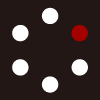
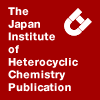
HETEROCYCLES
An International Journal for Reviews and Communications in Heterocyclic ChemistryWeb Edition ISSN: 1881-0942
Published online by The Japan Institute of Heterocyclic Chemistry
e-Journal
Full Text HTML
Received, 6th November, 2015, Accepted, 11th December, 2015, Published online, 18th December, 2015.
DOI: 10.3987/COM-15-13360
■ Facile and Efficient Access to Tetrahydrobenzo[b]pyrans Catalyzed by N,N-Dimethylbenzylamine
Hamzeh Kiyani* and Mozhgan Sadat Jalali
School of Chemistry, Damghan University, Cheshmeh Ali Road, Daneshgah square, Damghan 36715-364, Iran
Abstract
The N,N-dimethylbenzylamine (DMBA) has been used as an efficient, inexpensive, and commercially available organocatalyst for the one-pot, three-component synthesis of tetrahydrobenzo[b]pyrans in good to high yields. It was found that the one-pot Knoevenagel-Michael-Thorpe-Ziegler cyclization sequence of dimedone, malononitrile/ethyl cyanoacetate, and various aldehydes was efficiently implemented in ethanol at 45 °C. This procedure offers attractive several features: mild reaction conditions, use of ethanol as a green solvent, reusability the reaction media, shorter reaction times, and the ease of the work-up.INTRODUCTION
The multicomponent reactions (MCRs) are highly efficient tools in modern organic synthesis chemistry for the construction of several bonds in a one-step operation, which leads to biologically and pharmaceutically active compounds. In this category of reactions, the desired products are formed by association more than three chemical species. MCRs have many benefits, such as high atom economy, the avoidance of time-consuming, simplicity in processing, high efficacy in constructing libraries of heterocyclic molecules from simple and readily available materials without isolation of any intermediate, minimal waste generation, shorter reaction times, the avoidance of changing the conditions during the implementation of the reaction, energy saving, the possible structural variations, high yields and the simplicity of a one-pot procedure.1-5 The tetrahydrobenzo[b]pyrans are unique classes of 4H-pyran-annulated heterocyclic frameworks represent a “drug-like” structural motif with a broad spectrum of applications in organic synthesis and medicinal chemistry. A series of these six-membered oxygen-containing heterocyclic compounds exhibited a wide range of biological and pharmaceutical activities including, anticancer,6 antibacterial,7 cytotoxicity,8 and the human excitatory amino acid transporter subtype1 (EAAT1) inhibitory activity.9 They can also be used as cognitive enhancers for the treatment of schizophrenia, Alzheimer’s disease, amyotrophic lateral sclerosis, Huntington’s disease, Parkinson’s disease, and Down’s syndrome.10 Some exemplary biologically active tetrahydrobenzo[b]pyran-containing compounds are shown in Figure 1.
In recent years, considering the aforementioned properties, it is expected that the multicomponent synthesis of tetrahydrobenzo[b]pyrans and their derivatives has received much attention from researchers interested in organic synthetic chemistry. To date, various synthetic approaches have been reported in the literature for the preparation of these eye-catching heterocyles by one-pot, three-component annulation (3CA) of different aldehydes, malononitrile and 5,5-dimethylcyclohexane-1,3-dione (dimedone) using a variety of catalysts such as nanoparticles,11,12 ionic liquids,13,14 carbohydrates,15 solid acids,16-21 solid bases,22,23 organocatalysts,24-28 and iodine/DMSO system.29 Microwave30,41 and ultrasound32 irradiations, ball milling,33 and electrosynthesis34 techniques have also been used to the promoting synthesis of tetrahydrobenzo[b]pyrans. Furthermore, uncatalyzed versions in aqueous media,35 and 2,2,2-trifluoroethanol (TFE)36 have been utilized for this 3CA. Substituted tetrahydrobenzo[b]pyran-containing compounds have also been obtained using the reaction of 1,3-oxazines with dimedone and malononitrile in refluxing acetonitrile:acetic acid (10:1).37 We have recently used the potassium phthalimide38-40 and potassium hydrogen phthalate41 as catalysts for the synthesis of diverse 4H-pyran-annulated molecules.
Each of above-mentioned procedures has their own merits. However, some of the methods required the use of organic solvents such as DMSO and TFE, reflux conditions, relatively low yields, tedious work-up procedures, expensive catalysts, special apparatus (ultrasound, microwave, and ball milling) or electrosynthesis system. In consequence, using an inexpensive, mild, and efficient catalyst leading to the tetrahydrobenzo[b]pyran scaffold via a simple MCR remains an issue of interest.
The N,N-dimethylbenzylamine (DMBA) has been broadly utilized as a useful ortho-metalated ligand in organometallic chemistry for the synthesis of a number of transition metallocycles.42,43 This tert-amine was also used as the directing group to ortho-functionalizations such as, ortho arylation,44 ortho olefination,45 borylation of arenes,46 ortho carbonylation,47 and ortho-silylation.44 It is noteworthy to mention that the N,N-dimethylaminomethyl group present in the functionalized N,N-dimethylbenzylamines can be easily converted to various and useful functional groups including, amide,48 aldehyde,49 alkene,50 as well as chloromethyl substituent.51 In addition, DMBA has been employed as a promoter for the process production of heat-cured epoxy systems52 and polyurethanes,53 as well as the reaction of oils with maleic anhydride to functionalize the tri-glycerides.54
As above-mentioned, there are a plethora of catalysts about the synthesis of pyran-annulated heterocycles, but we have not seen any publication about catalytic effect of DMBA on the preparation of these heterocyclic scaffolds and this work is the first report on the use of DMBA for the preparation of them (Scheme 1).
