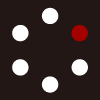
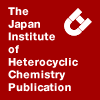
HETEROCYCLES
An International Journal for Reviews and Communications in Heterocyclic ChemistryWeb Edition ISSN: 1881-0942
Published online by The Japan Institute of Heterocyclic Chemistry
e-Journal
Full Text HTML
Received, 25th November, 2015, Accepted, 6th January, 2016, Published online, 15th January, 2016.
■ Design, Synthesis, Antitumor and Antimicrobial Activity of Some Novel 6,7-Dimethoxyquinazoline Derivatives
Asmaa E. Kassab,* Ehab M. Gedawy, Zienab Mahmoud, and Rania A. Khattab
Pharmaceutical Organic Chemistry Department, Faculty of Pharmacy, Cairo University, 33 Kaser El Ainin Street 11562, Egypt
Abstract
Novel 4-substituted-6,7-dimethoxyquinazolines 3, 4a and 4b were synthesized via reacting the corresponding 4-chloro derivative 2 with 2-(4-aminopiperazin-1-yl)ethanol, ethylpiperazine or benzylpiperidine. Quinazolines 6a-c and 8a-d were obtained through reacting 4-hydrazinylquinazoline 5 with different aromatic aldehydes or aromatic isothiocyanates. An attempt to synthesize 6,7-dimethoxyquinazolin-4-yl hydrazinecarboxamides via reacting the hydrazinyl derivative 5 with certain aromatic isocyanates was unsuccessful and the unexpected triazoloquinazoline 7 was obtained regardless to the isocyanate used .The anticancer activity of 4 compounds, namely 3, 4a, 4b and 7 was evaluated by National Cancer Institute (USA) at single dose (10-5 M) utilizing 59 different human tumor cell lines. Moreover, the antimicrobial activity of all the newly synthesized quinazolines was screened against Gram positive bacteria (Staphylococcus aureus and Bacillus subtilis), Gram negative bacteria (Escherichia coli and Klebsiella) and a fungal strain (Candida albicans).INTRODUCTION
Quinazolines are considered to be an important chemical synthon of various therapeutic efficacy and pharmaceutical utility. They possess variety of biological effects including anticancer1-13 and antimicrobial14-27 activities. Moreover, the quinazoline core is an integral part of numerous potential marketed anticancer agents for example gefitinib (IressaTM),28 and tandutinib (MLN518) (phase II clinical trials).29 It was reported that the thiosemicarbazide quinazoline hybrid I displayed promising antitumor potency (Figure 1).30
From Structure-based drug design point of view, quinazolines have proven to bind to the (epidermal growth factor receptor) EGFR ATP binding site. Accordingly, the interaction of quiazoline nucleus with this particular site indicated the importance of N-3 on the pyrimidine ring as a Hydrogen-bonded with a water molecule interacting with Thr 766 and the dialkyloxy substituents on the benzene one.31,32 Moreover, The arylidenhydrazinyl and thioureido moieties are well known to contribute to enhancement of antitumor activity.33 Furthermore, the quinazoline hybrids II,34 III35 and IV36 showed potent antimicrobial activity. Moreover, (AlbaconazoleTM) is a potent, marketed antifungal agent containing quinazoline moiety (Figure 2).37
Several studies indicated that the presence of arylidenhydrazinyl moiety and pharmacophoric moieties like NO2, Br and Cl possessed potent, marked antimicrobial activities.36,38-40 Molecular hybridization, through comprising two bioactive pharmacophoric sub-units in one molecular union is a strategy of rational design for better therapeutic results.41 Encouraged by the above findings and interested in discovering more quinazoline hybrids for biological evaluation, we focused on the synthesis of a new series of 6,7-dimethoxyquinazolines with unsubstituted quinazoline N-3 bearing different pharmacophoric moieties at the 4th position. Especially, we aimed to introduce biologically significant pharmacophoric moieties which are frequently found in potent antitumor and antimicrobial agents [piperazine, piperidine, arylidenehydrazinyl or thiosemicarbazide]. The anticancer activity of four of the synthesized quinazolines was evaluated by National Cancer Institute (USA). Compounds 3, 4a, 4b and 7 were screened against a panel of 59 human tumor cell lines. The antimicrobial activity of all the newly synthesized quinazolines was tested against Gram positive bacteria (Staphylococcus aureus and Bacillus subtilis), Gram negative bacteria (Escherichia coli and Klebsiella) and one fungal strain (Candida albicans).
The synthetic routes to the target compounds are outlined in Schemes 1 and 2. The starting material methyl 2-amino-4,5-dimethoxybenzoate was refluxed with formamide for 3 h to afford 6,7-dimethoxyquinazolin-4(3H)-one (1). The corresponding 4-chloro derivative 2 was obtained upon refluxing with phosphorus oxychloride for 8 h according to the previously reported methods with certain modifications.42 Compound 2 is considered as the corner stone for the preparation of finals in Scheme 1. Reacting compound 2 with 2-(4-aminopiperazin-1-yl)ethanol gave the 4-substitued piperazinoquinazoline 3. The formation of 3 was proven through versatile spectroscopic tools. The IR spectrum revealed the appearance of two absorption bands at 3309 and 3200 cm-1 corresponding to OH and NH groups. Further evidence was obtained from 1H NMR spectrum which showed two D2O-exchangeable signals at δ 5.25 and 10.60 ppm corresponding to NH and OH protons as well as the signals corresponding to the piperazine aliphatic protons. The 4-substituted amino-6,7-dimethoxyquinazolines 4a,b were obtained through reacting the chloro derivative 2 with ethylpiperazine or benzylpiperidine, respectively. Furthermore, refluxing the 4-chloro derivative 2 with hydrazine hydrate in ethanol afforded the 4-hydrazinyl-6,7-dimethoxyquinazoline (5).30 Scheme 2 outlines the different reactions of 5.
