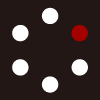
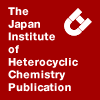
HETEROCYCLES
An International Journal for Reviews and Communications in Heterocyclic ChemistryWeb Edition ISSN: 1881-0942
Published online by The Japan Institute of Heterocyclic Chemistry
e-Journal
Full Text HTML
Received, 20th November, 2015, Accepted, 21st December, 2015, Published online, 14th January, 2016.
■ Mesoionic Tetrazoles – Progress Since 1980
Dietrich Moderhack*
Institute of Medicinal and Pharmaceutical Chemistry, Technical University, Braunschweig, Beethovenstrasse 55, D-38106, Germany
Abstract
This article summarizes the findings made during the past decades with the traditional classes of mesoionic tetrazoles of type (A) and (B); it also deals with the 'abnormal' carbenes derived therefrom. – Emphasis is placed on preparative aspects.INTRODUCTION
Mesoionic tetrazoles and their five-membered analogues (8π systems) belong to the extensive family of conjugated heterocyclic mesomeric betaines (HMBs) which occur in two types, generally known as A and B (Chart 1).1a-c The entire field has been reviewed repeatedly, covering the literature through 1980.1d–f The present report, however, focusses on the tetrazole derivatives as here substantial progress has been made, especially by contributions from the Araki group. It deals not only with a wide variety of type (A) and (B) systems like A1–A5 and B1, B2, B4, B5 (including B1'), but also features the corresponding mesoionic, 'abnormal' carbenes (C) and (D) as well as their metal complexes (C') and (D') – species of recent vintage.2
GENERAL
The main objective of this survey is the preparative chemistry, comprising synthesis and transformations. Scheme 1 gives a preview of the former, showing the general routes to A–D. To draw a full picture, material that has not been covered by the preceding reviewers,1d–f or dealt with too briefly, is considered as well. Selected physical data are provided in Sections (2) and (3). The traditional representation of A and B with a sextet in the ring has been retained, although type (A) mesoions do not show classical aromaticity.1c,g
1) MESOIONIC TETRAZOLES OF TYPE (A)
a) Tetrazolium-5-olates (A1)
Alkali-induced ring transformation of 1,2,3,4-oxatriazolium-5-aminides (1) constitutes the earliest access to tetrazolium-5-olates (A1) [Scheme 2; cf. route (a) in Scheme 1].1d–f Regarding the scope, the reaction works well with substrates having aryl-substituted aminide groups (such as 1b-k),3a–8 but the process failed with alkyl analogues (1a).9a N-Nitroso derivatives (1; R = NO) might be expected to afford the respective 1-nitrosotetrazolium-5-olates (A1; NO for Ar'), but an attempt with 1 (Ar = Ph, R = NO) showed that the compound is thermally unstable: it lost dinitrogen to give the corresponding 1,2,3,4-oxatriazolium-5-olate in high yield.9b Also the parent substrate (1; Ar = Ph, R = H) could not be induced to rearrange (i.e. into 2-phenyl-2H-tetrazol-5-ol), since it undergoes C–O bond breaking (instead of N–O cleavage).9b
Cyclization reactions using arenediazonium chlorides and (arylhydrazono)methanedisulfonate salts (2) or, alternatively, bis(alkyl/arylsulfonyl)methanes (3a,b) are also established entries to olates (A1) (Scheme 3; cf. route (c) in Scheme 1].1d–f The first mode may be viewed as likewise suited for products having different aryl substituents, but in practice ligand exchange occurs.1e In addition to the previously reviewed1d,f studies dealing with A1b,q,s,u,v,y,3a,10 other early work is referred to for completion: it describes the preparation of A1b,l-r,t-x.11, 12 Of noticeable results, the higher yields of A1b,q,u obtained from 3a,b deserve mention.11a A later variant of this procedure utilized the tris(sulfonyl)methane (3c): from the respective 4-substituted benzenediazonium salts, the derivatives (A1u,v,x) were obtained in yields up to 60% – besides a variety of linear coupling products.12a Also the propene (4) led to olates like A1n,u,v (in yields depending on the amount of the diazonium salt), but this was fairly surprising: in view of the behaviour of the acetyl analogue of 4 (COMe instead of CN), the coupling compound (5) had been expected actually.12b
As recorded only briefly in references,1d,e treatment of the sweetening agent dulcin (6a) with nitrous acid gives rise to the fluorescent olate (A1s) (Scheme 4); this constitutes the principle of a new fluorometric determination of that drug.13a The main findings are detailed below. Compound (A1s) reverted to 6a on reduction with zinc and was identical to the material prepared from the diazonium salt (7) and potassium dinitromethanide (8) [the earlier synthesis of A1s (Scheme 3) was obviously not known to the authors].13b A1s was also formed from phenetidine (6b) and, for an X-ray study, the 2-bromoethoxy analogue (A1z) was made from 6c.13c The mechanism of the process (6a A1s) was clarified to the following extent: Nitrous acid converted 6a to the cation of 7, part of which was hydrolyzed to ethanol. The latter was oxidized to acetaldehyde which coupled with the diazonium ion at C(2); this was consistent with the fact that incorporation of C(2)-labelled ethanol gave the derivative (A1s').13d The reaction proceeds via two species (X, Y) whose structures have not been elucidated; since both materials were isolable, it could be demonstrated that the step (X Y) required nitrous acid, whereas alkali was needed for the conversion of Y into A1s. Evidence for the central role of acetaldehyde came also from the observation that addition of this component to the original reaction mixture intensified the fluorescence.13e
Nucleophilic displacement of functional groups at C(5) of appropriate tetrazolium salts is a straightforward access to olates (A1) [cf. route (b) in Scheme 1]. To date this method (formally hydrolysis) has been applied only occasionally, as exemplified by the reaction of the 5-chloro- and 5-azidotetrazolium salts (9a)5 and (10a)14 with alkali hydroxide; in both cases the product (A1b) was obtained in high yield (Scheme 5). While the azido group of 10a is also displaced by amines (see below Scheme 14), soft nucleophiles like cyanide and p-toluenesulfinate ion attack this group at the terminal nitrogen atom to give addition products, as will be shown in Scheme 16.14
Using non-quaternary tetrazoles as starting materials, three modes have been reported [cf. Scheme 1, routes (d) – (f)]. Alkylation of the tetrazolones (11a-c) with 2-chloroethanol afforded mixtures of 12 and A1, with the former predominating.15 More importantly: when tetrazolones such as 11b,d-f were treated with tertiary alcohols in conc. sulfuric acid, the attack occurred regioselectively at N(3) to give the derivatives (A1dd-ii) as the sole products.16 Small amounts of olates (A1) were found as side products on heating tetrazolyl ethers like 13 in the presence of sodium iodide: 13a rearranged into the tetrazolone (12d) and A1jj. This O N alkyl migration proceeded intermolecularly via the anion of 11, as evidenced by addition of methyl iodide which produced – as a third component – substantial quantities of the congener (12e). Possibly, also the methyl ether (13b) gave some olate (A1kk), but the traces were not isolated, nor were they separated from a parallel run of 11g with methyl iodide – in contrast to A1jj (made from 11g and benzyl iodide).17
An unexpected formation of olates (A1) was encountered on treatment of the tetrazolethiones (14a-c) with oxirane in acetic acid (Scheme 6) [cf. mode (e) of Scheme 1]. The reaction was recognized as proceeding via the thio ethers (15a-c); remarkably, these species underwent regioselective alkylation to give the salts (16a-c) which, during work-up, hydrolyzed to the olates (A1aa-cc).15 Following the same sequence, olates like A1ll-rr (mostly ionic liquids) have been prepared; here the intermediary thio ethers (15d-j) were isolated (in contrast to 15a-c), whereas the quaternary salts were hydrolyzed in situ by adding alkali hydroxide.18 The latter step also finished an earlier synthesis of A1rr that started from the thio ether (17).19
A peculiar olate-giving process constitutes the reaction of the heteropentalene mesomeric betaine (18a)20 with benzyne (Scheme 7):21 the product (A1ss) was assumed to arise from electrophilic attack at N(4), followed by sequential protonation of the phenyl group and ring opening through the residual hydroxide ion; this led to the species (19) which underwent proton transfer [cf. mode (g) of Scheme 1].
