6, 2.4 Hz, 1H), 4.42 (q, J = 7.1 Hz, 2H), 2.69 (s, 3H), 1.42 (t, J = 7.1 Hz, 3H). 13C NMR (126 MHz, CDCl3) δ 161.35, 154.77, 153.43, 152.89, 144.36, 119.13, 116.89, 115.42, 113.88, 60.66, 16.79, 14.55. MS-ESI (m/z): 223.3 (M + H)+.
Ethyl 6-chloro-2-methylimidazo[1,2-a]pyridine-3-carboxylate (3d): Light yellow, mp 86-88 °C. 1H NMR (500 MHz, CDCl3) δ 9.38 (dd, J = 2.0, 0.6 Hz, 1H), 7.58 – 7.49 (m, 1H), 7.34 (dd, J = 9.4, 2.1 Hz, 1H), 4.43 (q, J = 7.1 Hz, 2H), 2.69 (s, 3H), 1.43 (t, J = 7.1 Hz, 3H). 13C NMR (126 MHz, CDCl3) δ 161.34, 153.33, 145.24, 137.20, 128.90, 126.13, 122.06, 116.99, 60.73, 16.79, 14.57. MS-ESI (m/z): 239.7 (M + H)+.
Ethyl 6-bromo-2-methylimidazo[1,2-a]pyridine-3-carboxylate (3e): Light yellow, mp 90-91 °C. 1H NMR (500 MHz, CDCl3) δ 9.37 (s, 1H), 7.56 (d, J = 9.4 Hz, 1H), 7.34 (d, J = 9.0 Hz, 1H), 3.97 (s, 3H), 3.09 (dd, J = 14.9, 7.4 Hz, 2H), 1.34 (t, J = 7.5 Hz, 3H). 13C NMR (126 MHz, CDCl3) δ 161.48, 158.66, 145.32, 128.74, 126.03, 121.94, 117.04, 112.20, 51.52, 23.40, 13.37. MS-ESI (m/z): 283.3, 285.2 (M + H)+.
Ethyl 2,7-dimethylimidazo[1,2-a]pyridine-3-carboxylate (3f): White solid, mp 76-78 °C. 1H NMR (500 MHz, CDCl3) δ 9.38 (dd, J = 2.0, 0.6 Hz, 1H), 7.58 – 7.49 (m, 1H), 7.34 (dd, J = 9.4, 2.1 Hz, 1H), 4.43 (q, J = 7.1 Hz, 2H), 2.69 (s, 3H), 1.43 (t, J = 7.1 Hz, 3H). 13C NMR (126 MHz, CDCl3) δ 161.59 (s), 152.89, 147.39, 139.05, 127.20, 116.16, 115.43, 112.22, 77.41, 77.16, 76.91, 60.24, 21.47, 16.76, 14.60. MS-ESI (m/z): 219.3 (M + H)+.
Ethyl 7-chloro-2-methylimidazo[1,2-a]pyridine-3-carboxylate (3g): Light yellow, mp 80-83 °C. 1H NMR (500 MHz, CDCl3) δ 9.23 (d, J = 7.4 Hz, 1H), 7.59 (d, J = 1.8 Hz, 1H), 6.95 (dd, J = 7.4, 2.1 Hz, 1H), 4.42 (q, J = 7.1 Hz, 2H), 2.69 (s, 3H), 1.43 (t, J = 7.1 Hz, 3H). 13C NMR (126 MHz, CDCl3) δ 161.40, 153.67, 146.89, 134.36, 128.31, 115.84, 115.17, 112.95, 77.41, 77.16, 76.91, 60.67, 16.79, 14.59. MS-ESI (m/z): 239.8 (M + H)+.