Series 6 was prepared by reacting the 4-hydrazinyl derivative 5 with the selected aromatic aldehydes in dioxane. The 1H NMR spectra showed the presence of one exchangeable signal at δ 12.07- 13.47 ppm corresponding to NH proton, in addition to the characteristic signals of phenyl or substituted phenyl groups. An attempt to synthesize 6,7-dimethoxyquinazolin-4-yl hydrazinecarboxamides A via reacting the hydrazinyl derivative 5 with certain aromatic isocyanates was unsuccessful regardless to the isocyanate used. The formation of this unexpected product was proven through different tools. The 1H NMR spectrum of 7 revealed the absence of any extra aromatic protons other than those of quinazoline moiety. In addition, one exchangeable signal at δ 12.03 ppm corresponding to only one NH proton was another strong evidence. The IR spectrum showed the absorption band at 1651 cm-1 corresponding to the cyclic amidic C=O group. Moreover, the 13C NMR spectrum displayed the presence of seven types of aromatic carbons at the range of δ 105.38-154.93 ppm, in addition to the peak at δ 160.53 ppm indicating the presence of C=O group. The mass spectrum of 7 showed a molecular ion peak at m/z 246. A possible mechanism for the formation of 7 from reacting the 4-hydrazinylquinazoline 5 with the aromatic isocyanates was proposed as follows: The carbon atom of the carbonyl group has a high electrophilic character so facilitates the nucleophilic attack by quinazoline N-3 with the elimination of Ar1NH2 group and formation of the unique triazoloquinazoline 7 (Scheme 3).
Finally, the 4-hydrazinyl quinazoline 5 was reacted with the appropriate aromatic isothiocyanate in ethanol to give the 6,7-dimethoxyquinazolin-4-yl hydrazinecarbothioamides 8a-d. The carbon of the thiocarbonyl group has lower electrophilic character than that of the carbonyl group so the N-3 of quinazoline did not attack the thiocarbonyl group affording compounds 8a-d. The IR spectra indicated the presence of three absorption bands at the range 3367-3120 cm-1 corresponding to three NH groups. In addition, C=S group appeared as an absorption band at the range 1251-1228 cm-1. On the other hand, the 1H NMR spectra displayed three exchangeable signals at δ 8.50 - 12.02 ppm assigned to NH protons.
Antitumor activity
In this study, 4 newly synthesized compounds 3, 4a, 4b and 7 were selected by National Cancer Institute (USA) for anticancer evaluation. These compounds were evaluated at a single dose (10-5 M) utilizing 59 different human tumor cell lines representing leukemia, melanoma, and cancers of lung, colon, central nervous system (CNS), ovary,kidney, prostate as well as breast. The growth inhibition percentages obtained from the single dose test for compounds 3, 4a, 4b and 7 are shown in Table 1.
The results revealed that the test compounds showed mild activity against certain tumor cell lines. Interestingly, renal cancer UO-31 cell line proved to be sensitive to compounds 3, 4a, 4b and 7 with growth inhibition percentages 24.56, 10.58, 15.05 and 14.60 respectively. Compound 3 showed growth inhibition percentage 16.77 against T-47D cell line belonging to breast cancer. Compound 4b exhibited growth inhibition percentage 10.42 against SR cell line representing leukemia. Additionally, compounds 4b and 7 demonstrated activity against SNB-75 cell line belonging to CNS cancer with growth inhibition percentages 21.52 and 20.06, respectively. The antitumor activity correlation of the test dimethoxyquinazolines showed that compounds 3 and 4a with 4-piperazinyl moiety showed activity against renal cancer UO-31 cell line and T-47D cell line belonging to breast cancer. Compound 3 with additional NH and OH groups exhibited improved anticancer activity. Replacement of piperazine moiety with benzylpiperidine in compound 4b kept the activity against renal cancer UO-31 cell line and improved the anticancer activity against SR cell line representing leukemia and SNB-75 cell line belonging to CNS cancer. Regarding the 8,9-dimethoxytriazoloquinazoline 7, it was found that this compound demonstrated activity against renal cancer UO-31 cell line and also, showed activity against CNS cancer SNB-75 cell line.
Antimicrobial activity
The antimicrobial activity of the newly synthesized quinazolines was tested against bacterial and fungal strains: Gram positive (Staphylococcus aureus and Bacillus subtilis), Gram-negative (Escherichia coli and Klebsiella) and one fungal strain (Candida albicans) at Microbiology and Immunology Department, Faculty of Pharmacy, Cairo University. The testing of the antibacterial and antifungal activity of the new quinazolines was investigated by qualitative screening of the susceptibility spectrum of different microbial strains to the test compounds by Kirby-Bauer disk diffusion method. Interpretation for susceptible (sensitive) and non-susceptible (intermediate and resistant) responses were in accordance with the criteria of the Clinical and Laboratory Standards Institute. The obtained results are represented in Table 2.
It was observed that among the test quinazolines, compounds 6a, 6b and 8d showed the most potent, marked activity against Candida albicans. Also, compound 4b demonstrated good activity against Candida albicans. Compounds 7 and 8c showed moderate antibacterial activity against Escherichia coli and Bacillus subtilis, respectively. The test quinazolines exerted no antimicrobial activity against Staphylococcus aureus or Klebsiella. Furthermore, the quantitative assay of the antimicrobial activity was performed for the active compounds by nutrient broth serial method in order to establish the minimal inhibitory concentration (MIC), results are shown in Table 3.
It was found that quinazolines 6a, 6b and 8d with MIC of 83.5 μg/mL against Candida albicans exhibited 6 folds more potent antifungal activity than griseofulvin (MIC: 500 μg/mL). Also, quinazoline 4b showed MIC of 600 μg/mL against Candida albicans representing 83.33% of griseofulvin activity.