Conversions of olates (A1) into a variety of tetrazolium salts are collected in Scheme 8. As a reversal of the reaction (9a A1b) (Scheme 5), treatment of A1b,e,j with phosphoryl chloride and tetrafluoroboric acid gave the 5-chlorotetrazolium salts (9a-c) in good to excellent yield.7, 14 Meerwein alkylation transformed the respective substrates (A1) into the 5-ethoxy salts (20a-e).3a,c Further reactions of the olate (A1b) include its conversion into the dicationic ether salt (21)22 and the metal complex (22).23
b) Tetrazolium-5-thiolates (A2) and -5-selenolates (A3)
In contrast to the ring transformations of Scheme 2, attempts to rearrange thialogues of 1 (S instead of O) into thiolates (A2) failed.3b Thereupon, the authors successfully treated 20a (and analogues) with sodium sulfide [Scheme 9; cf. mode (b) of Scheme 1]. Later, it was found that application of the 5-chloro salt (9a) improved the yield.5 For preparing A2a, the methylthio function served as a leaving group,19 as in the earlier synthesis of the 3-ethyl-1-phenyl congener.1f The selenolate (A3a) was also best made from 9a, whereas employment of the iodo substrate (9e) was deteriorating.5 A novel strategy for A2 constitutes the conversion of olates (A1) by Lawesson's reagent [cf. route (i) of Scheme 1]. This method was first applied for the process (A1b A2b).24 Soon thereafter, the derivatives (A2c,d) were made in the same manner.5
Another new synthesis of thiolates (A2) was found in the behaviour of tetrazolethiones (14) towards tertiary alcohols in sulfuric acid; established examples are the derivatives (A2e-i) (Scheme 10).16a, 25 The reaction corresponds to mode (d) of Scheme 1 and resembles the process (11 A1) (Scheme 5). Whereas tert-butyl alcohol only yielded the thiolates (A2h,i), alkylation with adamantan-1-ol was not regioselective. The latter
applies also to acetylation (Scheme 11),26 but here it is questionable whether the third component obtained from 14'e,m has structure (26), as thought by the authors.26b Tetrazoles with a 1,2-substitution pattern are not known, and a DFT study on acetyl derivatives having R = Me revealed that the respective compound (26) is higher in energy than the thiolate (A2).26c A similar relationship has recently been calculated for 1,2-dimethyltetrazol-5(2H)-one and the isomeric olate (A1rr).1c
Specific reactions of A2 and A3 are shown in Scheme 12. Heating of the derivatives (A2b) and (A3a) with hexacarbonylmolybdenum(0) gave the complexes (27) and (28), the latter in low yield. Attempts to prepare the chromium analogue of 27 and the iron complex corresponding to 22 (Scheme 8) failed.23 As expected, nitric acid smoothly desulfurized the thiolates (A2b-d) to the 5-unsubstituted tetrazolium salts (29a-c).5 A remarkable product, the mesoionic thiocarbonyl ylide (30), arose on sequential treatment of A2b with bromine and sodium dicyanomethanide. The reaction was thought to proceed via a 1:1 bromine adduct that separated from the reaction mixture, but could not be fully characterized because of instability.27
c) Tetrazolium-5-aminides (A4)
The simplest aminide (A4a) has been generated in a frozen matrix by UV light-induced tautomerism of the tetrazole (31) (Scheme 13). Of all possible structures formed through proton migration, the ap conformer of A4a has been calculated to be lowest in energy (next to 31).28 Homologues of the earliest derivative (A4b)29 were obtained from the salts (9), (10), and (32), i.e. after modes (b) and (g) of Scheme 1. Of these entries, deprotonation proved to be the most expeditious one (see 32b A4c,30 32c A4d14). Ammonolysis, exemplified with 9a, mainly gave A4g, as the desired aminide (A4d) reacted further.14 Photolysis of azides such as 10a,b turned out to be complex, since both triplet and singlet nitrenes are involved; in general, the former lead to aminides (A4),7, 31 the latter to azapentalenes (18).7 So, when 10a was irradiated in methanol, A4d and 18a were formed in equal quantities. However, on addition of the triplet sensitizer acetophenone,
the formation of 18a was suppressed. Singlet promotors like pyrene and anthracene should favour 18a, but, surprisingly, these additives increased the yield of A4d (relative to 18a) and gave N-substituted aminides (A4j) and (A4k) as side products (see Scheme 14).
Another unexpected result was encountered with the mesityl derivative (10c): instead of benzylic hydrogen abstraction to give the tricycle (33a), the solvent was affected to afford A4f in good yield.7 However, changing from methanol to acetonitrile, the amount of A4f was reduced and – as remarkable products – the tricycles (33b) and (34) were found, which obviously arose via 33a.31 Regarding irridiation of 10a,b in acetonitrile, instead of the aminides (A4d,e) conspicuous amounts of acetanilide and p-acetotoluidide were formed (besides 18a,b).7 Finally, hydrolysis of the cyclic amidine function in the eight-membered ring of (34) led to another unsubstituted aminide (A4h).31
N-Substituted aminides (A4) have been obtained from both the tetrazolium salts (9) and (10) (Scheme 14). Treatment of 10a with the aminide (A4d) or 1-phenyltetrazol-5-amine furnished the derivatives (A4g) and (A4i), while photolysis in the presence of pyrene or anthracene produced small amounts of A4j and A4k (cf. preceding paragraph).7, 14 But more frequently the salts (9) served as substrates, and their reactions with primary amines proceeded satisfactorily in most cases ( A4i,j,l-t).5, 7, 8, 14, 21, 32, 33 The procedure has also been applied to the synthesis of polymers of the type (A4u) which are useful for electrolytes of lithium batteries.33 In the same fashion, certain bisaminides such as A4v-y have been provided for electrochemical investigations; these materials represent reversible four-stage redox systems (Scheme 15).34
Following the above method, also derivatives bearing a heteroatom at the aminide function, such as A4z and A4aa-ff, have been prepared, viz. by reacting 9a with hydroxylamine and the appropriate hydrazines (Scheme 16).14, 34, 35 In the first case, part of the substrate underwent hydrolysis to give the olate (A1b). Using N,N-diphenylhydrazine, the expected hydrazinide (A4ee) was obtained in low yield only, because rearrangement took place to afford the aminide (A4hh).14 Since, as shown, hydrazine hydrate reacted twofold ( A4aa), a hydrazinide having a free N–NH2 function like A4gg had to be approached via the benzamido derivative (A4ff).35
A special class of heteroatom-substituted aminides, viz. the triazenides (A4ii,jj), was discovered by treating the salt (10a) with the soft anions cyanide and p-toluenesulfinate, which – in contrast to hard nucleophiles like hydroxide ion and amines (cf. Schemes 5 and 14) – do not displace the azido group. The same kind of addition was found with triphenylphosphine (another soft nucleophile).14
Many aminides (A4) have been submitted to conversions, part of which led to further representatives of A4 by simply modifying the functional group [cf. mode (i) of Scheme 1]. Thus, treatment of the unsubstituted aminide (A4d) with reagents having electron-deficient multiple bonds gave the addition products (A4kk) and (A4mm) (Scheme 17); using tetracyanoethene, the primary adduct extruded hydrogen cyanide to yield compound (A4ll).21 As expected,36 in no case did A4d react as a (masked) azimine dipole [see, however, the behaviour of the 1,2,3,4-oxatriazolium-5-aminide (1b) towards an isocyanate3a].
Substitution products, such as A4nn-pp, arose from treatment with acyl chlorides or a benzenediazonium salt;31, 35 the latter reaction implies an alternative (i.e. N–N forming) process giving a triazenide.