Ethyl 2,8-dimethylimidazo[1,2-a]pyridine-3-carboxylate (3h): Light yellow, mp 100-102 °C. 1H NMR (500 MHz, CDCl3) δ 9.16 (d, J = 6.9 Hz, 1H), 7.16 (m, 1H), 6.87 (t, J = 7.0 Hz, 1H), 4.41 (q, J = 7.1 Hz, 2H), 2.73 (s, 3H), 2.62 (s, 3H), 1.42 (t, J = 7.1 Hz, 3H). 13C NMR (126 MHz, CDCl3) δ 161.71, 152.14, 147.07, 126.87, 126.50, 125.89, 113.76, 113.05, 60.37, 17.16, 16.83, 14.60. MS-ESI (m/z): 219.3 (M + H)+.
Ethyl 8-chloro-2-methylimidazo[1,2-a]pyridine-3-carboxylate (3i): Light yellow, mp 79-82 °C. 1H NMR (500 MHz, CDCl3) δ 9.24 (d, J = 6.9 Hz, 1H), 7.42 (m, 1H), 6.88 (d, J = 7.2 Hz, 1H), 4.41 (q, J = 7.1 Hz, 2H), 2.74 (s, 1H), 1.42 (t, J = 7.1 Hz, 3H). 13C NMR (126 MHz, CDCl3) δ 161.36, 153.12, 144.19, 126.71, 126.61, 122.50, 114.28, 113.36, 60.74, 16.86, 14.53. MS-ESI (m/z): 239.8 (M + H)+.
Ethyl 6-methoxy-2-methylimidazo[1,2-a]pyridine-3-carboxylate (3j): White solid, mp 60-63 °C. 1H NMR (500 MHz, CDCl3) δ 9.09 (d, J = 7.6 Hz, 1H), 6.87 (d, J = 2.5 Hz, 1H), 6.63 (dd, J = 7.6, 2.6 Hz, 1H), 4.39 (q, J = 7.1 Hz, 2H), 3.87 (s, 3H), 2.65 (s, 3H), 1.41 (t, J = 7.1 Hz, 3H). 13C NMR (126 MHz, CDCl3) δ161.49, 159.79, 153.10, 148.85, 128.45, 111.74, 107.59, 94.78, 77.30, 77.05, 76.80, 60.10, 55.61, 16.60, 14.53. MS-ESI (m/z): 235.2 (M + H)+.
Ethyl 5-chloro-2-methylimidazo[1,2-a]pyridine-3-carboxylate (3k): Light yellow, mp 70-72 °C. 1H NMR (500 MHz, CDCl3) δ 9.15 (d, J = 6.9 Hz, 1H), 7.15 (d, J = 6.9 Hz, 1H), 6.86 (m, 1H), 4.40 (q, J = 7.1 Hz, 2H), 2.72 (s, 3H), 1.42 (t, J = 7.1 Hz, 3H). 13C NMR (126 MHz, CDCl3) δ 161.71, 152.22, 147.15, 126.74, 126.51, 125.87, 113.67, 113.03, 60.33, 17.15, 14.60. MS-ESI (m/z): 239.8 (M + H)+.
Ethyl 2-ethyl-6-methylimidazo[1,2-a]pyridine-3-carboxylate (3l): White solid, mp 67-68 °C. 1H NMR (500 MHz, CDCl3) δ 9.13 (s, 1H), 7.52 (d, J = 9.0 Hz, 1H), 7.20 (d, J = 9.0 Hz, 1H), 4.40 (q, J = 7.1 Hz, 2H), 3.08 (q, J = 7.4 Hz, 2H), 2.36 (s, 3H), 1.42 (t, J = 7.1 Hz, 3H), 1.33 (t, J = 7.5 Hz, 3H). 13C NMR (126 MHz, CDCl3) δ 161.66, 158.02, 146.12, 130.49, 126.14, 123.54, 116.18, 111.66, 60.27, 23.76, 18.54, 14.55, 13.70. MS-ESI (m/z): 233.3 (M + H)+.
