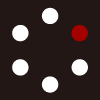
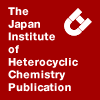
HETEROCYCLES
An International Journal for Reviews and Communications in Heterocyclic ChemistryWeb Edition ISSN: 1881-0942
Published online by The Japan Institute of Heterocyclic Chemistry
e-Journal
Full Text HTML
Received, 19th August, 2015, Accepted, 8th October, 2015, Published online, 15th October, 2015.
DOI: 10.3987/COM-15-13307
■ Facile One-Pot Synthesis of Cyclic N-Sulfonylamidines from Lactam and Sulfonamide
Ayinigeer Mulati and Abudureheman Wusiman*
School of Chemistry & Chemical Engineering, Xinjiang Normal University, Xinyi Road No 102, Urumqi, Xinjiang 830054, China
Abstract
A simple method has been developed for one-pot synthesis of five-, six- and seven-membered cyclic N-sulfonylamidines from lactams and sulfonamides under mild conditions. The method involves a Vilsmeier like reaction promoted by phosphoryl chloride. Detailed synthetic studies showed that the corresponding substituted N-sulfonylamidines were obtained in moderate to good yields.Amidines contain structural motifs with fascinating chemical properties and have been widely used in medicinal chemistry,1 coordination chemistry,2 and synthetic chemistry.3 They also occur widely in bioactive natural products.4 Classical methods for synthesizing amidine derivatives are based on transforming the functional groups of amides,5 isocyanides,6 nitriles,7 and aldoximes.8 In recent years several new protocols have been developed to prepare N-sulfonylamidines: these protocols include convergent direct coupling of Cu-catalyzed, three-component reactions of a terminal alkyne, sulfonyl azide, and amine;9 oxidative dehydration of tertiary amines and tandem reaction with sulfonylazides;10 three-component aerobic oxidative coupling of a terminal alkyne, amine, and sulfonamide;11 and others.12 Efficient synthesis of cyclic N-sulfonylamidines has also been achieved. It has been established earlier that these products were formed via nucleophilic displacement by the appropriate sulfonamide in lactim ether (Scheme 1, Route A).13 More recently, Chiba et al. developed a way to couple cyclic thioamides and sulfonyl azides, generating cyclic N-sulfonylamidines (Scheme 1, Route B).14 Bicyclic N-sulfonyl- amidines have also been reported via intramolecular one pot reaction,15 and cyclic N-sulfonylamidines with a protected nitrogen at the N-amino position can be accessed by the direct-coupling methods.9c,10d
Despite these advances, direct transformation of lactams to sulfonylamidines frequently involves complicated functional group transformations.5,7,8 This highlights the need for simpler, more efficient synthetic methods to generate sulfonylamidine derivatives. Here we describe our efforts to achieve one-pot synthesis of cyclic N-sulfonylamidines (Scheme 1, Route C). Phosphoryl chloride triggers a reaction involving a Vilsmeier-type complex.16 To the best of our knowledge, this is the first report of using lactam and sulfonamide to prepare cyclic N-sulfonylamidines.
We commenced our study by mixing butyrolactam (1a) and p-toluenesulfonamide (2a) in a 1:1 molar ratio in the presence of 1.0 equiv of POCl3 in chlorobenzene (PhCl), which afforded the desired sulfonylamidine (3a) in 40% yield (Table 1, entry 1). Replacing the 1 equiv of POCl3 with 1 equiv of PCl5 led to 30% yield (Table 1, entry 2). The reaction did not proceed well in the presence of other chlorinating reagents, such as oxalyl dichloride and thionyl chloride (Table 1, entries 3 and 4). Screening solvents showed that PhCl gave the best results, and that yield was no higher with other common solvents, including dichloromethane (DCM), 1,2-dichloroethane (DCE), toluene (PhMe) and chloroform (Table 1, entries 5-8). Starting from the original acidic reaction conditions, we tested various organic and inorganic bases, including pyridine, triethylamine (TEA), NaOH, K2CO3 and Cs2CO3 (Table 1, entries 9-13). Using 5 equiv of TEA gave good yield (60%; Table 1, entry 12). Using only 3.0 equiv of TEA (Table 1, entry 14) or reducing the temperature to 60 °C (Table 1, entry 15) decreased yield and prolonged reaction time. Increasing the molar ratio of butyrolactam improved yield (Table 1, entry 17), while increasing the amount of POCl3 to 1.5 equiv did not (Table 1, entry 18).
