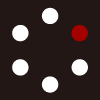
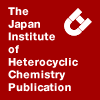
HETEROCYCLES
An International Journal for Reviews and Communications in Heterocyclic ChemistryWeb Edition ISSN: 1881-0942
Published online by The Japan Institute of Heterocyclic Chemistry
e-Journal
Full Text HTML
Received, 16th October, 2015, Accepted, 26th November, 2015, Published online, 18th December, 2015.
DOI: 10.3987/COM-15-13346
■ Solvent Extraction Ability of New Diethyl Phosphate-Modified Thiacalix[6]arene towards PGM Solution
Kunda Uma Maheswara Rao, Takashi Kimuro, Manabu Yamada, Yoshihiko Kondo, and Fumio Hamada*
Department of Applied Chemistry, Akita University, Tegata-gakuenncho 1-1, Akita 010-0852, Japan
Abstract
New lower rim modified phosphorylated thiacalix[6]arene derivative such as TC6A-phosphate (2) was synthesized. IR, 1H & 31P NMR and mass spectral and elemental analysis characterized the structure of the synthesized compound. We also investigated the metal extraction ability of the newly synthesized compound through solvent extraction technique from automotive catalyst residue, standard mixed metal, standard Pd and Zr solutions. This compound showed high selectivity towards Zr and Pd ions.INTRODUCTION
In recent years, great attention has been focused on derivatization of thiacalixarenes due to their unique properties in various sectors and structural similarity to the corresponding calixarenes.1 Because of difficulties in the synthesis of thiacalix[6/8]arenes, very few reports were published on their derivatives.2 Hence there is great demand for the synthesis of thiacalix[6/8]arene derivatives. Among the various properties of thiacalixarenes, precious metal separation from the mixture of metals through solvent extraction technique is one of the major applications, which makes these materials as significant scaffolds in supramolecular chemistry. In continuation of our interest in the development of thiacalixarene chemistry, we successfully synthesized new diethyl phosphate-modified thiacalix[6]arene and investigated its solvent extraction behavior towards automotive catalytic residue (ACR) solution.
ACR solution was prepared from secondary resources,3 which includes nine metals. Among these, Pd, Pt and Rh, collectively called platinum group metals (PGMs), are the precious metals based on their production and demand. Separation of PGMs from this solution is challenging objective. However commercial extractants such as dioctyl sulfide, dihexyl sulfide for Pd, tri-n-octylamine, tributyl phosphate for Pt4 are using for precious metals separation since last two decades,5 the poor selectivity and extractability of these extractants opens up the challenging research to determine the efficient extractant. In this context, many reports were published with several extractants. p-tert-Butylthiacalix[n]arenes (n = 4, 6, 8) can extract metals from the mixed metal solution but with low selectivity.6 Modification on upper or lower rim of these compounds results increases in selectivity of extracting metals. Acetylthiomethylthiacalix[6]arene extracts 86% of Pd from ACR solution along with < 20% of other metals.7 p-tert-Butylhexakis[(carboxyl)methoxy]thiacalix[6]arene extracts 74%, 90% of Pd, Zr respectively. p-tert-Butylhexamethoxythiacalix[6]arene and p-tert-butylhexaethoxythiacalix[6]arene also extract Pd and Zr selectively from ACR solution.8 Dimethylthiacarbomyl-modified thiacalix[n]arenes (n = 4, 6) selectively extract Pd along with traces of Zr from ACR solution.9 Majority of these reports particularly with p-tert-butylthiacalix[6]arene (TC6A) derivatives exposes that Zr was extracted predominantly than Pd. But the reason for Zr domination was not explained clearly.
Review of literature reveals that, to till date, there is no such extractant that can efficiently separate precious metals from the ACR solution in industrial level. Hence extensive research is required to determine the efficient extractant. In addition to our recent reports on this emergent research, the current paper will contribute to some extent to achieve this goal.
RESULTS AND DISCUSSION
In our previous work, we reported the synthesis of diethyl phosphate-modified thiacalix[4]arene which showed high selectivity towards Pd (II) ions from the ACR solution.10 Now we would like report here the synthesis and solvent extraction behavior of diethyl phosphate-modified thiacalix[6]arene. Reflux of TC6A (1) with diethyl chlorophosphate in the presence of potassium carbonate in acetone for 30 h yields diethyl phosphate-modified TC6A (2) (Scheme 1). Further we studied the extraction behavior of 2 towards ACR, standard PGM, Pd and Zr solutions.
Liquid-liquid extraction of 2 using ACR solution:
We studied the liquid-liquid extraction of metals from ACR solution using 2. Compound 2 showed high selectivity towards Zr. 99.35% of Zr was extracted with 2 (Figure 1) from ACR solution and no other metal was found along with it. We surprised about the result because diethyl phosphate-modified thiacalix[4]arene selectively extracted Pd along with 18.6% of Zr from ACR solution. We proceed to further experiments with 2, but the reason for high selectivity towards Zr remains unclear.