The triazoloquinazoline 7 and compound 8c showed moderate antibacterial activity against Escherichia coli and Bacillus subtilis, respectively with MIC of 167 μg/mL. The antimicrobial activity correlation of the test dimethoxyquinazolines showed that the piperazinyl derivatives 3 and 4a without phenyl ring in the moiety at C-4 position were inactive. Introduction of phenyl ring in compound 4b improved the antifungal activity. Among the most potent antifungal compounds were 6a and 6b with the active moieties (benzylidenehydrazinyl and 2-nitrobenzylidenehydrazinyl), while introduction of the electron rich 4-methoxyphenyl group in quinazoline 6c resulted in loss of the antifungal activity. The triazoloquinazoline 7 showed moderate antibacterial activity. Among the hydrazinecarbothioamide derivatives, 8d with the electron donating 4-MeO group exerted excellent antifungal activity, while other derivatives with unsubstituted phenyl or phenyl ring substituted with electron withdrawing groups (Br or Cl) showed no antifungal activity. Compound 8c with 4-Cl phenyl group showed moderate antibacterial activity. Overall results revealed that:
a- 6,7-Dimethoxyquinazoline is a satisfactory backbone for antifungal activity.
b- The presence of a moiety containing both NH and phenyl groups at C-4 position (such as the arylidenehydrazinyl or phenylthiosemicarbazide) is essential for the antifungal activity.
Conclusion
The present work led to the development of a series of novel of 6,7-dimethoxyquinazoline derivatives containing different moieties at C-4 position and one 8,9-dimethoxytriazoloquinazoline. Compounds 3, 4a, 4b and 7 was evaluated by National Cancer Institute (USA) at single dose (10-5 M). It was found that the test compounds showed mild activity against certain tumor cell lines. Interestingly, renal cancer UO-31 cell line proved to be sensitive to these compounds. Additionally, compounds 4b and 7 demonstrated activity against SNB-75 cell line belonging to CNS cancer. The antimicrobial activity of the newly synthesized quinazolines was tested against bacterial and fungal strains. Compounds 6a, 6b and 8d showed the most potent, marked activity against Candida albicans with MIC 83.5 μg/mL representing 6 folds more potent activity than griseofulvin. Also, quinazoline 4b showed MIC of 600 μg/mL against Candida albicans representing 83.33% of griseofulvin activity. Compounds 7 and 8c showed moderate antibacterial activity against Escherichia coli and Bacillus subtilis, respectively. These quinazoline hybrids could be considered as useful templates for further development to obtain more potent antifungal agents.
EXPERIMENTAL
Chemistry
Melting points were obtained on a Griffin apparatus and were uncorrected. Microanalyses for C, H and N were carried out using Heraew and Vario El III (elemntar), CHNS analyzer (Germany) at the Microanalytical Center, Al-Azhar University. IR spectra were recorded on a Shimadzu 435 spectrometer, using KBr discs. 1H NMR were performed on joel NMR FXQ-300 MHz and 13C NMR spectra were performed on joel NMR FXQ-400 MHz spectrometers, using TMS as the internal standard. Mass spectra were recorded on a GCMP-QP1000 EX Mass spectrometer utilizing the electron ionization (EI) method. Progress of the reactions were monitored by TLC using precoated aluminum sheet silica gel MERCK 60F 254 and was visualized by UV lamp.
6,7-Dimethoxyquinazolin-4(3H)-one (1) 42
A suspension of the methyl 2-amino-4,5-dimethoxybenzoate (2.11 g, 0.01mol) in formamide (15 mL) was heated under reflux for 3 h, then allowed to cool to room temperature. The separated solid was filtered and dried. mp 292-294 oC; yield 83%; IR (KBr) vmax: 3143 (NH), 3051 (C-H aromatic), 2931 (C-H aliphatic), 1674 (C=O) cm-1; 1H NMR (300 MHz, DMSO-d6): δ 3.87 (s, 3H, CH3O), 3.97 (s, 3H, CH3O), 7.13 (s,1H, ArH), 7.44 (s,1H, ArH) and 7.99 (s,1H, C2-H) and 12.07 (s, 1H, NH, D2O exchangeable) ppm.
4-Chloro-6,7-dimethoxyquinazoline (2) 42
A mixture of phosphorus oxychloride (15 mL), the quinazolin-4(3H)-one 1 (2.06 g, 0.01mol) and 1 mL N,N-dimethylaninline was heated under reflux for 8 h, then concentrated to dryness, the oily residue was poured into ice cold water (100 mL). The formed solid was filtered, dried and crystallized from EtOH. mp 182-184 oC; yield 75%; IR (KBr) vmax: 3048 (C-H aromatic), 2933 (C-H aliphatic), 1618 (C=N) cm-1; 1H NMR (300 MHz, DMSO-d6): δ 3.85 (s, 3H, CH3O), 3.89 (s, 3H, CH3O), 7.13 (s, 1H, ArH), 7.43 (s, 1H, ArH) and 8.14 (s,1H, C2-H) ppm.
2-(4-(6,7-Dimethoxyquinazolin-4-ylamino)piperazin-1-yl)ethanol (3)
A mixture of the chloro derivative 2 (0.2 g, 0.001 mol), 2-(4-aminopiperazin-1-yl)ethanol (0.15 g, 0.001 mol) and triethylamine (0.36 mL) in absolute EtOH (12 mL) was heated under reflux for 15 h. The reaction mixture was then cooled, the separated solid was filtered, dried and crystallized from EtOH. mp 244-246 oC; yield 41%; IR (KBr) vmax: 3309,3200 (NH and OH), 3014 (C-H aromatic), 2954 (C-H aliphatic)cm-1; 1H NMR (300 MHz, DMSO-d6): δ 2.90.2.95 (m, 2H, CH2), 3.05-3.15 (m, 4H, 2CH2), 3.20-3.25 (m, 2H, CH2), 3.45-3.51 (m, 2H, NCH2CH2OH), 3.64-3.67 (m, 2H, NCH2CH2OH), 3.75 (s, 3H, CH3O), 3.83 (s, 3H, CH3O), 5.25 (s, 1H, NH, D2O exchangeable), 7.15 (s,1H, ArH), 7.39 (s,1H, ArH), 8.65 (s,1H, C2-H) and 10.60 (brs, 1H, OH, D2O exchangeable) ppm; 13C NMR (100 MHz, DMSO-d6): δ 40.78, 55.78, 56.15, 56.39, 57.11, 105.39, 108.48, 112.80, 145.34, 149.01, 149.09, 154.91, 160.51 ppm; MS [m/z, %]: 334 [M+, 0.68]. Anal. Calcd for C16H23N5O3 (333.39): C, 57.64; H, 6.95; N, 21.01. Found: C, 57.79; H, 6.98; N, 21.17.