To further enhance yield, we tested a two-step reaction process. In the first step, a cyclic imidoyl- chloride17 was prepared by mixing 1a and POCl3 at room temperature. To this mixture we added 2a and TEA and incubated the reaction for 12 h at 80 °C. We obtained only 40% yield of product (Table 1, entry 19). Therefore, we defined the optimal reaction conditions as the one-pot combination of 1a and 2a (1.2:1) in the presence of 1.0 equiv of POCl3 and 5.0 equiv of TEA in PhCl at 80 °C for 5 h under ambient air (Table 1, entry 17).
Using these optimal reaction conditions, we explored the scope of the annulation using various lactams (1a-1i) and sulfonamides (2a-2n). Lactam ring size clearly affected the reaction (Table 2): butyrolactam (1a) required much longer reaction time and gave lower yield than valero- (1b) and caprolactams (1c) (Table 2, entries 2, 8 and 12). Substrates with electron-donating groups such as -Me and -OMe on the phenyl ring provided the corresponding sulfonylamidines in good yields (Table 2, entries 1, 3, 7, 11 and
13). In contrast, substrates with an electron-withdrawing substituent such as -NO2 in the para position gave substantially lower product yields (Table 2, entries 5 and 15). Notably, the bulky and strongly electron-withdrawing trifluoromethyl group led to relatively low yield of the corresponding amidine (Table 2, entries 6, 10 and 16). The even more sterically hindered tert-butylsulfonamide group was not tolerated under the optimal reaction conditions (Table 2, entry 17).
These results, together with studies in the literature18 lead us to propose the following mechanism for the formation of N-sulfonylamidines (Scheme 2). First, the lactam (1) can be activated by POCl3 to form Vilsmeier complex A. Then attack by nucleophilic sulfonamide (2) on A leads to the formation of intermediate B, which loses a molecule of HOPOCl2 to become C. At last, this intermediate traps HCl to provide the sulfonylamidine (3).
The structure of compound 3c was confirmed by single-crystal X-ray diffraction analysis (Figure 1).19 It is interesting to note that the lengths of the two C-N bonds [N1-C1, 1.316(2) Å; N2-C1, 1.315(2) Å] in the generated amidines are similar, this indicates the delocalized nature of the C-N double bond.
In summary, we have described a convenient one-pot method for synthesizing cyclic N-sulfonylamidines from inexpensive commercially available reagents such as lactam, sulfonamide and POCl3. Moderate to good product yields are obtained under mild conditions.
EXPERIMENTAL
Dichloromethane (DCM), PhCl, PhMe, and 1,2-dichloroethane (DCE) were distilled from CaH2. Other solvents and commercial reagents were used without additional purification. Reaction products were purified by flash column chromatography using 200-300 mesh silica gel. NMR spectra were obtained on a Varian spectrometer. Single-crystal X-ray diffraction was carried out using monochromatic Mo Kα radiation (λ = 0.71073 Å) at 296(2) K.
General procedure for preparing cyclic N-sulfonylamidine 3a–3o: A solution of phosphoryl chloride (2.0 mmol, 306.6 mg, 1.0 equiv) in PhCl (3.0 mL) was added dropwise to a mixture of the appropriate lactam 1 (2.4 mmol, 1.2 equiv) and sulfonamide 2 (2.0 mmol, 1.0 equiv) in PhCl (7.0 mL) at room temperature. After the addition of phosphoryl chloride, the TEA (10.0 mmol, 1.01 g, 5.0 equiv) was added to the mixture in one portion and the reaction was stirred at 80 oC for the indicated time (see Table 2). It was then the reaction mixture was cooled to room temperature and the solvent evaporated under vacuum. The resulting residue was purified by silica gel column chromatography to give the desired product.
N-(2-Pyrrolidon-2-yl)toluenesulfonamide (3a)20 white solid: mp 149–150 oC; 1H NMR (400 MHz, CDCl3): δ (ppm) 7.99 (brs, 1H), 7.80 (d, J = 8.2 Hz, 2H), 7.27 (d, J = 8.2 Hz, 2H), 3.59 (t, J = 7.2 Hz, 2H), 2.68 (t, J = 8.1 Hz, 2H), 2.40 (s, 3H), 2.10–2.02 (m, 2H). 13C NMR (100 MHz, CDCl3): δ (ppm) 171.5, 142.6, 139.5, 129.3, 126.4, 46.3, 32.9, 21.4, 20.0.