Extending the above treatment with DMAD to N-aryl congeners, such as A4p,t, no addition products were obtained; rather, the respective Michael adducts (α1) and (α2) (concomitantly formed) underwent further conversions (Scheme 18): The first species hydrolyzed to the olates (A1b,c), whereas the second rearranged
to the tetrazene-substituted dihydropyridines (β) which, after extrusion of benzenediazonium ion, cyclized to the pyrazolo[3,4-b]pyridines (35a,b). A complementary experiment of A4p with benzyne afforded the tetrazolium salt (36); this compound arose through attack of the anionoid carbon of the adduct (γ) at C(5) of the tetrazolium moiety, followed by C–N bond cleavage of the four-membered ring of intermediate (δ).21
A unique reaction cascade started when the 'biaminide' (A4aa) was exposed to DMAD or dibenzoylethyne: The 1: 1 adduct (ε) was attacked at C(5) by the hydroxide ion (obviously from traces of moisture), with the consequence of expelling the adjacent tetrazole portion as olate (A1b); the residual enehydrazinic fragment underwent proton migration to afford the hydrazone derivatives (A4qq) and (A4rr) as final products. In a parallel run, compound (A4qq) was obtained in high yield from DMAD and the hydrazinide (A4gg).21
Further studies with the 'biaminide' (A4aa) comprise protonation, alkylation, and redox reactions (Scheme 19).34 Using acetic and trifluoroacetic acid, A4aa could be selectively mono- and diprotonated to afford the
derivatives (A4ss) and (37). But methylation with methyl iodide (even used in excess) only allowed mono alkylation (A4tt). Treatment with lead tetraacetate and tetrafluoroborate ion gave the radical salt (A4uu), a remarkably stable compound, of which – for EPR and ENDOR spectra – also 15N-labelled derivatives were made. Subsequent reaction with conc. nitric acid afforded the azo compound (38), while treatment of A4uu (and likewise 38) with zinc powder restored the starting material. Further reduction did not give defined products, but voltammetric studies showed that A4aa is both oxidizable and reducible electrochemically, demonstrating that the compound is part of a reversible four-stage redox system. As for the 'phenylogue' (A4x) of Scheme 15, reduction with zinc proceeded readily ( A4vv), i.e. after the pattern that converted A4p to N,N '-diphenylguanidine, but chemical oxidation failed – in contrast to the electrochemical methods, which were also effective with the congeners (A4v,w,y).34
Selected aminides (A4) including the azo salt (38) have been studied photochemically (Scheme 20).35 The results were shown to depend on the solvent. Thus, when 38 was irradiated in acetone, the (CH-acidic) solvent added to the double bond, giving compound (39) besides its conjugate base (A4ww). Changing to diethyl ketone, no addition took place, but the N=N bond was cleaved to afford the aminotetrazolium salt (32c). Using methanol, 38 as well as the radical salt (A4uu) were reduced to 37 (BF4 for CF3CO2) which on base treatment gave A4aa. Yet, considering that the phenylazo salt (40) releases dinitrogen and benzene to afford 29a, the substrate (38) should have led to the 1,1',3,3'-tetraphenyl-5,5'-bitetrazolium ion.35
Also irradiation of the triazenides (A4ii) and (A4jj) gave product patterns that depend on the solvent. Using methanol, A4jj was mainly transformed into the N-unsubstituted aminide (A4d) and only negligibly into the tosyl derivative (A4oo), while in acetonitrile the yield of A4oo increased, although now, surprisingly, the azapentalene (18a) became the main product. Regarding A4xx, again a smaller quantity was found in methanol. Turning to the substrates (A4yy) and (A4i), both compounds reacted only in the presence of the sensitizer acetophenone. In the first reaction, a mixture of A4d (predominant material) and the hydrazone (A4zz) arose (the latter presumedly via transient A4gg); in the second case, ring cleavage occurred to give the guanidine (41).35
d) Tetrazolium-5-methanides (A5)
The material obtained in 1893 from 42 (or 4-oxoheptanedioic acid) and benzenediazonium hydroxide and at that time viewed as the biformazan (43)37a has later been recognized as the methanide (A5a)38 (cf. ref.1f) (Scheme 21). The stepwise formation [which in part resembles route (c) of Scheme 1] was studied in detail, and it was shown that the fifth diazonium ion is required for dehydrogenation.38 In order to complement ref.1f, it might be added that an analogue (A5b) was prepared in the same way (low yield too) and, second, that the reduction of A5a following the procedure of ref.37b did not give the diamidrazone (44) as earlier described,37a,b but afforded the 'mixed' functionalized derivative (45).38
An expedient entry to derivatives of class (A5) consists in nucleophilic displacement with 5-functionalized tetrazolium salts [cf. route (b) of Scheme 1]. A systematic study has revealed that specific leaving groups are needed (Scheme 22):5, 22 On action of the conjugate base of malononitrile, the desired product (A5c) was obtained from substrates having a chloro (9a), bromo (9d), ethoxy (20a) or tetrazoliumyloxy ligand (21). However, with electropositive substituents – as existing in 9e and C'a – the carbanion preferentially attacked the ring at N(2) to give a transient dihydrotetrazole (with 9d only in part); this species fragmented to eventually afford the hydrazone (46) and the cyanamide (47). The same reaction took place when 5-unsubstituted tetrazolium salts like 29b-d (Scheme 12) were treated with malononitrile and base.5
Also the congener (A5d) was easily available.22 But experiments with indane-1,3-dione failed: the salts (9a), (20a), and (21) all underwent hydrolysis to the olate (A1b) instead of being converted to the expected methanide (A5).5
A sesquifulvalene-type methanide (A5e) and some benzo-fused analogues, such as A5f-h, arose readily when the tetrazolium salt (20a) was treated with the sodium or lithium salts of the respective hydrocarbons (Scheme 23). The products are stable as crystals, but the solutions turned out to be air-sensitive. Protonation with trifluoroacetic acid occurred at the site indicated; the divergent position with A5f relative to A5e avoids a less stable o-quinonoid structure.40
Treatment of A5e with a dienophile such as tetracyanoethylene showed that the substrate has no olefinic but aromatic character (by virtue of the 5-substituent): instead of the cycloadduct (48), the substitution product (A5i) was formed (Scheme 24) [cf. route (i) of Scheme 1]. This property was confirmed by reactions with electrophiles like methyl chloroformate as well as the benzenediazonium and 2-bromo-1-methylpyridinium
salts, which gave rise to the (monosubstituted) derivatives (A5j + A5j'), (A5k + A5k'), and (A5n). When using the more reactive N-halosuccinimides, full substitution took place ( A5l,m).41 Treatment of A5e with the appropriate metal reagents produced the complexes (A5o,p); these materials are stable as solids, but their solutions rapidly released the free 'methanide' on exposure to air.23 Also the indenide (A5f) proved capable of undergoing electrophilic substitution, as evidenced by formation of A5q; expectedly, the attack occurred regioselectively (in contrast to A5e); this was confirmed by the reaction of 20a with methyl indene-3-carboxylate.40b
Finally, as interesting congeners of the tripolar compound (A5n), the derivatives (A5r-t) were formed on treatment of the 'methanides' (A5e) and (A5f) with the dicationic ether salts (21) and (49); both reagents showed comparable reactivity (Scheme 25). In the case of A5f, again substitution occurred exclusively at C(3) of the bicycle.42
2) MESOIONIC TETRAZOLES OF TYPE (B)
a) Tetrazolium-5-olates (B1)
Oxidative cyclizations of C-functionalized NNCNN chains constitute the traditional entry to olates (B1).1d–f Recent examples include oxidation of diphenylcarbazide (50) with lead tetraacetate43 or p-benzoquinone44 as well as ozonolysis of the ylides (51a,b)43 (Scheme 26), i.e. reactions that follow the routes (n) and (p) of Scheme 1. The latter experiment aimed at detecting the open-chain valence isomers of B1c,d: compounds (51) having O instead of PPh3, but these species were found to cyclize extremely rapidly once they were formed43 [see, however, structure (59) in Scheme 27]. Regarding the use of chloroformazans [cf. route (l) of
Scheme 1], the old process (52b B1b)46 (reviewed previously1d,e) may be complemented by the reaction of 52a,47 the more so since in that paper the modern representation of type (B) as a mesoion appears for the first time! New precursors to B1 are the tetrazolium derivatives (53b) and (54b): submitted to hydrolysis, they yielded the olates (B1c) and (B1a), respectively [cf. route (m) of Scheme 1].43, 45
Conversions include treatment of B1a with cobalt(II) halides to afford the complexes (55a,b)44 as well as the reaction with the nitrosyl cation to yield the interesting peroxide (56),43 while the products (53a)48 and (57)22 arose from methods already applied in the A1 series [cf. Section (1a)].