General procedure for the preparation of 4-substitutedamino-6,7-dimethoxyquinazolines (4a,b)
A mixture of the chloro derivative 2 (0.2 g, 0.001 mol), ethylpiperazine or benzylpiperidine (0.001 mol) and triethylamine (0.36 mL) in absolute EtOH (12 mL) was heated under reflux for 15 h. The reaction mixture was cooled and the separated solid was filtered, dried and crystallized from EtOH.
4-(4-Ethylpiperazin-1-yl)-6,7-dimethoxyquinazoline (4a)
mp 245-247 oC; yield 66%; IR (KBr) vmax: 3093 (C-H aromatic), 2920 (C-H aliphatic), 1612 (C=N) cm-1; 1H NMR (300 MHz, DMSO-d6): δ 1.23- 1.27 (t, 3H, J= 6.9 Hz, CH2CH3), 2.80- 2.83 (m, 4H, 2CH2), 3.00-3.05 (q, 2H, J= 6.9 Hz, CH2CH3), 3.20-3.40 (m, 4H, 2CH2), 3.93 (s, 3H, CH3O), 3.94 (s, 3H, CH3O), 7.15 (s,1H, ArH), 7.26 (s,1H, ArH) and 8.61 (s,1H, C2-H) ppm; 13C NMR (100 MHz, DMSO-d6): δ 11.01, 40.78, 52.10, 53.83, 56.20, 56.32, 107.51, 111.05, 112.80, 129.76, 133.54, 149.09, 166.31, 177.05 ppm; MS [m/z, %]: 302 [M+, 3.99]. Anal. Calcd for C16H22N4O2 (302.37): C, 63.55; H, 7.33; N, 18.53. Found: C, 63.72; H, 7.42; N, 18.81.
4-(4-Benzylpiperidin-1-yl)-6,7-dimethoxyquinazoline (4b) mp 180-182 oC; yield 50%; IR (KBr) vmax: 3024 (C-H aromatic), 2920 (C-H aliphatic), 1616 (C=N) cm-1; 1H NMR (300 MHz, DMSO-d6): δ 1.37-1.48 (m, 1H, CH piperidine), 1.71-1.84 (m, 4H, 2CH2), 2.59 (d, 2H, C6H5CH2), 2.83-2.99 (m, 4H, 2CH2), 3.89 (s, 3H, CH3O), 3.92 (s, 3H, CH3O), 7.01 (s,1H, ArH), 7.19-7.32 (m, 6H, ArH) and 8.50 (s,1H, C2-H) ppm; 13C NMR (100 MHz, DMSO-d6): δ 32.07, 38.21, 42.76, 50.02, 56.01, 56.30, 103.48, 107.61, 112.80, 126.29, 128.66, 129.14, 140.67, 148.41, 148.94, 152.94, 154.56, 163.66 ppm; MS [m/z, %]: 363 [M+, 73.72]. Anal. Calcd for C22H25N3O2 (363.45): C, 72.70; H, 6.93; N, 11.56. Found: C, 72.94; H, 7.08; N, 11.69.
4-Hydrazinyl-6,7-dimethoxyquinazoline (5) 30
A mixture of the chloro derivative (0.002 mol) and hydrazine hydrate (99%, 0.62 g, 0.012 mol) in absolute EtOH (20 mL) was refluxed for 6 h. The reaction mixture was then cooled and the precipitate was filtered, dried and crystallized from EtOH. mp 202-204 oC; yield 62%; IR (KBr) vmax: 3342, 3294, 3167 (NH /NH2), 3055 (C-H aromatic), 2960 (C-H aliphatic) , 1618 (C=N) cm-1.
General procedure for the preparation of 4-(2-benzylidenehydrazinyl)-6,7-dimethoxyquinazolines (6a-c)
A mixture of the hydrazinyl derivative 5 (0.003 mol) and the selected aromatic aldehyde (0.003 mol) in dioxane (17 mL) was heated under reflux for 6 h. The reaction mixture was cooled, the separated solid was filtered, dried and crystallized from EtOH.
4-(2-Benzylidenehydrazinyl)-6,7-dimethoxyquinazoline (6a)
mp 240-242 oC; yield 90%; IR (KBr) vmax: 3057 (C-H aromatic), 2933 (C-H aliphatic), 1616 (C=N) cm-1; 1H NMR (300 MHz, DMSO-d6): δ 3.94 (s, 3H, CH3O), 3.95 (s, 3H, CH3O), 7.12 (s,1H, ArH), 7.34 (s,1H, ArH), 7.34-7.53 (m, 3H, ArH), 7.92-7.95 (m, 2H, ArH), 7.98 (s,1H, C2-H), 8.68 (s, 1H, CH=N) and 12.10 (s, 1H, NH, D2O exchangeable) ppm; 13C NMR (100 MHz, DMSO-d6): δ 56.16, 56.39, 105.39, 108.39, 114.86, 116.04, 127.02, 129.82, 130.44, 144.34, 145.21, 149.03, 154.93, 160.94, 162.14 ppm; MS [m/z, %]: 308 [M+, 56.19]. Anal. Calcd for C17H16N4O2 (308.33): C, 66.22; H, 5.23; N, 18.17. Found: C, 66.38; H, 5.30; N, 18.43.