Ethyl 6-methyl-2-propylimidazo[1,2-a]pyridine-3-carboxylate (3m): White solid, mp 53-55 °C. 1H NMR (500 MHz, CDCl3) δ 9.13 (s, 1H), 7.51 (d, J = 9.0 Hz, 1H), 7.20 (d, J = 9.0 Hz, 1H), 4.40 (q, J = 7.1 Hz, 2H), 3.03 (t, J = 7.7 Hz, 2H), 1.86 – 1.70 (m, 2H), 1.41 (t, J = 7.1 Hz, 3H), 0.99 (t, J = 7.4 Hz, 3H). 13C NMR (126 MHz, CDCl3) δ 161.67, 156.75, 146.06, 130.50, 126.13, 123.50, 116.14, 112.01, 60.24, 32.37, 23.05, 18.51, 14.49, 14.29. MS-ESI (m/z): 247.2 (M + H)+.
Ethyl 2-cyclopropyl-6-methylimidazo[1,2-a]pyridine-3-carboxylate (3n): Light yellow, mp 95-98 °C. 1H NMR (500 MHz, CDCl3) δ 9.10 (s, 1H), 7.43 (d, J = 9.0 Hz, 1H), 7.17 (dd, J = 9.0, 1.3 Hz, 1H), 4.43 (q, J = 7.1 Hz, 2H), 2.85 – 2.65 (m, 1H), 2.34 (s, 3H), 1.43 (t, J = 7.1 Hz, 3H), 1.21 – 1.11 (m, 2H), 1.09 – 0.97 (m, 2H). 13C NMR (126 MHz, CDCl3) δ 162.00, 157.93, 146.31, 130.60, 126.08, 123.08, 115.84, 112.48, 60.28, 18.49, 14.63, 10.00, 9.91. MS-ESI (m/z): 245.3 (M + H)+.
Ethyl 2-ethyl-6-fluoroimidazo[1,2-a]pyridine-3-carboxylate (3o): Light yellow, mp 90-93 °C. 1H NMR (500 MHz, CDCl3) δ 9.31 (dd, J = 4.8, 2.4 Hz, 1H), 7.61 (dd, J = 9.7, 5.2 Hz, 1H), 7.29 (td, J = 9.9, 7.6, 2.5 Hz, 1H), 4.43 (q, J = 7.1 Hz, 2H), 3.10 (q, J = 7.6 Hz, 2H), 1.43 (t, J = 7.1 Hz, 3H), 1.34 (t, J = 7.6 Hz, 3H). 13C NMR (126 MHz, CDCl3) δ 161.28, 158.67, 154.83, 152.94, 144.40, 119.19, 117.05, 115.52, 113.19, 60.69, 23.66, 14.52, 13.59. MS-ESI (m/z): 237.1 (M + H)+.
Ethyl 6-bromo-2-ethylimidazo[1,2-a]pyridine-3-carboxylate (3p): Light yellow, mp 107-109 °C. 1H NMR (500 MHz, CDCl3) δ 9.50 (d, J = 1.1 Hz, 1H), 7.53 (d, J = 9.4 Hz, 1H), 7.43 (dd, J = 9.4, 1.8 Hz, 1H), 4.43 (q, J = 7.1 Hz, 2H), 3.09 (q, J = 7.6 Hz, 2H), 1.43 (t, J = 7.1 Hz, 3H), 1.34 (t, J = 7.6 Hz, 3H). 13C NMR (126 MHz, CDCl3) δ 161.26, 158.39, 145.40, 131.04, 128.34, 117.43, 112.34, 108.64, 60.73, 23.61, 14.51, 13.54. MS-ESI (m/z): 299.2, 297.2(M + H)+.
Ethyl 7-chloro-2-ethylimidazo[1,2-a]pyridine-3-carboxylate (3q): Light yellow, mp 80-83 °C. 1H NMR (500 MHz, CDCl3) δ 9.23 (d, J = 7.3 Hz, 1H), 7.60 (s, 1H), 6.93 (d, J = 7.2 Hz, 1H), 4.41 (q, J = 7.1 Hz, 2H), 3.08 (q, J = 7.5 Hz, 2H), 1.41 (t, J = 7.1 Hz, 3H), 1.32 (t, J = 7.6 Hz, 3H).13C NMR (126 MHz, CDCl3) δ 161.27, 158.97, 146.96, 134.22, 128.34, 115.98, 115.11, 112.17, 60.60, 23.65, 14.49, 13.00. MS-ESI (m/z): 253.1 (M + H)+.