As formal congeners of olates (B1), quinonoid derivatives (B1') have been made (Scheme 27). Compounds like B1'a-f were obtained from salts (58a-f) on treatment with base. As a consequence of the pronounced electron-withdrawal of the tetrazolium ring, these precursors are stronger acids than the parent phenol.49 The material resulting from 58g differed spectroscopically (UV, NMR) from the derivatives (B1'a-f) so as to point to the quinone methide (59) rather than the dipolar 'phenolate' (B1'g).49b
b) Tetrazolium-5-thiolates (B2)
The classical entry to these mesoions consists in oxidative cyclization of thiocarbazones (60) [cf. route (n) of Scheme 1], and several new preparations have been reported. Apart from manganese dioxide39 and the traditional potassium hexacyanoferrate(III),50–52 new oxidants like hydrogen peroxide53, 54 and air54, 55 have been used (Scheme 28). Electrochemical oxidation of 60a by cyclic voltammetry (CV) generated a radical that dimerized to the disulfide (61) which gave the fully oxidized species (62) in turn; the latter should have cyclized chemically to B2a, but this did not occur on the CV time scale.51 In a diluted ethanolic medium, manganese(II) chloride catalyzed the oxidation of the thiocarbazide (63) to 60a, which disproportionated into B2a and 63.56 Theoretical studies on B2 have been extensive; out of the various findings,54, 55, 57 the new resonance hybride structure (B2a') showing refined atomic charge distributions may be selected here.54
Conversions of thiolates (B2) are detailed below (Scheme 29). Oxidation of B2a with sodium periodate produced a mixture of the sulfonate (54b) and the disulfide (66) as main components, while with conc. nitric acid the 5-defunctionalized salt (65a) arose, obviously via sequential release of sulfur dioxide from the intermediary sulfinate (54a) and protonation of the transient carbene (D) (cf. Scheme 12: A2 29).45
Protonation equilibria have been investigated with B2a and some analogues, showing that the substrates are Hammett bases; proton attack was assumed to occur mainly at the exocyclic sulfur atom ( 64a-e), but to a minor extent at nitrogen ( 64') too,52 a view that was verified later.54 Reactions with other electrophiles concern the preparation of the deuterated 5-(alkylthio)tetrazolium salts (67a-c); these materials served as precursors to the respective formazans, which were sought for comparative purposes.50 The sulfur atom is also attacked by metal ions; but because of the plethora of examples, the field can be covered appropriately only by a specialized review. Nevertheless, attention might be drawn to the tetrahedral complexes (68a,b):
here, interestingly, the single ion magnetic anisotropy parameter (D), which was found positive in the case of the oxalogues (55a,b) (Scheme 26), switched to negative with a significant change in magnitude.44
Sequential treatment of B2a with bromine and the conjugate base of an active methylene compound gave mesoionic thiocarbonyl ylides like 70a,b (Scheme 30). The reaction proceeded through a labile bromine adduct believed to be 69.27, 58 However, using esters of malonic as well as cyano- and acetoacetic acid, the ylides (70c-e) eluded isolation, and the 1,3,4-thiadiazoles (71a-c) were obtained instead; these compounds apparently arose through 1,5-cyclization of the open-chain valence isomers (70'). Also B2a was found to ring-open, namely in the reaction with diazo compounds, which led to the derivatives (71a,c,d).58
Resuming earlier reactions with unsaturated systems (cf. ref.1f), B2a was submitted to dibenzoylethyne, N-ethylmaleimide, diphenylcyclopropenethione, and, again, benzyne (Scheme 31).60a Since the latter was applied under milder conditions than originally, the azimine-functionalized benzothiazole (72a) could be isolated; an analogous product resulted from dibenzoylethyne ( 72b). However, the primary adduct with the maleimide underwent oxidation, followed by loss of phenylnitrene to afford the phenylazo-substituted pyrrolothiazole derivative (73). Finally, in the reaction with the cyclopropenethione, the thiadiazepine (74)
was formed; the isomeric structure having a 7-thioxo group could be excluded spectroscopically. All of the above conversions are understood as proceeding through the acyclic valence isomer of B2a.
c) Tetrazolium-5-aminides (B4)
Following the concept of aminolysis of 5-chlorotetrazolium salts, which had proved fruitful in the isomeric A4 series [see Section (1c)], the substrate (53a) was treated with various primary amines in the presence of triethylamine [cf. routes (m) and (o) of Scheme 1].48 However, using reagents with an α methylene group, neither the expected aminotetrazolium salts (75a-d) nor the corresponding aminides (B4a-d) were found; instead, the phenylazo-substituted triazoles (79a-d) were isolated (Scheme 32). Apparently, the aminides (B4a-d) once formed ring-opened to the valence isomers (76) which, after proton loss, gave the species (77); the latter cyclized to the dihydrotriazoles (78) which in turn were oxidized to 79. A second pathway seems to involve an aminoformazan (80) which arose from 75 through reduction by the transient triazole (78): indeed, treatment of 3-chloro-1,5-diphenylformazan (52a; Scheme 26) with benzylamine led directly to 79a, the respective formazan (80: R = Ph) being elusive.
While triethylamine induced a cascade reaction to afford 79, the intermediary salts (75), such as 75a,b, could be isolated when using an inorganic base. However, separate experiments with these salts have not been described.
In contrast to primary amines of the RCH2NH2 type, isopropyl- and tert-butylamine gave the expected salts (75e,f) even in the presence of triethylamine, but these substrates do not appear to have been checked for aminide formation.48
Aminides having acceptor groups like B4e-g were obtained by treating the aminotetrazolium salts (81a,b) with sulfonyl chloride1d, 61 and nitrous acid,59, 61–63 respectively (Scheme 33) [cf. route (m) of Scheme 1]. An attempt to get the derivative (B4g) by trapping the 5-lithiotetrazolium salt (105c; X = BPh4) (Scheme 39) with dinitrogen oxide was unrewarding.63
Conversions of class (B4) members include methylation of B4f to afford the salt (82)61 and, as reviewed earlier,1d,e ring transformation to the N-oxide (83) in hot ethanol or water62 – a process that is comparable to the above sequence (B4 76 / 77 78). Whether also the nitroso analogue (B4g) is capable of undergoing this rearrangement, has not been investigated; only pyrolysis in the gas phase was studied, but this produced insignificant results.43
d) Tetrazolium-5-methanides (B5)
Applying principle (n) of Scheme 1, treatment of the formazans (84a,b) with either (an aminyl forming) tetraarylhydrazine or lead tetraacetate gave oxidation products that were presented as the methanide (B5a) and the nitrosoethylene (85), respectively (Scheme 34).65 Since the latter, however, was reviewed to exist in the cyclic form (B5b),1f DFT calculations were conducted to show that the open-chain valence isomer (85) is energetically favoured.66 Access to the cyano- and alkoxycarbonyl-substituted methanides (B5c,d) was achieved by nucleophilic displacement [cf. mode (m) of Scheme 1]. Treatment of the dicationic ether salt (57) with malononitrile in the presence of a base gave B5c,22 after an earlier attempt of converting the substrate (86) had proved unrewarding.39 Later, the analogue (B5d) was made accordingly,59, 67, 68 but the authors did not provide details, except for referring to the procedure applied with B5c.22 An unusual formation of a methanide (B5) occurred in the mass spectrometer under EI conditions: the quinonoid compounds (B1'a-c) fragmented to the cyclopentadienide (B5e).49b Thereupon, the authors undertook to synthesize the compound by flash vacuum thermolysis, but the results do not seem to have been disclosed.