N-(2-Pyrrolidon-2-yl)benzenesulfonamide (3b)13 white solid: mp 155–156 oC; 1H NMR (400 MHz, CDCl3): δ (ppm) 8.11 (brs, 1H), 7.91 (d, J = 7.2 Hz, 2H), 7.54–7.44 (m, 3H), 3.58 (t, J = 7.2 Hz, 2H), 2.70 (t, J = 8.0 Hz, 2H), 2.05 (p, J = 7.6, 2H). 13C NMR (100 MHz, CDCl3): δ (ppm) 171.6, 142.4, 132.2, 128.8, 126.5, 46.6, 33.2, 20.8.
4-Methoxy-N-(2-pyrrolidon-2-yl)benzenesulfonamide (3c)20 white solid: mp 146–147 oC; 1H NMR (400 MHz, CDCl3): δ (ppm) 8.03 (brs, 1H), 7.84 (d, J = 8.9 Hz, 2H), 6.93 (d, J = 8.9 Hz, 2H), 3.84 (s, 3H), 3.58 (t, J = 7.1 Hz, 2H), 2.68 (t, J = 8.0 Hz, 2H), 2.12–1.98 (m, 2H). 13C NMR (100 MHz, CDCl3): δ (ppm) 171.1, 162.6, 134.4, 128.6, 114.1, 55.7, 46.7, 33.3, 20.1.
N-(2-Pyrrolidon-2-yl)methanesulfonamide (3d)20 white solid: mp 145–146 oC; 1H NMR (400 MHz, CDCl3): δ (ppm) 7.73 (brs, 1H), 3.60 (t, J = 7.1 Hz, 2H), 2.98 (s, 3H), 2.68 (t, J = 7.6 Hz, 2H), 2.12–2.05 (m, 2H). 13C NMR (100 MHz, CDCl3): δ (ppm) 170.8, 46.6, 41.9, 33.2, 19.8.
4-Nitro-N-(2-pyrrolidon-2-yl)benzenesulfonamide (3e) yellow solid: mp 195 oC (decompose); 1H NMR (400 MHz, DMSO-d6): δ (ppm) 9.25 (brs, 1H), 8.35 (d, J = 8.8 Hz, 2H), 8.03 (d, J = 8.8 Hz, 2H), 3.40 (t, J = 7.1 Hz, 2H), 2.75 (t, J = 8.0 Hz, 2H), 2.03–1.95 (m, 2H). 13C NMR (100 MHz, DMSO-d6): δ (ppm) 172.83, 149.02, 148.87, 127.43, 124.21, 44.95, 31.45, 20.32. ESI-MS calcd for MNa+, C10H11N3NaO4S: 292.0368; found 292.0365.
N-(2-Pyrrolidon-2-yl)trifluoromethanesulfonamide (3f) white solid: mp 149–150 °C; 1H NMR (400 MHz, CDCl3): δ (ppm) 8.53 (brs, 1H), 3.68 (t, J = 7.2 Hz, 2H), 2.94 (t, J = 8.1 Hz, 2H), 2.25–2.17 (m, 2H). 13C NMR (100 MHz, CDCl3): δ (ppm) 176.79, 120.01 (q, J = 322 Hz, CF3) 46.81, 33.02, 20.28. ESI-MS calcd for MNa+, C5H7F3N2NaO2S: 239.0078; found 239.0072.
N-(2-Piperidon-2-yl)toluenesulfonamide (3g) white solid: mp 129–130 oC; 1H NMR (400 MHz, CDCl3): δ (ppm) 8.62 (brs, 1H), 7.82 (d, J = 7.6 Hz, 2H), 7.40 (d, J = 8.0 Hz, 2H), 3.34 (d, J = 6.8 Hz, 2H), 2.45 (t, J = 5.8 Hz, 2H), 2.37 (s, 3H), 1.73–1.70 (m, 4H). 13C NMR (100 MHz, CDCl3): δ (ppm) 166.30, 142.74, 140.11, 129.48, 126.47, 42.60 (s), 31.03, 21.69, 21.55, 19.50. ESI-MS calcd for MNa+, C12H16N2NaO2S: 275.0830; found 275.0831.