Further, we studied liquid-liquid extraction of 2 using standard PGM solution without Zr at various pH ranging from 0-3 and the results were showed in Figure 2. Compound 2 was extracted 99.98% of Pd at pH 3 along with another metal in trace levels (less than 10%). Literature survey reveals that, Pd metal at pH 3 is in the form of PdCl42-, which is more favorable species for extraction with TCnAs.9 This may be the reason for significant extraction of Pd at pH 3 with compound 2.
In addition to this, we also carried out liquid-liquid extraction experiments with Pd and Zr standard solutions using 2 to investigate the suitable conditions for extraction.
Liquid-liquid extraction with Pd standard solution:
Effect of concentration of 2:
The E% of 2 with various concentrations ranging from 0.2 – 2 mM towards Pd standard solution was studied at pH 3 with fixed contact time of 24 h. The E% of 2 gradually increased (Figure 3) and 100% extraction was occurred with 2 mM concentration.
Jobs Continuous Variation Method:
The favorable complexation formed between 2 and Pd was determined using Jobs continuous variation method. Same concentrations of 2 in chloroform (1 mM) and PdCl2 (1 mM, pH 3) in HCl were mixed in different ratios. The solutions were shaken for 24 h and 300 strokes/min, then the layers were separated and the absorbance of the organic phase was determined using UV-visible spectrophotometry at 390 nm. The results were plotted as absorbance (A) versus mole fraction of 2 (Figure 4). The 2-Pd complex exhibited maximum absorbance at 0.66 mole fraction, which indicates that the two molecules of 2 coordinate one Pd.
Liquid-liquid extraction with Zr standard solution:
Effect of HCl concentration:
The effect of HCl concentration on the extraction of Zr with 2 was studied using 1 mM ZrCl2 standard solution prepared with various HCl concentrations ranging from 8N to 0.001N. The extraction ability of 2 highly depends on the concentration of HCl. Significant extraction was observed at lower concentrations. 100% Extraction was obtained with 0.001 N HCl (Figure 5). The Zr extraction experiments with organophosphorus extractants indicates the complexation between host and guest due to high affinity of phosphorus compounds towards Zr is responsible for significant extraction of Zr at lower concentrations of HCl.11
Effect of contact time:
The effect of contact time on the extraction of Zr with 2 was determined by varying the time in the range of 1 h to 42 h (Figure 6). The E% values were highly depends on the contact time. As the contact time increases, the E% also increased and 100% extraction was observed at 24 h. Hence contact time for further studies was fixed as 24 h.
Effect of concentration of 2:
The E% of 2 with various concentrations ranging from 0.2 – 1 mM towards 1 mM ZrCl2 standard solution in 0.001 N HCl was studied with fixed contact time of 24 h. The E% of 2 gradually increased and 100% extraction was occurred with 1 mM concentration of 2 (Figure 7).
CONCLUSIONS
In conclusion, we synthesized new lower rim phosphate modified TC6A derivative and investigated its extractability towards rare metal ions from ACR and model PGM solutions. The compound showed high selectivity towards Zr and Pd metal ions. Further we also examined the extraction behavior of the synthesized compound towards Zr and Pd metal ions using their standard solutions.
EXPERIMENTAL
Experimental procedure for the synthesis of 2:
To a suspension of 1 (0.0005 mol) in acetone (20 mL), K2CO3 (0.0075 mol) was added. Then, diethyl chlorophosphate (0.012 mol) in 10 mL of acetone was added dropwise. The mixture was refluxed for 30 h. The progress of the reaction was monitored using thin layer chromatography (TLC). After cooling down to room temperature, the solvent was removed under reduced pressure. The resultant material was added to CHCl3, and then washed with 1N HCl solution. The solvent was removed under reduced pressure. The crude product was obtained, which was recrystallized from 1:3 CHCl3 and n-hexane to give pure product 2.
Spectral data of 2:
Yield: 65.8%; White solid; 1H NMR (600 MHz, CDCl3): 7.51 (s, 1H, Ar), 6.65 (s, 1H, Ar), 4.39-4.36 (m, 4H, P(O)CH2CH3), 1.41-1.24 (m, 15H); 13C NMR (150 MHz, CDCl3): 155.26, 148.64, 135.25, 120.38, 64.87 (CH2-P(O)), 34.22 (C(CH3)3), 31.28 (C(CH3)3), 16.20 (CH3CH2-P(O)); 31P NMR (600 MHz, CDCl3): -5.41; FT-IR: 1272.98 (P=O); MALDI-TOF MS: 1896.98 (M+), 1918.94 (M+Na). Anal. Calcd for C84H126O24P6S6 (%): C, 53.15; H, 6.69. Found: C, 52.64; H, 6.36.
Liquid – liquid extraction with PGM solution:
PGM solution containing nine metal ions, namely, Al, Ba, Ce, Pd, Pt, Rh, La, Zr and Y was diluted 10 times with water and used for the liquid liquid extraction study. The pH of the diluted PGM solution was 0.78. The concentrations of metal cations in the diluted solution were determined by inductively coupled plasma atomic emission spectrometry (ICP-AES) and listed in ESI Table 1.