Experimental Structural Methods
All derivatives (A) and (B) dealt with in Sections (1) and (2) have been characterized by physical methods. Literature data on bond lengths and angles are available for an increased number of systems (Table 1); the five-membered ring of the compounds listed is planar, and – also expectedly – in all cases the C–Z bond length was found intermediate between a double-bond and a single-bond distance.
Spectral data have been determined in great number (for a selection, see Table 2). Outstanding systematic studies constitute the multinuclear NMR investigations conducted by the Jaźwiński group, and here also a range of other azolic mesoions of the type (A) were looked at; the majority of the findings have been reviewed.71–73
The collection of UV/Vis data shows that most derivatives are coloured materials; with lacking data, the compounds were described as such. A prominent feature is the pronounced negative solvatochromism which has been studied with several derivatives, viz. the methanides (A5c,d) and (B5c) (Schemes 22, 34),22 the thiolates (B2a,b,d–f) [including analogues having Ar = 2-Cl/F/MeOC6H4 and 4-FC6H4] (Scheme 28),53 the cyclopentadienide (B5e) and its benzo-fused congeners (B5f-h) (Scheme 23),40a,b and, finally, the phenolates (B1'a-e,g) (Scheme 27).49a,b A comparison of the isomers (A5c) and (B5c) shows that the solvatochromism is stronger with the latter derivative.22
From published MS spectra it is apparent that – under EI conditions – compounds of the A series are more stable: quite a number of derivatives gave parent ions of high intensity.
The radical cation (A4uu) (Scheme 19) and its bis- and tetra-15N-labelled analogues were characterized by EPR spectroscopy (the labelled species also by 14N and 15N ENDOR spectroscopy which revealed that the largest N hyperfine coupling constants result from the central bridge).34
3) MESOIONIC TETRAZOLYLIDENES OF TYPE (C)
It is with these species that the general term 'mesoionic carbene' has been introduced into the literature.5, 81 There are two procedures of generation (Scheme 35): (i) Deprotonation of 5-unsubstituted tetrazolium salts (29) and (ii) treatment of the 5-azido congeners (10) with azide ion [cf. routes (j) and (k) of Scheme 1].
(i) The carbene (Ca) was postulated as intermediate in an H/D exchange experiment with 29d to give the deuterated salt (87) (even below pH 7), whereas potassium hydroxide (50% in water) was shown to split the substrate into diazomethane (89) and potassium methylcyanamide (90). These compounds originated from the cyanotriazene (88a) which arose through ring cleavage of Ca.82 But also milder conditions caused ring opening, as observed on treatment of 29a with DBU in dichloromethane; the isolated material, first viewed as 88b,5, 14 was later revised to be the azo compound (91).83
(ii) Treatment of the salt (10a) with sodium azide not only afforded 91 but also aminides like A4yy and A4i [cf. route (h) of Scheme 1], whereas the congener (10b) gave A4aaa exclusively. With reference to Balli's 'azidinium' salts and their carbene-based formation,84 these aminides were thought to arise via Cb and Cc, respectively.14 The behaviour of 10a has been reviewed elsewhere,2c but without realizing the correction in ref.83 where the putative triazene (88b) was discarded.
The nucleophilic nature of the carbene (Cb) – more properly expressed by the mesomeric ylide structure – was also demonstrated by trapping with a diazonium salt ( 92) (Scheme 36).5 Interception was likewise successful with tetracyanoethene or an electronegatively substituted 1,2,4,5-tetrazine, giving the methanide (A5u) or a mixture of two 4H-pyrazoles (95, 96) and the methanide (A5v).83 The latter materials arose via
the joint intermediate (93) showing dichotomous behaviour: extrusion of dinitrogen led to the spirane (94) which was stabilized by tetrazole ring opening, whereas contraction of the tetrazine moiety gave A5v. The interesting route to this compound provides a new direct access to 2H-tetrazoles by ring transformation.85 However, on attempts to trap Cb with weaker electrophiles (N-phenylmaleimide, fumaronitrile, DMAD), the tetrazole ring did not survive: only fragments of it were incorporated to yield phenylated maleimides, fumaronitriles, and cyanamido-substituted N-phenylsuccinimide and DMAD.83 Attempted interceptions of Cb with alkyl halides and carbonyl compounds were unrewarding too.
Metal complexes of C have been made in considerable number (Schemes 37, 38). The first examples were the derivatives (C'a-c), obtained by mercuration of the salts (29b-d). Studying the stability of the C–Hg bond, it was found that heating with conc. hydrochloric acid returned the starting compounds (29), whereas action of base released the ligand (Cb); moreover, treatment with halogens produced the tetrazolium salts
(9a,d-g), the chloro derivative of which was converted into the palladium complex (C'd).5 For synthesizing the rhodium congeners (C'e,f), again a 5-unsubstituted tetrazolium salt was used.83 The same applies to the silver (C'g), iridium (C'h), and chromium complexes (C'i), whereas the derivatives (C'k) and (C'l) were made through carbene transfer reaction and ligand exchange.86 As a congener of C'i, also the iron complex
(C'j) has been provided (details not disclosed).87 But the true incentive to prepare these materials was to compare them with the corresponding complexes (98') of the 'normal' carbene (98). Indeed, conspicuous differences emerged: The compounds (C') were more stable than their isomers (98') and were obtained in higher yields, because the latter – like the free carbene (98) – tend to release dinitrogen. Moreover, detailed spectroscopic, crystallographic, and computational studies led the authors to conclude that the carbene (Ca) has a stronger donor strength than 98. While considering also the carbene complexes (99') and (100'), they found through DFT calculations a decreasing donor strength in the order 100 > Ca > 99 > 98.86 Another DFT study directed to all possible 'normal' and 'abnormal' azolic carbenes (a total of 14) showed that the donor strength of Da surpasses that of Ca.88 Thermodynamically, Da proved to be the least stable species: it is higher in energy than Ca by ca. 30 kcal/mol and lies even 47 kcal/mol above the 'normal' carbene (98), whereas the carbene (101) – chemically irrelevant because of the 1,2-substitution pattern (cf. ref.26c) – is intermediate between Ca and Da.88 Recently, this relationship was also found with the dimethyl-substituted olates (A) and (B) and the two covalent tetrazol-5-ones.1c
Finally, to synthesize the nickel complex (C'm), a divergent route was followed: the entry took advantage from the regioselective quaternization of the tetrazole ring in the derivative (102); but to accomplish the process, a strong electrophile was needed, ethyl iodide – even used in excess – did not affect the substrate.89 Notably, C'm combines the features of a 'normal' and an 'abnormal' carbene (benzimidazole and tetrazole, respectively).