6,7-Dimethoxy-4-(2-(2-nitrobenzylidene)hydrazinyl)quinazoline (6b) mp > 300 oC; yield 78%; IR (KBr) vmax: 3080 (C-H aromatic), 2924 (C-H aliphatic), 1618 (C=N) cm-1; 1H NMR (300 MHz, DMSO-d6): δ 3.89 (s, 3H, CH3O), 3.91 (s, 3H, CH3O), 7.03 (s,1H, ArH), 7.27 (s,1H, ArH), 7.61-7.76 (m, 4H, ArH), 8.18 (s,1H, C2-H), 8.60 (s, 1H, CH=N) and 13.47 (s, 1H, NH, D2O exchangeable) ppm; 13C NMR (100 MHz, DMSO-d6): δ 56.31, 56.40, 103.13, 108.12, 110.86, 125.25, 128.29, 129.90, 132.63, 134.39, 143.79, 147.76, 148.77, 150.57, 155.27, 159.12, 160.75 ppm; MS [m/z, %]: 353 [M+, 1.85]. Anal. Calcd for C17H15N5O4 (353.33): C, 57.79; H, 4.28; N, 19.82. Found: C, 57.94; H, 4.33; N, 19.97.
6,7-Dimethoxy-4-(2-(4-methoxybenzylidene)hydrazinyl)quinazoline (6c) mp 229-231 oC; yield 88%; IR (KBr) vmax: 3055 (C-H aromatic), 2935(C-H aliphatic), 1606 (C=N) cm-1; 1H NMR (300 MHz, DMSO-d6): δ 3.90 (s, 3H, CH3O), 3.97 (s, 3H, CH3O), 4.03 (s, 3H, CH3O), 7.05 (d, 1H, J= 9.0 Hz, ArH), 7.10 (d, 1H, J= 9.0 Hz, ArH), 7.12 (s, 1H, ArH), 7.44 (s, 1H, ArH), 7.80 (d, 1H, J= 9.0 Hz, ArH), 7.90 (d, 1H, J= 9.0 Hz, ArH), 7.99 (s, 1H, C2-H), 8.69 (s, 1H, CH=N) and 12.07 (s, 1H, NH, D2O exchangeable) ppm; 13C NMR (100 MHz, DMSO-d6): δ 55.85, 56.15, 56.39, 105.39, 108.41, 113.26, 114.86, 127.04, 130.44, 144.34, 149.02, 154.92, 160.51, 160.95, 162.00, 162.14 ppm; MS [m/z, %]: 338 [M+, 40.74]. Anal. Calcd for C18H18N4O3 (338.36): C, 63.89; H, 5.36; N, 16.56. Found: C, 64.01; H, 5.44; N, 16.74.
8,9-Dimethoxy-[1,2,4]triazolo[4,3-c]quinazolin-3(2H)-one (7)
A mixture of hydrazinyl derivative 5 (0.001 mol) and the appropriate isocyanate (0.001 mol) in absolute EtOH (5 mL) was heated under reflux for 6 h. After cooling, the separated solid was filtered, dried and crystallized from EtOH. mp 253-255 oC ; average yield 86%; IR (KBr) vmax: 3160 (NH), 3014 (C-H aromatic), 2935 (C-H aliphatic) , 1651 (C=O), 1610 (C=N) cm-1; 1H NMR (300 MHz, DMSO-d6): δ 3.87 (s, 3H, CH3O), 3.90 (s, 3H, CH3O), 7.11 (s, 1H, ArH), 7.42 (s, 1H, ArH), 7.96 (s, 1H, ArH) and 12.03 (s, 1H, NH, D2O exchangeable) ppm; 13C NMR (100 MHz, DMSO-d6): δ 56.16, 56.39, 105.38, 108.47, 116.06, 144.29, 145.33, 149.02, 154.93, 160.53 ppm; MS [m/z, %]: 246 [M+, 1.62], 205 [C10H11N3O2, 100]. Anal. Calcd for C11H10N4O3 (246.22): C, 53.66; H, 4.09; N, 22.75. Found: C, 53.78; H, 4.16; N, 22.74.
General procedure for the preparation of (6,7-dimethoxyquinazolin-4-yl)hydrazinecarbothioamides (8a-d)
A mixture of hydrazinyl derivative 5 (0.001 mol) and the appropriate isothiocyanate (0.001 mol) in absolute EtOH (5 mL) was heated under reflux for 6 h. After cooling, the separated solid was filtered, dried and crystallized from EtOH.
2-(6,7-Dimethoxyquinazolin-4-yl)-N-phenylhydrazinecarbothioamide (8a) mp 228-230 oC; yield 75%; IR (KBr) vmax: 3367, 3205, 3188 (3 NH), 3051 (C-H aromatic), 2939 (C-H aliphatic), 1616 (C=N), 1232 (C=S) cm-1; 1H NMR (300 MHz, DMSO-d6): δ 3.89 (s, 3H, CH3O), 3.98 (s, 3H, CH3O), 7.12 (s, 1H, ArH), 7.28-7.30 (m, 3H, ArH), 7.43 (s, 1H, ArH), 7.53-7.75 (m, 2H, ArH), 8.07 (s, 1H, C2-H), 9.44 (s, 1H, NH, D2O exchangeable), 9.85 (s, 1H, NH, D2O exchangeable) and 12.08 (s, 1H, NH, D2O exchangeable) ppm; 13C NMR (100 MHz, DMSO-d6): δ 56.14, 56.30, 100.57, 108.43, 112.78, 128.86, 129.41, 129.75, 141.68, 147.29, 150.60, 154.97, 159.38, 160.76, 173.75 ppm; MS [m/z, %]: 355 [M+, 67.21]. Anal. Calcd for C17H17N5O2S (355.41): C, 57.45; H, 4.82; N, 19.70. Found: C, 57.62; H, 4.89; N, 19.84.