N-(2-Piperidon-2-yl)benzenesulfonamide (3h)13 white solid: mp 133–134 oC; 1H NMR (400 MHz, CDCl3): δ (ppm) 8.54 (brs, 1H), 7.92 (d, J = 7.6, 2H), 7.53–7.44 (m, 3H), 3.38 (d, J = 7.0 Hz, 2H), 2.48 (t, J = 6.4 Hz, 2H), 1.77 (t, J = 7.2, 7.5 Hz, 4H). 13C NMR (100 MHz, CDCl3): δ (ppm) 166.4, 142.9, 132.0, 128.8, 126.3, 42.5, 31.0, 21.4, 19.4.
N-(2-Piperidon-2-yl)methanesulfonamide (3i)13 white solid: mp 104–105 oC; 1H NMR (400 MHz, CDCl3): δ (ppm) 8.23 (bs, 1H), 3.34 (t, J = 7.0 Hz, 2H), 2.93 (s, 3H), 2.45 (t, J = 7.1 Hz, 2H), 1.77 (m, 4H). 13C NMR (100 MHz, CDCl3): δ (ppm) 165.91, 42.52, 31.02, 21.53, 19.49.
N-(2-Piperidon-2-yl)trifluoromethanesulfonamide (3j) white solid: mp 97–98 oC; 1H NMR (400 MHz, CDCl3): δ (ppm) 8.73 (br s, 1H), 3.46 (d, J = 2.4 Hz, 2H), 2.68 (d, J = 5.8 Hz, 2H), 1.87–1.81 (m, 4H). 13C NMR (100 MHz, CDCl3): δ (ppm) 170.39, 119.01 (q, J = 320 Hz, CF3), 42.09, 29.66, 19.94, 17.88. ESI-MS calcd for MNa+, C6H9F3N2NaO2S: 253.0235; found 253.0229.
N-(1-Aza-2-cycloheptanon-2-yl)toluenesulfonamide (3k) white solid: mp 94–95 oC; 1H NMR (400 MHz, CDCl3): δ (ppm) 8.56 (brs, 1H), 7.78 (d, J = 8.2 Hz, 2H), 7.24 (d, J = 8.2 Hz, 2H), 3.32 (dd, J = 9.9, 5.7 Hz, 2H), 2.45 (t, J = 5.2 Hz, 2H), 2.37 (s, 3H), 1.72–1.59 (m, 6H). 13C NMR (100 MHz, CDCl3): δ (ppm) 172.07, 142.88, 139.73, 129.46, 126.49, 44.80, 36.92, 30.18, 28.82, 23.83, 21.69. ESI-MS calcd for MNa+, C13H18N2NaO2S: 289.0987, found 289.0998.
N-(1-Aza-2-cycloheptanon-2-yl)benzenesulfonamide (3l)13 white solid: mp 100–101 oC; 1H NMR (400 MHz, CDCl3): δ (ppm) 8.58 (brs, 1H), 7.91 (d, J = 6.8 Hz, 2H), 7.53–7.44 (m, 3H), 3.34 (dd, J = 10, 4.4 Hz, 2H), 2.48 (t, J = 5.6 Hz, 2H), 1.74–1.60 (m, 6H). 13C NMR (100 MHz, CDCl3): δ (ppm) 172.52, 142.56, 132.15, 128.77, 126.37, 44.75, 36.82, 30.09, 28.71, 23.74.
4-Methoxy-N-(1-aza-2-cycloheptanon-2-yl)benzenesulfonamide (3m) white solid: mp 119–120 oC; 1H NMR (400 MHz, CDCl3): δ (ppm) 8.53 (brs, 1H), 7.85 (d, J = 8.8 Hz, 2H), 6.93 (d, J = 8.8 Hz, 2H), 3.83 (s, 3H), 3.33 (dd, J = 10, 3.6 Hz, 2H), 2.46 (t, J = 3.2 Hz, 2H), 1.74–1.63 (m, 6H). 13C NMR (100 MHz, CDCl3) δ (ppm) 171.85, 162.63, 134.59, 128.55, 114.02, 55.73, 44.80, 36.94, 30.21, 28.88, 23.88. ESI-MS calcd for MNa+, C13H18N2NaO3S: 305.0930; found 305.0936.