Experimental structural methods
Crystallographic data are available for the complexes (C'e,f),83 (C'g-i,k,l) and (98'h,i)86 as well as (C'm);89 for a direct comparison of structural parameters of the isomers (C'h) and (98'h), see Table 3. The elongated bonds C(2)–C(3) and C(4)–C(5) of the former derivative point to a more pronounced donor strength of the 'abnormal' ligand (Ca).86
Spectral data were determined throughout; for a selection, see Table 4. The majority of the complexes (C') are coloured materials, but UV/Vis data have been reported for two derivatives only (C'e,f).83 A detailed comparative EIMS study has been performed with the isomers (C'i,j) and (98'i,j).87 In the 13C NMR spectra, the tetrazole carbon appears at relatively low field, depending on the metal. Data that testify to an increased donor strength of the carbene (Ca) include, inter alia, the upfield shift of the low-field olefinic signal of the cyclooctadiene (COD) ligand in C'h and, in the case of carbonyl complexes like C'i, the appearance of the CO stretching bands at lower wavenumbers.86
4) MESOIONIC TETRAZOLYLIDENES OF TYPE (D)
Similarly to the congeners (C), the class (D) has first been envisaged fifty years ago as an intermediate of base-catalyzed H/D exchange, viz. Db in the course of the process [65a (X = Cl) 106a] (Scheme 39).90 But the 'preparative chemistry' of these carbenes did not begin until the early 1990s. Three procedures of generation have been described [cf. routes (s) and (r) of Scheme 1]: (i) desulfurization of the thiolate (B2a), (ii) dephosphonionation of the substrate (103b), and (iii) deprotonation of the salts (65a-e).43, 45, 63 The latter reaction is known to proceed more slowly than the analogous entry to the carbenes (C) (cf. also ref.43). – For another review of these methods, see reference.2c
(i) Recurring to Section (2b), treatment of B2a with sodium periodate led, inter alia, to the sulfinate (54a) which by loss of sulfur dioxide gave the carbene (Db); the latter ring-opened to the cyanoazimine (104a),91 but since also some olate (B1a) was found, it was speculated that part of Db took up atmospheric oxygen.45
(ii) On exposure to water or mineral acid, the tetrazolium salt (103b) was hydrolyzed to triphenylphosphine oxide and the 5-defunctionalized derivative (65b; X = BF4), the latter arose through protonation of transient Dc; accordingly, treatment of 103b with deuterium oxide gave the derivative (106b).43 However, on action of cesium fluoride, the functional group of 103b was split off as triphenylphospine difluoride to leave Dc which ring-opened to 104b. Yet, using an electrophilic trapping agent such as bromine, Dc was intercepted to give compound (53b') which, through excess cesium fluoride, was converted to the 5-fluoro derivative (53b); this sequence demonstrated that compound (53b) does not simply arise by nucleophilic attack of fluoride ion on the 5-position of 103b.45
(iii) Treatment of 65a-e with strong bases like butyllithium or lithium hexamethyldisilazide generated the carbenoids (105a-e).45, 63 These species, which could be studied spectroscopically at –100 °C, showed the behaviour of D: On warming to room temperature, they ring-opened to the azimines (104a-e), and action of deuterated sulfuric acid on 65b gave the above salt (106b).45 Trapping of 105b,c with a benzenediazonium ion led to azo compounds like 107b,c, whereas interception of 105c with tosyl azide gave the 5-azido salt (108c) [the primarily formed aminide (B4h) was elusive]; however, treatment with chlorine or bromine restored the starting salts (65; X = Cl or Br) instead of yielding 5-halotetrazolium salts like 53b'; also trapping with dinitrogen oxide failed [cf. Section (2c)].63
Metal complexes of D seem to have been made only once: Treatment of 65b (X = HgCl3) with mercury(II) chloride in the presence of lithium hydroxide gave a material of the composition (D'a), auxiliary bases like
hydrogencarbonate or acetate ion turned out to be too weak.43 Also no reaction occurred between the metal salt and the carbenoid (105c; X = BPh4) when generated with butyllithium.63 On pyrolysis, compound (D'a) released the carbene (Dc), as evidenced by detection of the azimine (104b); attemps of bromination to give the salt (53b') failed.43
CONCLUSION
Comparing the preparative achievements made with the classes (A) and (B), some areas of the B series wait for further studies: (i) A conspicuous gap concerns the aminides (B4); there are only derivatives having electronegatively substituted functions, while representatives with the parent NH functionality and simple NR groups are unknown. Considering the instability of the derivatives (B4) having Z = NCH2R, a general study of the ring opening of B4, i.e. the valence isomerism {tetrazolium tetraazapentadiene}, is needed. (ii) Turning to the series (B5), it is still open whether the cyclopentadienide (B5e) detected in the EIMS spectra of the phenolates (B1') can also be synthesized. (iii) In view of the theoretical conclusion that the carbene (D) exhibits a more pronounced donor strength than its isomer (C), the preparation of appropriate metal complexes (D') seems desirable.
References
1. a) W. D. Ollis, S. P. Stanforth, and C. A. Ramsden, Tetrahedron, 1985, 41, 2239; CrossRef b) according to an advanced classification, type (A) belongs to conjugated HMBs (Class 1B), whereas compounds of type (B) represent semi-conjugated HMBs (Class 3) or, more rigorously, pseudo-semi-conjugated HMBs (Class 5): C. A. Ramsden, Tetrahedron, 2013, 69, 4146; cf. also ref.1c; c) W. P. Oziminski and C. A. Ramsden, Tetrahedron, 2015, 71, 7191; CrossRef d) W. D. Ollis and C. A. Ramsden, 'Advances in Heterocyclic Chemistry: Meso-ionic Compounds', Vol. 19, ed. by A. R. Katritzky and J. A. Boulton, Academic Press, London, 1976, pp. 1–122; e) C. A. Ramsden, 'Comprehensive Organic Chemistry: Meso-ionic Heterocycles', Vol. 4, ed. by D. H. R. Barton and W. D. Ollis, Pergamon Press, Oxford, 1979, pp. 1171–1228; f) C. G. Newton and C. A. Ramsden, Tetrahedron, 1982, 38, 2965; CrossRef g) A. M. Simas, J. Miller, and P. F. de Athayde Filho, Can. J. Chem., 1998, 76, 869; cf. also refs.1h,i; h) C. W. Bird, Heterocycles, 1994, 37, 249; C. W. Bird, Tetrahedron, 1985, 41, 1409; i) Yu. I. Nein and Yu. Yu. Morzherin, Izv. Akad. Nauk, Ser. Khim., 2012, 1103; Russ. Chem. Bull., 2012, 61, 1111. CrossRef
2. The new symbols (C) and (D) are tentatively introduced as an extension of (A) and (B). – Regarding the term 'abnormal' for this type of N-heterocyclic carbenes (NHCs), see, for example; a) O. Schuster, L. Yang, H. G. Raubenheimer, and M. Albrecht, Chem. Rev., 2009, 109, 3445; CrossRef b) A. Krüger and M. Albrecht, Aust. J. Chem., 2011, 64, 1113; CrossRef c) A. Schmidt, S. Wiechmann, and T. Freese, ARKIVOC, 2013, i, 424; d) R. H. Crabtree, Coord. Chem. Rev., 2013, 257, 755; CrossRef e) M. Albrecht, 'Advances in Organometallic Chemistry: Normal and Abnormal N-Heterocyclic Carbene Ligands: Similarities and Differences of Mesoionic C-Donor Complexes', Vol. 62, ed. by P. J. Pérez, Academic Press, London, 2014, pp. 111–158.
3. a) R. N. Hanley, W. D. Ollis, and C. A. Ramsden, J. Chem. Soc., Perkin Trans. 1, 1979, 736; CrossRef b) R. N. Hanley, W. D. Ollis, and C. A. Ramsden, J. Chem. Soc., Perkin Trans. 1, 1979, 741; CrossRef c) this reaction3a not reviewed.1f.
4. J. Wood, R. F. Jones, and J. H. Davies, GB 2015878; Sep. 19, 1979 (Chem. Abstr., 1980, 93, 26443).
5. S. Araki, Y. Wanibe, F. Uno, A. Morikawa, K. Yamamoto, K. Chiba, and Y. Butsugan, Chem. Ber., 1993, 126, 1149. CrossRef
6. J. Jaźwiński, O. Staszewska, L. Stefaniak, S. Araki, and G. A. Webb, J. Mol. Struct., 2000, 523, 103. CrossRef
7. S. Araki, H. Hattori, N. Shimizu, K. Ogawa, H. Yamamura, and M. Kawai, J. Heterocycl. Chem., 1999, 36, 863. CrossRef
8. J. Jaźwiński, Z. Rozwadowski, D. Magiera, and H. Duddeck, Magn. Reson. Chem., 2003, 41, 315. CrossRef
9. a) C. Christophersen and S. Treppendahl, Acta Chem. Scand., 1971, 25, 625; CrossRef b) C. Christophersen and S. Treppendahl, Acta Chem. Scand., 1972, 26, 858. CrossRef
10. R. G. Dubenko, V. M. Neplyuev, and P. S. Pel'kis, Zh. Org. Khim., 1966, 2, 504; J. Org. Chem. USSR, 1966, 2, 506.
11. a) V. M. Neplyuev and P. S. Pel'kis, Zh. Org. Khim., 1974, 10, 1725; J. Org. Chem. USSR, 1974, 10, 1740; b) V. M. Neplyuev, R. G. Dubenko, and P. S. Pel'kis, Zh. Org. Khim., 1969, 5, 1832; J. Org. Chem. USSR, 1969, 5, 1779.