Ethyl 8-chloro-2-ethylimidazo[1,2-a]pyridine-3-carboxylate (3r): Light yellow, mp 94-97 °C. 1H NMR (500 MHz, CDCl3) δ 9.29 (d, J = 6.8 Hz, 1H), 7.44 (d, J = 7.4 Hz, 1H), 6.91 (t, J = 7.2 Hz, 1H), 4.44 (q, J = 7.1 Hz, 2H), 3.15 (q, J = 7.5 Hz, 2H), 1.44 (t, J = 7.1 Hz, 3H), 1.35 (t, J = 7.5 Hz, 3H). 13C NMR (126 MHz, CDCl3) δ 161.35, 158.71, 144.38, 126.84, 126.57, 122.76, 113.56, 113.36, 60.74, 23.92, 14.49, 14.22. MS-ESI (m/z): 253.1 (M + H)+.
Ethyl 2-ethyl-8-methylimidazo[1,2-a]pyridine-3-carboxylate (3s): Light yellow, mp 71-73 °C. 1H NMR (500 MHz, CDCl3) δ 9.19 (d, J = 6.9 Hz, 1H), 7.16 (d, J = 7.0 Hz, 1H), 6.87 (t, J = 7.0 Hz, 1H), 4.43 (q, J = 7.1 Hz, 2H), 3.13 (q, J = 7.5 Hz, 2H), 2.63 (s, 3H), 1.43 (t, J = 7.1 Hz, 3H), 1.34 (t, J = 7.5 Hz, 3H). 13C NMR (126 MHz, CDCl3) δ 161.53, 157.70, 150.03, 147.13, 126.63, 125.87, 113.62, 112.16, 60.22, 23.81, 17.15, 14.43, 14.27. MS-ESI (m/z): 233.2 (M + H)+.
Ethyl 6-chloro-2-propylimidazo[1,2-a]pyridine-3-carboxylate (3u): Light yellow, mp 88-90 °C. 1H NMR (500 MHz, CDCl3) δ 9.42 (s, 1H), 7.64 (d, J = 9.4 Hz, 1H), 7.38 (dd, J = 9.4, 1.4 Hz, 1H), 4.43 (q, J = 7.1 Hz, 2H), 3.08 – 3.04 (m, 2H), 1.84 – 1.74 (m, 2H), 1.43 (t, J = 7.1 Hz, 3H), 1.00 (t, J = 7.4 Hz, 4H). 13C NMR (126 MHz, CDCl3) δ 161.12, 156.51, 144.70, 129.44, 126.27, 122.51, 116.84, 112.85, 60.87, 31.88, 22.91, 14.44, 14.21. MS-ESI (m/z): 267.3 (M + H)+.
Ethyl 6-fluoro-2-propylimidazo[1,2-a]pyridine-3-carboxylate (3v): Light yellow, mp 72-75 °C. 1H NMR (500 MHz, CDCl3) δ 9.30 (dd, J = 3.2, 1.5 Hz, 1H), 7.58 (dd, J = 9.7, 5.2 Hz, 1H), 7.28 (ddd, J = 9.8, 7.7, 2.3 Hz, 1H), 4.42 (q, J = 7.1 Hz, 2H), 3.11 – 2.97 (m, 2H), 1.89 – 1.66 (m, 2H), 1.43 (t, J = 7.1 Hz, 3H), 1.00 (t, J = 7.4 Hz, 3H). 13C NMR (126 MHz, CDCl3) δ 161.34, 157.66, 154.77, 152.88, 144.52, 119.07, 117.08, 115.50, 113.55, 60.61, 32.34, 22.97, 14.49, 14.26. MS-ESI (m/z): 251.1 (M + H)+.