N-(1-Aza-2-cycloheptanon-2-yl)methanesulfonamide (3n)13 white solid: mp 83–84 oC; 1H NMR (400 MHz, CDCl3) δ (ppm) 8.26 (brs, 1H), 3.31 (t, J = 2.8 Hz, 2H), 2.94 (s, 3H), 2.45 (t, J = 5.2 Hz, 2H), 1.74–1.62 (m, 6H). 13C NMR (100 MHz, CDCl3) δ (ppm) 171.99, 44.81, 42.36, 36.90, 30.24, 28.85, 23.95.
4-Nitro-N-(1-aza-2-cycloheptanon-2-yl)benzenesulfonamide (3o) yellow solid: mp 163 oC (decompose); 1H NMR (400 MHz, CDCl3): δ (ppm) 8.58 (brs, 1H), 8.31 (d, J = 8.8Hz, 2H), 8.10 (d, J = 8.8 Hz, 2H), 3.39 (dd, J = 10, 3.6 Hz, 2H), 2.51 (t, J = 5.2 Hz, 2H), 1.80–1.65 (m, 6H). 13C NMR (100 MHz, CDCl3): δ (ppm) 172.83, 149.67, 148.14, 127.60, 123.96, 44.85, 36.76, 29.94, 28.42, 23.54. ESI-MS calcd for MNa+, C12H15N3NaO4S 320.0681; found 320.0685.
N-(1-Aza-2-cycloheptanon-2-yl)trifluoromethanesulfonamide (3p) white solid: mp 66–67 oC; 1H NMR (400 MHz, CDCl3) δ (ppm) 8.74 (brs, 1H), 3.45 (dd, J = 9.6, 5.6 Hz, 2H), 2.64 (t, J = 5.2 Hz, 2H), 183–1.69 (m, 6H). 13C NMR (100 MHz, CDCl3) δ (ppm) 177.26, 119.75 (q, J= 322 Hz, CF3), 45.34, 36.55, 30.05, 27.79, 23.20. ESI-MS calcd for MNa+, C7H11F3N2NaO2S: 267.0391; found 267.0391.
ACKNOWLEDGEMENTS
This work was supported by the National Natural Science Foundation of China (21362038), and the China Scholarship Council (CSC).
References
1. (a) G. V. Boyd, In “The Chemistry of Amidines and Imidates”, ed. by S. Patai and Z. Rappoport, Wiley, New York, 1991, Vol. 2, Chapter 8; (b) M. Y. Lee, M. H. Kim, J. Kim, S. H. Kim, B. T. Kim, I. H. Jeong, S. Chang, S. H. Kim, and S.-Y. Chang, Bioorg. Med. Chem. Lett., 2010, 20, 541. CrossRef
2. J. Barker and M. Kilner, Coord. Chem. Rev., 1994, 133, 219. CrossRef
3. T. Kumamoto, In “Superbases for Organic Synthesis: Guanidines, Amidines, Phosphazenes and Related Organoctalysts”, ed. by I. Ishikawa, John Wiley & Sons Press, West Sussex, UK, 2009, p 295. CrossRef
4. (a) J. V. Greenhill and P. Lue, Prog. Med. Chem., 1993, 30, 203; CrossRef (b) R. G. S. Berlinck and M. H. Kossuga, Nat. Prod. Rep., 2005, 22, 516; CrossRef (c) R. G. S. Berlinck, A. C. B. Burtoloso, and M. H. Kossuga, Nat. Prod. Rep., 2008, 25, 919. CrossRef
5. N. Kumagai, S. Matsunaga, and M. Shibasaki, Angew. Chem. Int. Ed., 2004, 43, 478. CrossRef
6. (a) C. King, J. Org. Chem., 1960, 25, 352; CrossRef (b) P. Jakobsen and S. Treppendahl, Tetrahedron, 1977, 33, 3137. CrossRef
7. U. E. W. Lange, B. Schafer, D. Baucke, E. Buschmann, and H. Mack, Tetrahedron Lett., 1999, 40, 7067. CrossRef
8. T. Takuwa, T. Minowa, J. Y. Onishi, and T. Mukaiyama, Bull. Chem. Soc. Jpn., 2004, 77, 1717. CrossRef