12. a) V. M. Neplyuev, T. A. Sinenko, and P. S. Pel'kis, Zh. Org. Khim., 1975, 11, 854;; J. Org. Chem. USSR, 1975, 11, 843; b) V. M. Neplyuev, Zh. Org. Khim., 1979, 15, 563;; J. Org. Chem. USSR, 1979, 15, 500.
13. a) Z. Tamura, Y. Iitaka, H. Tanabe, and S. Uchiyama, Chem. Pharm. Bull., 1970, 18, 2359; CrossRef b) S. Uchiyama, H. Tanabe, and Z. Tamura, Chem. Pharm. Bull., 1972, 20, 357; CrossRef c) Y. Iitaka, S. Uchiyama, and Z. Tamura, Chem. Pharm. Bull., 1972, 20, 1181; CrossRef d) S. Uchiyama and Z. Tamura, Chem. Pharm. Bull., 1975, 23, 1032; CrossRef e) S. Uchiyama and Z. Tamura, Yakugaku Zasshi, 1977, 97, 58 (Chem. Abstr., 1977, 86, 146011).
14. S. Araki, K. Yamamoto, M. Yagi, T. Inoue, H. Fukagawa, H. Hattori, H.Yamamura, M. Kawai, and Y. Butsugan, Eur. J. Org. Chem., 1998, 121. CrossRef
15. A. Awadallah, P. Rademacher, and R. Boese, J. Prakt. Chem./Chem.-Ztg., 1995, 337, 636.
16. a) A. V. Logvinov, I. N. Polyakova, and E. L. Golod, Zh. Obshch. Khim., 2009, 79, 1726;; Russ. J. Gen. Chem., 2009, 79, 2220; CrossRef b) V. V. Zarubaev, E. L. Golod, P. M. Anfimov, A. A. Shtro, V. V. Saraev, A. S. Gavrilov, A. V. Logvinov, and O. I. Kiselev, Bioorg. Med. Chem., 2010, 18, 839. CrossRef
17. A. Vollmar and A. Hassner, J. Heterocycl. Chem., 1974, 11, 491. CrossRef
18. S. Araki, T. Hirashita, and Y. Kakumu, PCT Int. Appl., WO 2008 056776; May 15, 2008 (Chem. Abstr., 2008, 148, 561916).
19. W. Bocian, J. Jaźwiński, W. Koźmiński, L. Stefaniak, and G. A. Webb, J. Chem. Soc., Perkin Trans. 2, 1994, 1327. CrossRef
20. For a review of this class of compounds, see: D. Moderhack, Heterocycles, 2008, 75, 1. CrossRef
21. S. Araki, M. Kuzuya, K. Hamada, M. Nogura, and N. Ohata, Org. Biomol. Chem., 2003, 1, 978. CrossRef
22. S. Araki, J. Mizuya, and Y. Butsugan, J. Chem. Soc., Perkin Trans. 1, 1985, 2439. CrossRef
23. S. Araki and Y. Butsugan, Chem. Ber., 1993, 126, 1157. CrossRef
24. S. Araki, T. Goto, and Y. Butsugan, Bull. Chem. Soc. Jpn., 1988, 61, 2977. CrossRef
25. A. V. Logvinov, V. V. Saraev, I. N. Polyakova, Yu. A. Strelenko, and E. L. Golod, Zh. Obshch. Khim., 2007, 77, 2041; Russ. J. Gen. Chem., 2007, 77, 2186. CrossRef
26. a) E. Lippmann, D. Reifegerste, and E. Kleinpeter, Z. Chem., 1973, 13, 134; b) E. Lippmann, D. Reifegerste, and E. Kleinpeter, Z. Chem., 1974, 14, 16; c) relative energies of 23 / 24 / 26 / Ac-A2 (R = Me): 0.00 / 7.18 / 17.57 / 12.78 kcal/mol (B3LYP-6/31G**, gas phase) (D. Moderhack, unpublished results).
27. S. Araki and Y. Butsugan, Chem. Lett., 1985, 1639. CrossRef
28. A. Gómez-Zavaglia, I. D. Reva, L. Frija, M. L. Cristiano, and R. Fausto, J. Phys. Chem. A, 2005, 109, 7967. CrossRef
29. R. A. Henry, W. G. Finnegan, and E. Lieber, J. Am. Chem. Soc., 1954, 76, 2894. CrossRef
30. S. V. Voitekhovich, P. N. Gaponik, A. S. Lyakov, and O. A. Ivashkevich, Tetrahedron, 2008, 64, 8721. CrossRef
31. S. Araki, H. Hattori, H. Yamamura, and M. Kawai, J. Heterocycl. Chem., 2000, 37, 1129. CrossRef
32. J. Jaźwiński, Polish J. Chem., 1999, 73, 1719.
33. J. Oku, S. Araki, and Y. Yamamoto, JP 2007 297561; Nov 15, 2007 (Chem. Abstr., 2007, 147, 523046).
34. S. Araki, K. Yamamoto, T. Inoue, K. Fujimoto, H. Yamamura, M. Kawai, Y. Butsugan, J. Zhou, E. Eichhorn, A. Rieker, and M. Huber, J. Chem. Soc., Perkin Trans. 2, 1999, 985. CrossRef
35. S. Araki, H. Hattori, K. Ogawa, M. Kuzuya, T. Inoue, H. Yamamura, and M. Kawai, J. Chem. Soc., Perkin Trans. 1, 2001, 2476. CrossRef
36. K. T. Potts, '1,3-Dipolar Cycloaddittion Chemistry: Mesoionic Ring Systems', Vol. 2, ed. by A. Padwa, Wiley, New York, 1984, pp. 1–82.
37. a) E. Bamberger and F. Kuhlemann, Ber. Dtsch. Chem. Ges., 1893, 26, 2978; CrossRef b) E. Bamberger and J. Müller, J. Prakt. Chem., 1901, 64, 199. CrossRef
38. F. A. Neugebauer, H. Fischer, and C. Krieger, Chem. Ber., 1979, 112, 2369. CrossRef
39. R. N. Hanley, W. D. Ollis, C. A. Ramsden, and I. S. Smith, J. Chem. Soc., Perkin Trans. 1, 1979, 744. CrossRef
40. a) S. Araki and Y. Butsugan, J. Chem. Soc., Chem. Commun., 1983, 789; CrossRef b) S. Araki and Y. Butsugan, J. Chem. Soc., Perkin Trans. 1, 1984, 2545. CrossRef
41. S. Araki and Y. Butsugan, Tetrahedron Lett., 1984, 25, 441. CrossRef
42. S. Araki, J. Mizuya, N. Aoyama, and Y. Butsugan, J. Chem. Soc., Perkin Trans. 1, 1995, 1989.
43. R. H. Lowack, 'Derivatisierung und Ringöffnung reduzierbarer Tetrazolium-Systeme', Dissertation, University of Erlangen-Nürnberg (Germany), 1990.
44. S. Vaidya, A. Upadhyay, S. K. Singh, T. Gupta, S. Tewary, S. K. Langley, J. P. S. Walsh, K. S. Murray, G. Rajaraman, and M. Shanmugam, Chem. Commun., 2015, 51, 3739. CrossRef
45. R. H. Lowack and R. Weiss, J. Am. Chem. Soc., 1990, 112, 333. CrossRef
46. M. O. Lozinskii and P. S. Pel'kis, Zh. Obshch. Khim., 1963, 33, 113; J. Gen. Chem. (USSR), 1963, 33, 106..
47. R. Fusco and R. Romani, Gazz. Chim. Ital., 1946, 76, 439.
48. S. Araki, S. Hirose, Y. Konishi, M. Nogara, and T. Hirashita, Beilstein J. Org. Chem., 2009, 5 (8), no pp. given (Chem. Abstr., 2009, 151, 381263).