Ethyl 6-chloro-2-cyclopropylimidazo[1,2-a]pyridine-3-carboxylate (3w): Light yellow, mp 132-135 °C. 1H NMR (500 MHz, CDCl3) δ 9.38 (d, J = 1.9Hz, 1H), 7.49 (d, J = 9.4 Hz, 1H), 7.32 (dd, J = 9.4, 2.1 Hz, 1H), 2.80 (m, 1H), 1.22 – 1.17 (m, 2H), 1.12 – 1.07 (m, 2H). 13C NMR (126 MHz, CDCl3) δ 162.04, 158.97, 145.71, 129.10, 126.13, 121.72, 116.82, 113.08, 60.74, 51.67, 14.62, 10.40, 9.99. MS-ESI (m/z): 265.0 (M + H)+.
Ethyl 2-cyclopropyl-6-fluoroimidazo[1,2-a]pyridine-3-carboxylate (3x): White solid, mp 120-122 °C. 1H NMR (500 MHz, CDCl3) δ 9.27 (d, J = 2.1 Hz, 1H), 7.50 (d, J = 9.4, 1H), 7.24 (dd, J = 9.9, 2.6 Hz, 1H), 4.54 – 4.34 (m, 2H), 2.76-2.80 (m, 1H), 1.41-1.45 (m, 3H), 1.16 – 1.17 (m, 2H), 1.08 – 1.04 (m, 2H). 13C NMR (126 MHz, CDCl3) δ 161.62, 158.80, 154.57, 152.69, 144.62, 119.17, 116.61, 115.43, 113.88, 60.65, 49.65, 14.58, 10.19, 9.99. MS-ESI (m/z): 249.1 (M + H)+.
Ethyl 7-chloro-2-cyclopropylimidazo[1,2-a]pyridine-3-carboxylate (3y): Light yellow, mp 112-115 °C. 1H NMR (500 MHz, CDCl3) δ 9.25 (d, J = 7.4 Hz, 1H), 7.57 (d, J = 1.9 Hz, 1H), 6.93 (dd, J = 7.4, 2.1 Hz, 1H), 4.47 (q, J = 7.1 Hz, 2H), 2.82 (tt, J = 8.3, 4.9 Hz, 1H), 1.46 (t, J = 7.1 Hz, 3H), 1.24 – 1.20 (m, 2H), 1.14 – 1.08 (m, 2H). 13C NMR (126 MHz, CDCl3) δ 161.68, 158.98, 147.13, 134.52, 128.28, 115.62, 114.80, 112.92, 60.66, 14.62, 10.32, 10.02. MS-ESI (m/z): 265.3 (M + H)+.
Ethyl 2-cyclopropyl-7-methylimidazo[1,2-a]pyridine-3-carboxylate (3z): Light yellow, mp 110-112 °C. 1H NMR (500 MHz, CDCl3) δ 9.12 (d, J = 7.0 Hz, 1H), 7.31 (s, 1H), 6.73 (d, J = 6.9 Hz, 1H), 4.42 (q, J= 7.1 Hz, 2H), 2.89 – 2.67 (m, 1H), 1.42 (t, J = 7.1 Hz, 3H), 1.21 – 1.15 (m, 2H), 1.05 (m, 2H).13C NMR (126 MHz, CDCl3) δ 161.84, 158.06, 147.55, 139.25, 127.20, 115.85, 115.26, 112.30, 60.26, 21.46, 14.63, 10.00, 9.94. MS-ESI (m/z): 245.1 (M + H)+.
ACKNOWLEDGEMENTS
We are grateful for financial support from the National Science Foundation of China (81373267-003) and the National S&T Major Special Project on Major New Drug Innovations (2012ZX09301002-001- 017/023, 2014ZX09507009-003).