N-(4-Bromophenyl)-2-(6,7-dimethoxyquinazolin-4-yl)hydrazinecarbothioamide (8b) mp 240-242 oC; yield 75%; IR (KBr) vmax: 3361, 3197, 3138 (3 NH), 3053 (C-H aromatic), 2933 (C-H aliphatic) ,1616 (C=N), 1232 (C=S) cm-1; 1H NMR (300 MHz, DMSO-d6): δ 3.86 (s, 3H, CH3O), 3.90 (s, 3H, CH3O), 7.12 (s, 1H, ArH), 7.44 (s, 1H, ArH), 7.56 (d, 2H, J= 9.0 Hz, ArH), 7.61 (d, 2H, J= 9.0 Hz, ArH), 7.96 (s, 1H, C2-H), 8.50 (s, 1H, NH, D2O exchangeable), 9.45 (s, 1H, NH, D2O exchangeable) and 11.99 (s, 1H, NH, D2O exchangeable) ppm; 13C NMR (100 MHz, DMSO-d6): δ 56.14, 56.29, 100.53, 108.01, 112.79, 124.33, 129.75, 132.00, 133.49, 142.00, 147.61, 154.89, 160.51, 160.72, 173.36 ppm; MS [m/z, %]: 435 [(M+2)+, 3.45], 433 [M+, 4.73]. Anal. Calcd for C17H16BrN5O2S (434.31): C, 47.01; H, 3.71; N, 16.13. Found: C, 47.13; H, 3.68; N, 16.41.
N-(4-Chlorophenyl)-2-(6,7-dimethoxyquinazolin-4-yl)hydrazinecarbothioamide (8c) mp 240-242 oC; yield 79%; IR (KBr) vmax: 3367, 3160, 3140 (3 NH), 3040 (C-H aromatic), 2935 (C-H aliphatic) ,1614 (C=N), 1228 (C=S) cm-1; 1H NMR (300 MHz, DMSO-d6): δ 3.89 (s, 3H, CH3O), 3.97 (s, 3H, CH3O), 7.10 (s, 1H, ArH), 7.42 (s, 1H, ArH), 7.67 (d, 2H, J= 9.0 Hz, ArH), 7.83 (d, 2H, J= 9.0 Hz, ArH), 7.95 (s, 1H, C2-H), 9.42 (s, 1H, NH, D2O exchangeable) and 12.02 (s, 1H, NH, D2O exchangeable) ppm; 13C NMR (100 MHz, DMSO-d6): δ 56.14, 56.38, 100.54, 105.57, 109.54, 116.02, 124.34, 131.99, 134.20, 144.28, 148.99, 150.65, 155.09, 160.52, 173.35 ppm; MS [m/z, %]: 391 [(M+2)+, 0.09], 389 [M+, 0.1]. Anal. Calcd for C17H16ClN5O2S (389.86): C, 52.37; H, 4.14; N, 17.96. Found: C, 52.52; H, 4.20; N, 18.08.
2-(6,7-Dimethoxyquinazolin-4-yl)-N-(4-methoxyphenyl)hydrazine-carbothioamide (8d) mp 224-226 oC; yield 72%; IR (KBr) vmax: 3213, 3134, 3120 (3 NH), 3043 (C-H aromatic), 2960 (C-H aliphatic) , 1616 (C=N), 1251 (C=S) cm-1; 1H NMR (300 MHz, DMSO-d6): δ 3.77 (s, 3H, CH3O), 3.86 (s, 3H, CH3O), 3.88 (s, 3H, CH3O), 7.12 (s, 1H, ArH), 7.29 (d, 2H, J= 9.0 Hz, ArH), 7.43 (s, 1H, ArH), 7.52 (d, 2H, J= 9.0 Hz, ArH), 7.98 (s, 1H, C2-H), 9.41 (s, 1H, NH, D2O exchangeable), 9.46 (s, 1H, NH, D2O exchangeable) and 12.07 (s, 1H, NH, D2O exchangeable) ppm; 13C NMR (100 MHz, DMSO-d6): δ 55.78, 56.14, 56.30, 100.66, 108.44, 112.79, 114.07, 127.24, 130.94, 145.31, 150.52, 154.94, 156.92, 160.53, 161.04, 173.98 ppm; MS [m/z, %]: 385 [M+, 3.58]. Anal. Calcd for C18H19N5O3S (385.44): C, 56.09; H, 4.97; N, 18.17. Found: C, 56.23; H, 5.05; N, 18.42.
Measurement of anticancer activity
Anticancer activity screening of the newly synthesized compounds was measured in vitro utilizing 59 different human tumor cell lines provided by US National Cancer Institute according to previously reported standard procedure43-45 as follows:
Cells are inoculated into 96 well microtiter plates in 100 µL. After cell inoculation, the microtiter plates are incubated at 37 °C, 5% CO2, 95% air and 100% relative humidity for 24 h prior to addition of experimental drugs.
After 24 h, two plates of each cell line are fixed in situ with TCA, to represent a measurement of the cell population for each cell line at the time of drug addition (Tz). Experimental drugs are solubilized in dimethyl sulfoxide at 400-fold the desired final maximum test concentration and stored frozen prior to use. At the time of drug addition, an aliquot of frozen concentrate is thawed and diluted to twice the desired final maximum test concentration with complete medium containing 50 µg/mL gentamicin. Additional four, 10-fold or ½ log serial dilutions are made to provide a total of five drug concentrations plus control. Aliquots of 100 µL of these different drug dilutions are added to the appropriate microtiter wells already containing 100 µL of medium, resulting in the required final drug concentrations.