49. a) S. Araki, N. Aoyama, and Y. Butsugan, Tetrahedron Lett., 1987, 28, 4289; CrossRef b) S. Araki, N. Aoyama, and Y. Butsugan, Bull. Chem. Soc. Jpn., 1989, 62, 1612. CrossRef
50. A. T. Hutton and H. M. N. H. Irving, J. Chem. Soc., Perkin Trans. 2, 1982, 1117. CrossRef
51. K. G. von Eschwege and J. C. Swarts, Polyhedron, 2010, 29, 1727. CrossRef
52. A. M. Kiwan and G. A. Wanas, J. Chem. Soc., Perkin Trans. 2, 1981, 1534. CrossRef
53. A. Taha and A. M. Kiwan, New J. Chem., 2001, 25, 502. CrossRef
54. F. Jian, P. Zhao, L. Zhang, and Y. Hou, J. Org. Chem., 2005, 70, 8322. – For a critical view of structure (B2a'), see ref.
55. P. S. Zhao, F. F. Jian, R. R. Zhuang, and J. Zheng, Asian J. Chem., 2007, 19, 4258; – The material prepared by this method was earlier viewed as a perhydro derivative; the same applies to the substance obtained from dithizone: P. S. Zhao, F. F. Jian, H. L. Xiao, and Y. X. Hou, Bull. Korean Chem. Soc., 2004, 25, 1935 (Chem. Abstr., 2005, 142, 481990).
56. T. V. Koksharova, Zh. Strukt. Khim., 2004, 45, 361; J. Struct. Chem., 2004, 45, 344. CrossRef
57. a) T. Irshaidat, J. Phys. Org. Chem., 2010, 23, 67; b) K. G. von Eschwege, J. Conradie, and A. Kuhn, J. Phys. Chem. A, 2011, 115, 1463. CrossRef
58. S. Araki, T. Goto, and Y. Butsugan, Bull. Chem. Soc. Jpn., 1988, 61, 2979. CrossRef
59. R. Luboradzki, W. Koźmiński, and L. Stefaniak, J. Crystallogr. Spectrosc. Res., 1993, 23, 133. CrossRef
60. a) K. T. Potts, A. J. Elliott, G. R. Titus, D. Al-Hilal, P. F. Lindley, G. V. Boyd, and T. Norris, J. Chem. Soc., Perkin Trans. 1, 1981, 2692; CrossRef b) K. T. Potts, A. J. Elliott, G. R. Titus, D. Al-Hilal, P. F. Lindley, G. V. Boyd, and T. Norris, Acta Cryst. B, 1982, 38, 682. CrossRef
61. W. Koźmiński, J. Jaźwiński, and L. Stefaniak, Magn. Reson. Chem., 1993, 31, 200. CrossRef
62. E. Bamberger, R. Padova, and E. Ormerod, Liebigs Ann. Chem., 1926, 446, 260. CrossRef
63. R. Weiss and R. H. Lowack, Angew. Chem., 1991, 103, 1183; Angew. Chem. Int. Ed., 1991, 30, 1162. CrossRef
64. R. Luboradzki, J. Lipkowski, W. Koźmiński, and L. Stefaniak, J. Chem. Crystallogr., 1995, 25, 29. CrossRef
65. F. A. Neugebauer and H. Fischer, Chem. Ber., 1980, 113, 1226. CrossRef
66. Three conformers of 85 showing the PhN=N groups s-trans,s-trans, s-cis,s-trans or s-cis,s-cis relative to the C=C bond (with the N=O group kept s-trans throughout) were calculated (B3LYP-6/31G**, gas phase) (D. Moderhack, unpublished results).
67. W. Koźmiński, J. Jaźwiński, and L. Stefaniak, J. Mol. Struct., 1993, 295, 15. CrossRef
68. J. Jaźwiński, W. Koźmiński, L. Stefaniak, and G. A. Webb, Spectroscopy, 1994, 12, 21. CrossRef
69. K. G. von Eschwege and A. Muller, Acta Cryst. E, 2009, 65, o2. CrossRef
70. K. G. von Eschwege and A. Muller, Acta Cryst. E, 2009, 65, o1864.
71. L. Stefaniak and J. Jaźwiński, Khim. Geterotsikl. Soedin., 1995, 1180; L. Stefaniak and J. Jaźwiński, Chem. Heterocycl. Compd., 1995, 31, 1027. CrossRef
72. J. Jaźwiński, Bull. Polish Acad. Sci. Chem., 1998, 46, 79.
73. J. Jaźwiński, Bull. Polish Acad. Sci. Chem., 2000, 48, 35.
74. W. Bocian, J. Jaźwiński, W. Koźmiński, L. Stefaniak, and G. A. Webb, Magn. Reson. Chem., 1994, 32, 284. CrossRef
75. A. Barszczewicz, M. Jaszuński, and L. Stefaniak, Chem. Phys. Lett., 1991, 186, 313. CrossRef
76. J. W. Wiench, L. Stefaniak, A. Tabaszewska, and G. A. Webb, Electr. J. Theor. Chem., 1997, 2, 71. CrossRef
77. W. Koźmiński, J. Jaźwiński, L. Stefaniak, and G. A. Webb, Magn. Res. Chem., 1994, 32, 746. CrossRef
78. W. Koźmiński, J. Jaźwiński, L. Stefaniak, and G. A. Webb, Magn. Res. Chem., 1990, 28, 1027. CrossRef
79. a) P. B. Talukdar, S. K. Sengupta, and A. K. Datta, Indian J. Chem., 1971, 9, 1018; b) P. B. Talukdar, S. K. Sengupta, A. K. Datta, and A. Chakravorty, Indian J. Chem., 1973, 11, 611.
80. J. Fabian, Izv. Khim., 1987, 20, 562 (Chem. Abstr., 1988, 109, 200080).
81. G. Guisado-Barrios, J. Bouffard, B. Donnadieu, and G. Bertrand, Angew. Chem., 2010, 122, 4869; CrossRef Angew. Chem. Int. Ed., 2010, 49, 4759; CrossRef – The authors of this paper (on 1H-1,2,3-triazol-5-ylidenes) as well as those of reference2b were unaware of the original coinage5 of that term; for a later recognition, see however: G. Ung, D. Mendoza-Espinosa, and G. Bertrand, Chem. Commun., 2012, 48, 7088.
82. W. P. Norris and R. A. Henry, Tetrahedron Lett., 1965, 1213. CrossRef
83. S. Araki, K. Yokoi, R. Sato, T. Hirashita, and J.-I. Setsune, J. Heterocycl. Chem., 2009, 46, 164. CrossRef
84. H. Balli and F. Kersting, Liebigs Ann. Chem., 1963, 663, 96; CrossRef D. Schelz and H. Balli, Helv. Chim. Acta, 1970, 53, 1913. CrossRef
85. D. Moderhack, Heterocycles, 2013, 87, 493; CrossRef – The formation of A5v is an addendum to p. 518.
86. L.-A. Schaper, X. Wei, P. J. Altmann, K. Öfele, A. Pöthig, M. Drees, J. Mink, E. Herdtweck, B. Bechlars, W. A. Herrmann, and F. E. Kühn, Inorg. Chem., 2013, 52, 7031. CrossRef
87. J. Müller, K. Öfele, and G. Krebs, J. Organomet. Chem., 1974, 82, 383. CrossRef
88. J. C. Bernhammer, G. Frison, and H. V. Huynh, Chem. Eur. J., 2013, 19, 12892. CrossRef
89. R. Jothibasu and H. V. Huynh, Organometallics, 2009, 28, 2505. CrossRef
90. R. A. Olofson, W. R. Thompson, and J. S. Michelman, J. Am. Chem. Soc., 1964, 86, 1865. CrossRef
91. According to a model study with the N-unsubstituted carbene (D), ring opening at the N(2)–N(3) bond led to nitrilimine-type species that were found distinctly higher in energy than 104 (H instead of Ar).