9. (a) I. Bae, H. Han, and S. Chang, J. Am. Chem. Soc., 2005, 127, 2038; CrossRef (b) E. J. Yoo, I. Bae, S. H. Cho, H. Han, and S. Chang, Org. Lett., 2006, 8, 1347; CrossRef (c) S. Chang, M. J. Lee, D. Y. Jung, E. J. Yoo, S. H. Cho, and S. K. Han, J. Am. Chem. Soc., 2006, 128, 12366; CrossRef (d) X. Xu, D. Cheng, J. Li, H. Guo, and J. Yan, Org. Lett., 2007, 9, 1585; CrossRef (e) S. H. Cho and S. Chang, Angew. Chem. Int. Ed., 2008, 47, 2836; CrossRef (f) E. J. Yoo, M. Ahlquist, I. Bae, K. B. Sharpless, V. V. Fokin, and S. Chang, J. Org. Chem., 2008, 73, 5520; CrossRef (g) E. J. Yoo and S. Chang, Org. Lett., 2008, 10, 1163. CrossRef
10. (a) X. Xu, X. Li, L. Ma, N. Ye, and B. Weng, J. Am. Chem. Soc., 2008, 130, 14048; CrossRef (b) S. Wang, Z. Wang, and X. Zheng, Chem. Commun., 2009, 45, 7372; CrossRef (c) N. Liu, B.-Y. Tang, Y. Chen, and L. He, Eur. J. Org. Chem., 2009, 2059; CrossRef (d) X. Xu, Z. Ge, D. Cheng, L. Ma, C. Lu, Q. Zhang, N. Yao, and X. Li, Org. Lett., 2010, 12, 897; CrossRef (e) L. Zhang, J.-H. Su, S. Wang, C. Wan, Z. Zha, J. Du, and Z. Wang, Chem. Commun., 2011, 47, 5488. CrossRef
11. J. Kim and S. S. Stahl, J. Org. Chem., 2015, 80, 2448. CrossRef
12. (a) S. Chen, Y. Xu, and X. Wan, Org. Lett., 2011, 13, 6152; CrossRef (b) M. Yao and C.-D. Lu, Org. Lett., 2011, 13, 2782; CrossRef (c) J. Chen, Y.-P. Guo, M.-H. Sun, G.-T. Fan, and L. Zhou, Chem. Commun., 2014, 50, 12367. CrossRef
13. (a) V. A. Plit and S. I. Burmistrov, Ukr. Khim. Zh., 1958. 24, 467; (b) K. Maier, H. Baumann, and D. Leuchs, German Patent, 1960, 1085160 (Chem. Zentr., 1961, 132, 4524).
14. M. Aswad, J. Chiba, T. Tomohiro, and Y. Hatanaka, Chem. Commun., 2013, 49, 10242. CrossRef
15. (a) J. J. Krutak, R. D. Burpitt, W. H. Moore, and J. A. Hyatt, J. Org. Chem., 1979, 44, 3847; CrossRef (b) N. Tomita, D. Tomita, Y. Tominari, S. Imamura, S. Morimoto, T. Kojima, M. Toyofuku, Y. Hattori, T. Kaku, and M. Ito, WO 2013118845 A1.
16. H. H. Bosshard, R. Mory, M. Schmid, and H. Zollinger, Helv. Chim. Acta, 1959, 42, 1653. CrossRef
17. (a) I. Hermecz, L. Vasvari-Debreczy, A. Horvath, M. Balogh, J. Kokosi, C. DeVos, and L. Rodriguez, J. Med. Chem., 1987, 30, 1543; CrossRef (b) E. Vilsmaier, G. Kristen, and C. Tetzlaff, J. Org. Chem., 1988, 53, 1806. CrossRef
18. (a) H. Bredereck and K. Bredereck, Chem. Ber., 1961, 94, 2278; CrossRef (b) H. Bredereck, R. Gompper, K. Klemm, and H. Rempfer, Chem. Ber., 1959, 92, 837. CrossRef
19. X-Ray data deposited at the Cambridge Crystallographic Data Centre under CCDC 1409965 Unit cell parameters: a 6.533(2) b 7.642(3) c 12.602(4), space group triclinic P-1. Experimental and refinement details can be found in the Supplementary Data.
20. A. Wusiman, X. Tusun, and C.-D. Lu, Eur. J. Org. Chem., 2012, 3088. CrossRef