Following drug addition, the plates are incubated for an additional 48 h at 37 °C, 5% CO2, 95% air, and 100% relative humidity. For adherent cells, the assay is terminated by the addition of cold TCA. Cells are fixed in situ by the gentle addition of 50 µL of cold 50% (w/v) TCA (final concentration, 10% TCA) and incubated for 60 min at 4 °C. The supernatant is discarded, and the plates are washed five times with tap water and air dried. Sulforhodamine B (SRB) solution (100 µL) at 0.4% (w/v) in 1% acetic acid is added to each well, and plates are incubated for 10 min at room temperature. After staining, unbound dye is removed by washing five times with 1% acetic acid and the plates are air dried. Bound stain is subsequently solubilized with 10 mM trizma base, and the absorbance is read on an automated plate reader at a wavelength of 515 nm. For suspension cells, the methodology is the same except that the assay is terminated by fixing settled cells at the bottom of the wells by gently adding 50 µL of 80% TCA (final concentration, 16%TCA). Using the seven absorbance measurements [time zero, (Tz), control growth, (C), and test growth in the presence of drug at the five concentration levels (Ti)], the percentage growth is calculated at each of the drug concentrations levels. Percentage growth inhibition is calculated as:
[(Ti-Tz)/(C-Tz)] x 100 for concentrations for which Ti>/=Tz
[(Ti-Tz)/Tz] x 100 for concentrations for which Ti<Tz.
Qualitative screening of the antimicrobial properties of the tested compounds
Antibacterial activity of the test compounds was determined using a modified Kirbye Bauer disc diffusion method.46 In our experiment, there were used microbial suspensions of 1.5x108 UFC/ mL or equivalent to 0.5 McFarland density obtained from 15-18 h microbial cultures developed on solid media. For the disk diffusion method, sterile cotton swabs were used to transfer the inoculum onto Iso sensi-test agar and Sabourad dextrose agar plates for bacterial and Candida isolates respectively, to produce a confluent lawn of bacterial and fungal growth. After the inoculum on the plates was dried, disks were distributed over the inoculated plates. The test compounds were solubilized in DMSO (1 mg/mL). Ten μL of the compound solution were equally distributed on the paper filter disks then placed on Petri dishes previously seeded. These plates were then incubated at 37 °C for 24 h for under aerobic conditions.
Zone diameters were measured in millimeters from the edge of the disc to the edge of the zone. Standard antibacterial vancomycin for Gram +ve bacteria and ampicillin for Gram –ve bacteria and antifungal griseofulvin were also screened under similar condition for comparison.
Quantitative assay of the antimicrobial activity
The quantitative assay of the antimicrobial activity was performed by nutrient broth serial method47 in order to establish the minimal inhibitory concentration (MIC). For the quantitative assay of the antimicrobial activity of the new compounds by the broth serial dilution method in liquid medium distributed in wasser man tubes, serial dilutions of the test compounds solutions were performed. Double strength nutrient broth and Sabourad dextrose broth were used for bacterial and Candida isolates respectively. There were obtained concentrations from 1000 μg/mL to 0.48 μg/mL in a 1mL culture medium final volume, afterwards each tube was seeded with a 0.5mL microbial suspension of 0.5 MacFarland density. In each test a microbial culture control (a tube containing exclusively culture medium with the microbial suspension) and a sterility control (a tube containing exclusively culture medium) were performed. The tubes were incubated for 24 h at 37 °C. For the quantitative methods of the antimicrobial activity of the test compounds by the serial dilution method in liquid medium, the MIC was read by tubes observation. The lowest concentration which inhibited the visible microbial growth was considered the MIC (μg/mL) value for the test compound. In the microbial culture control tubes, the tubes had to be turbid and in the sterility control tubes, the medium had to remain clear.
ACKNOWLEDGMENT
We are grateful to all members of National Institute of Health, Meryland, USA for carrying out the anticancer screening.
References
1. S. T. Al-Rashood, I. A. Aboldahab, M. N. Nagi, L. A. Abouzeid, A. A. M. Abdel-Aziz, S. G. Abdel-hamide, K. M. Youssef, A. M. Al-Obaid, and H. I. El-Subbagh, Bioorg. Med. Chem., 2006, 14, 8608. CrossRef
2. N. M. Abdel Gawad, H. H. Georgey, R. M. Youssef, and N. El-Sayed, Eur. J. Med. Chem., 2010, 45, 6058. CrossRef
3. E. M. Ahmed, N. M. Taha, S. M. A. El-gawad, and N. M. S. Nady, Der Chem. Sin., 2011, 2, 197.
4. M. T. Conconi, G. Marzaro, L. Urbani, I. Zanusso, R. Di Liddo, I. Castagliuolo, P. Brun, F. Tonus, A. Ferrarese, A. Guiotto, and A. Chilin, Eur. J. Med. Chem., 2013, 67, 373. CrossRef
5. I. A. Al-Suwaidan, A. M. Alanazi, A. A. M. Abdel-Aziz, M. A. Mohamed, and A. S. El-Azab, Bioorg. Med. Chem. Lett., 2013, 23, 3935. CrossRef
6. T. V. T. Le, J. H. Suh, N. Kim, and H. J. Park, Bioorg. Med. Chem. Lett., 2013, 23, 2642. CrossRef
7. L. Xu and W. A. Russu, Bioorg. Med. Chem., 2013, 21, 540. CrossRef
8. Y.-Y. Xu, S.-N. Li, G.-J. Yu, Q.-H. Hu, and H.-Q. Li, Bioorg. Med. Chem., 2013, 21, 6084. CrossRef
9. X. Zhang, T. Peng, X. Ji, J. Li, L. Tong, Z. Li, W. Yang, W. Xu, M. Li, J. Ding, H. Jiang, H. Xie, and H. Liu, Bioorg. Med. Chem., 2013, 21, 7988. CrossRef
10. Y. Zhang, Y. J. Huang, H. M. Xiang, P. Y. Wang, D. Y. Hu, W. Xue, B. A. Song, and S. Yang, Eur. J. Med. Chem., 2014, 78, 23. CrossRef
11. M. L. D. C. Barbosa, L. M. Lima, R. Tesch, C. M. R. Sant’Anna, F. Totzke, M. H. G. Kubbutat, C. Schächtele, S. A. Laufer, and E. J. Barreiro, Eur. J. Med. Chem., 2014, 71, 1.