References
1. L. S. Feng, M. L. Liu, S. Zhang, Y. Chai, B. Wang, Y. B. Zhang, K. Lv, Y. Guan, H. Y. Guo, and C. L. Xiao, Eur. J. Med. Chem., 2011, 46, 341. CrossRef
2. P. Glaziou, D. Falzon, K. Floyd, M. Raviglione, and M. Raviglione, Semin. Respir. Crit. Care Med., 2013, 34, 3. CrossRef
3. K. Andries, P. Verhasselt, J. Guillemont, H. W. Gohlmann, J. M. Neefs, H. Winkler, J. Van Gestel, P. Timmerman, M. Zhu, E. Lee, P. Williams, D. de Chaffoy, E. Huitric, S. Hoffner, E. Cambau, C. Truffot-Pernot, N. Lounis, and V. Jarlier, Science, 2005, 307, 223. CrossRef
4. J. Cohen, Science, 2013, 339, 130. CrossRef
5. Y. S. Kwon, B. H. Jeong, and W. J. Koh, Curr. Opin. Pulm. Med., 2014, 20, 280. CrossRef
6. D. Bald and A. Koul, FEMS Microbiol. Lett., 2010, 308, 1. CrossRef
7. G. C. Moraski, L. D. Markley, M. Chang, S. Cho, S. G. Franzblau, C. H. Hwang, H. Boshoff, and M. J. Miller, Bioorg. Med. Chem., 2012, 20, 2214. CrossRef
8. G. C. Moraski, L. D. Markley, J. Cramer, P. A. Hipskind, H. Boshoff, M. Bailey, T. Alling, J. Ollinger, T. Parish, and M. J. Miller, ACS Med. Chem. Lett., 2013, 4, 675. CrossRef
9. J. Ollinger, M. A. Bailey, G. C. Moraski, A. Casey, S. Florio, T. Alling, M. J. Miller, and T. Parish, PLoS One, 2013, 8, e60531. CrossRef
10. S. Kang, R.Y. Kim, M. J. Seo, S. Lee, Y. M. Kim, M. Seo, J. Seo, Y. Ko, I. Choi, J. Jang, J. Nam, S. Park, H. Kang, H. J. Kim, J. Kim, S. Ahn, K. Pethe, K. Nam, Z. No, and J. Kim, J. Med. Chem., 2014, 57, 5293. CrossRef
11. K. A. Abrahams, J. A. Cox, V. L. Spivey, N. J. Loman, M. J. Pallen, C. Constantinidou, R. Fernández, C. Alemparte, M. J. Remuiñán, D. Barros, L. Ballell, and G. S. Besra, PLoS One, 2012, 7, e52951. CrossRef
12. K. Pethe, P. Bifani, J. Jang, S. Kang, S. Park, S. Ahn, J. Jiricek, J. Jung, H. K. Jeon, J. Cechetto, T. Christophe, H. Lee, M. Kempf, M. Jackson, A. J. Lenaerts, H. Pham, V. Jones, M. J. Seo, Y. M. Kim, M. Seo, J. J. Seo, D. Park, Y. Ko, I. Choi, R. Kim, S. Y. Kim, S. Lim, S. A. Yim, J. Nam, H. Kang, H. Kwon, C. T. Oh, Y. Cho, Y. Jang, J. Kim, A. Chua, B. H. Tan, M. B. Nanjundappa, S. P. Rao, W. S. Barnes, R. Wintjens, J. R. Walker, S. Alonso, S. Lee, J. Kim, S. Oh, T. Oh, U. Nehrbass, S. J. Han, Z. No, J. Lee, P. Brodin, S. N. Cho, K. Nam, and J. Kim, Nat. Med., 2013, 19, 1157. CrossRef
13. Y. Cheng, G. C. Moraski, J. Cramer, M. J. Miller, and J. S. Schorey, PLoS One, 2014, 9, e87483. CrossRef
14. K. C. Chunavala, G. Joshi, E. Suresh, and S. Adimurthy, Synthesis, 2011, 635. CrossRef