In a 50 mL of tube, 5 mL of 2 (1 mM) in CHCl3 was mixed with the diluted PGM solution (5 mL). The mixture was then shaken at 300 strokes/min for 24 h. The concentration of metal ions remaining in the aqueous phase was determined by ICP-AES.
Liquid – liquid extraction with model PGM solution:
A model PGM solution was prepared containing Al, Ba, Pd, Pt, Rh, La, Y and Ce with their corresponding chloride salts. The concentration of metal cations in the solution was determined by ICP-AES and listed in ESI Table 2. The pH of this solution was adjusted to 0, 1, 2 and 3 with saturated NaOH solution.
In a 50 mL of tube, 5 mL of 2 (2 mM) in CHCl3 was mixed with the model PGM solution (5 mL). The mixture was then shaken at 300 strokes/min. for 24 h. The concentration of metal ions remaining in the aqueous phase was determined by ICP-AES.
Liquid-liquid extraction of Pd and Zr ions:
Liquid-liquid extraction studies were performed in triplicate at room temperature with corresponding concentrations of 2 (5 mL) in CHCl3 and 1 mM PdCl2 or ZrCl2 in HCl (5 mL) in glass bottles. The mixture was shaken at 300 strokes/min for desired time. The concentration of Pd or Zr ions in the aqueous phase was measured using ICP-AES.
The E% was calculated using the following equation.
Where C1 and C2 are initial and final concentration (mM) of metal ion in aqueous layer, respectively.
References
1. (a) P. Lhotak, Eur. J. Org. Chem., 2004, 1675; CrossRef (b) N. Morohashi, F. Narumi, N. Iki, Tetustarohattori, and S. Miyano, Chem. Rev., 2006, 106, 5291; CrossRef (c) R. Kumar, Y. O. Lee, V. Bhalla, M. Kumar, and J. S. Kim, Chem. Soc. Rev., 2014, 43, 4824. CrossRef
2. (a) M. Ulzii, K. U. M. Rao, M. Yamada, and F. Hamada, Heterocycles, 2014, 89, 2554; CrossRef (b) M. Yamada, M. R. Gandhi, Y. Kondo, A. Shibayama, and F. Hamada, Sepa. Sci. Tech., 2015, 50, 1964; (c) M. R. Gandhi, M. Yamada, Y. Kondo, R. Sato, and F. Hamada, Ind. Eng. Chem. Res., 2014, 53, 2559. CrossRef
3. (a) S. Itoh, C. Li, M. Yamada, M. Akama, Y. Shimakawa, Y. Kondo, and F. Hamada, Int. J. Soc. Mater. Eng. Resour., 2010, 17, 211; CrossRef (b) M. Yamada, A. Shibayama, Y. Kondo, and F. Hamada, Int. J. Soc. Mater. Eng. Resour., 2007, 15, 13. CrossRef
4. (a) F. L. Bernardis, R. A. Grant, and C. D. Sherrington, React. Funct. Polym., 2005, 65, 205; CrossRef (b) H. Narita and M. Tanaka, J. MMIJ, 2011, 127, 175; CrossRef (c) J. Shibata and A. Okuda, MMIJ (Shigen-to-Sozai), 2002, 118, 1.
5. R. I. Edwards, W. A. M. te Riele, and G. J. Bernfield, Gmelin Handbook on Inorganic Chemistry, ed. by G. J. K. Acres, 1986, Vol. A1, Springer, Berlin, pp. 1-23.
6. (a) M. Yamada, A. Shibayama, Y. Kondo, and F. Hamada, Int. J. Soc. Mater. Eng. Resour., 2007, 15, 13; CrossRef (b) C. Li, Y. Yue, B. Liu, and S. Fan, Chinese J. Org. Chem., 2011, 31, 819.
7. S. Itoh, C. Li, M. Yamada, M. Akama, Y. Shimakawa, Y. Kondo, and F. Hamada, Int. J. Soc. Mater. Eng. Resour., 2010, 17, 211. CrossRef
8. Y. Kondo, A. Shibayama, F. Hamada, M. Yamada, M. Akama, and T. Imai, U. S. Patent 2010/0129277 A1, 2010.
9. M. Rajiv Gandhi, M. Yamada, Y. Kondo, R. Sato, and F. Hamada, Ind. Eng. Chem. Res., 2014, 53, 2559. CrossRef
10. K. U. M. Rao, M. Yamada, T. Kimuro, Y. Kondo, and F. Hamada, Heterocycles, 2015, 91, 989. CrossRef
11. (a) M. Taghizadeh, M. Ghandai, and E. Zolfonoum, J. Nucl. Mater., 2011, 412, 334; CrossRef (b) S. Biswas and S. Basu, J. Radioanal. Nucl. Chem., 1999, 242, 253; CrossRef (c) L. Y. Wang, H. Y. Lee, and M. S. Lee, Mater. Trans., 2013, 54, 1460. CrossRef