12. K. Paul, A. Sharma, and V. Luxami, Bioorg. Med. Chem. Lett., 2014, 24, 624. CrossRef
13. N. Raghav and M. Singh, Eur. J. Pharm. Sci., 2014, 54, 28. CrossRef
14. A. A. Shalaby, A. A. El-Khamry, S. A. Shiba, A. A. A. Ahmed, and A. A. Hanafi, Arch. Pharm. Pharm. Med. Chem., 2000, 333, 365. CrossRef
15. G. F. Xu, B. A. Song, P. S. Bhadury, S. Yang, P. Q. Zhang, L. H. Jin, W. Xu, D. Y. Hu, and P. Lu, Bioorg. Med. Chem., 2007, 15, 3768. CrossRef
16. A. K. Nanda, S. Ganguli, and R. Chakraborty, Molecules, 2007, 12, 2413. CrossRef
17. M. Minu, A. Thangadurai, S. R. Wakode, S. S. Agrawal, and B. Narasimhan, Arch. Pharm., 2008, 341, 231. CrossRef
18. S. Boyapati, U. Kulandaivelu, S. Sangu, and M.R. Vanga, Arch. Pharm., 2010, 343, 570. CrossRef
19. M. Veerapandian, M. Marimuthu, P. Ilangovan, S. Ganguly, K. S. Yun, S. Kim, and J. An, Med. Chem. Res., 2010, 19, 283. CrossRef
20. T. A. R. Farghaly, M.M. Edrees, and M. A. Mosselhi, Molecules, 2012, 17, 8483. CrossRef
21. R. P. Modh, A.C. Patel, D. H. Mahajan, C. Pannecouque, E. De Clercq, and K. H. Chikhalia, Arch. Pharm., 2012, 345, 964. CrossRef
22. G. Saravanan, V. Alagarsamy, and C. R. Prakash, Med. Chem. Res., 2013, 22, 340. CrossRef
23. P. Parasuraman, R. Srimathi, and A. Dineshraja, Int. J. Pharm. Pharm. Sci., 2013, 5, 273.
24. N. C. Desai, H. V. Vaghani, and P. N. Shihora, J. Fluorine Chem., 2013, 153, 39. CrossRef
25. Q.-G. Ji, D. Yang, Q. Deng, Z.-Q. Ge, and L.-J. Yuan, Med. Chem. Res., 2014, 23, 2169. CrossRef
26. N. S. El-Gohary and M. I. Shaaban, Med. Chem. Res., 2014, 23, 287. CrossRef
27. A. B. Patel, K. H. Chikhalia, and P. Kumari, Med. Chem. Res., 2014, 23, 2338. CrossRef
28. K. H. G. A. E.Wakeling , S. P. Guy, J. R. Woodburn, S. E. Ashton, B. J. Curry, and A. J. Barker, Cancer Res., 2002, 62, 5749.
29. M. C. H. D. J. DeAngelo, R. M. Stone, M. L. Heany, S. D. Nimer, R. L. Paquette, R. B. Klisovic, M. A. Caligiuri, M. R. Cooper, J. Leverf, M. D. Karol, S. Sheng, N. Holford, P. T. Curtin, and B. J. Druker, Blood, 2006, 108, 3674. CrossRef
30. J. He, X. Wang, X. Zhao, Y. Liang, H. He, and L. Fu, Eur. J. Med. Chem., 2012, 54, 925. CrossRef
31. H. Tingjun, Z. Lili, C. Lirong, and X. Xiaojie, Chem. Inf. Comput. Sci., 2003, 43, 273. CrossRef
32. B. D. Palmer, S. Trumpp-Kallmeyer, D. W. Fry, J. M. Nelson, H. D. H. Schwatter, and W. A. Denny, J. Med. Chem., 1997, 40, 1519. CrossRef
33. R. Pignatello, G. Spampinato, V. Sorrenti, L. Vicari, C. Di. Giacomo, A. Vanella, and G. Puglisi, Pharm. Pharmacol. Commun., 1999, 5, 299. CrossRef
34. V. Alagarsamy and P. Parthiban, RASAYAN J. Chem., 2011, 4, 736.
35. D. R. Shah, H. P. Lakum, and K. H. Chikhalia, Russ. J. Bioorg. Chem., 2015, 41, 209. CrossRef
36. P. Panneerselvam, B. A. Rather, D. R. S. Reddy, and N. R. Kumar, Eur. J. Med. Chem., 2009, 44, 2328. CrossRef
37. A. C. Pasqualotto, K. O. Thiele, and L. Z. Goldani, Curr. Opin. Investig. Drugs, 2010, 2, 165.
38. Y. D. Kulkarni, and A. Rowhani, J. Indian Chem. Soc., 1990, 67, 46.
39. P. Mishra, P. N. Gupta, and A. K. Shakya, J. Indian Chem. Soc., 1991, 68, 618.
40. P. Mishra, P. Pannerselvam, and S. Jain, J. Indian Chem. Soc., 1995, 72, 559.
41. C. Viegas-Junior, A. Danuello, B.V. da Silva, E. J. Barreiro, and C. A. Fraga, Curr. Med. Chem., 2007, 14, 1829. CrossRef
42. E. B. Bentecteux, C. Loison, N. Pommery, R. Houssin, and J. P. Henichart, Anticancer Drug Des., 1998, 13, 893.
43. M. C. Alley, D. A. Scudiero, P. A. Monks, M. L. Hursey, M. J., Fine, D. L. Czerwinski, B. J. Abbott, J. G. Mayo, R. H. Shoemaker, and M. R. Boyd, Cancer Res., 1988, 48, 589.
44. M. R. Grever, S. A. Schepartz, and B. A. Chabner, Semin. Oncol., 1992, 19, 622.
45. M. R. Boyd and K. D. Paull, Drug Develop.Res., 1995, 34, 91. CrossRef
46. A. W. Bauer, W. M. Kirby, C. Sherris, and M. Truck, Am. J. Clin. Pathol., 1966, 45, 493.
47. Clinical Laboratory and Standard Institute, Methods for Dilution Antimicrobial Susceptibility Tests for Bacteria That Grow Aerobically; Approved Standard, ninth ed., CLSI, Wayne, PA, 2010