RESULTS AND DISCUSSION
To find out the best experimental conditions for the preparation of tetrahydrobenzo[b]pyrans, a model reaction, involving the 3-component annulation (3CA) between dimedone (1), 3-nitrobenzaldehyde (2d) and malononitrile (3a) was selected (Table 1).
The initial studies were carried out on the model reaction using 25 mol% DMBA as the catalyst in benign solvent ethanol (EtOH) at various temperatures (Table 1, entries 1-8). The model reaction was implemented in the presence of 25 mol% loading of DMBA catalyst and 2-amino-7,7-dimethyl- 4-(3-nitrophenyl)-5-oxo-5,6,7,8-tetrahydro-4H-chromene-3-carbonitrile (4d) was isolated in good yield after 54 min at rt (Table 1, entry 1). In this stage, formation of the desired product (4d) revealed that the reaction proceeded efficiently by adding the catalyst. The chemical yields were improved by increasing the reaction temperatures from rt to 30, 40 and 45 °C, and the reaction times were significantly shortened (Table 1, entries 2-4). The reaction was also carried out at temperatures above 45 °C and did not achieve any improvement in the yields and reaction times (Table 1, entries 5-8). The best results were obtained when the model reaction was conducted at 45 °C, and hence this temperature was chosen as the optimum temperature. Increasing the amount of the catalyst beyond 25 mol% did not increase the yield noticeably (Table 1, entry 9). We investigated the model reaction without any added the catalyst at rt and optimum temperature; the product (4d) was formed in low yield even after prolonged reaction times (Table 1, entries 10 and 11) indicating that the catalyst is necessary for the 3CA. Next, the amount of DMBA was
decreased and it was observed that lower yield of the corresponding product (4d) was obtained and progress of the reaction was slow (Table 1, entries 12-15). Hence, the efficacy of the reaction is affected mainly by the amount of DMBA catalyst. It was concluded that 25 mol% DMBA loading and 45 °C was the best catalyst amount and reaction temperature, respectively. Under these optimized conditions, the efficacy of the solvent system was also examined using various solvents such as n-hexane, dichloromethane (CH2Cl2), ethyl acetate (EtOAc), methanol (MeOH) and water (H2O) (Table 1, entries 16-20). Under these conditions EtOH was again found to be superior to the other solvents. Due to the
shorter reaction time and high yield the optimum conditions for further reactions were selected as EtOH solvent with 25 mol% of DMBA and heating to 45 °C.
Using with optimized reaction conditions in hand, the reactions of various aryl, heteroaryl, and aliphatic aldehydes (2a-j) as well as the cyano substrates (3a-b) with dimedone (1) were tested to investigate the generality of the reaction. The results are summarized in Table 2. All aryl aldehydes substituted with either electron-withdrawing (Table 2, entry 2-7) or electron-donating (Table 2, entries 8-14) functional groups and heteroaryl aldehydes (Table 2, entries 15 and 16) reacted smoothly with dimedone (1) and malononitrile (3a) to produce the desired annulated products in good to excellent yields. The annulation process with α,β-unsaturated aldehydes such as cinnamaldehyde (2q) and butyraldehyde (2r) as an aliphatic aldehyde was also proceeded smoothly under the same optimized reaction conditions and the corresponding products (4q-r) were isolated in good yields (Table 2, entries 17 and 18). In these two cases, lower yields than that of the other aldehydes is probably owing to the high electron density of these two aldehydes.
Based on the results indicated in Table 2, the electronic nature of the substituents on the benzaldehydes did not significantly influence the chemical yields of the corresponding annulated products; however the reaction times are affected. When aromatic aldehydes with electron-withdrawing groups (2b-f) as substrates were used, the reaction time was shorter and the reaction proceeded well at faster rate compared with aromatic aldehydes with electron-donating groups (2h-n). Substitutions at the ortho positions of the benzaldehydes resulted in a slight decrease in the yield of the reaction in addition to a lower reaction rate (Table 2, entries 2, 7 and 22). It was observed that when the malononitrile (3a) replaced with a less reactive methylene compound such as ethyl cyanoacetate (3b), the corresponding products (4s-y) were isolated in small lower yields and required longer reaction times (Table 2, entries 19-25), which may be ascribed to the competency of the cyanide group in stabilizing the reaction intermediates compared to the ester group. In all cases, the reaction media can be reused for further reactions. Reusability of the reaction media was carried out using the model reaction for the synthesis of 4d. After completion of the reaction, the resulting solid product was collected by filtration. To the filtrate that containing DMBA, dimedone (1), 3-nitrobenzaldehyde (2d), and malononitrile (3a) were added in the same molar ratio devoid of extra load of catalyst. The reaction mixture was stirred at 45 °C for the required time. It showed a slight decrease of yield in first three runs (92%, 84%, and 80%), while in fourth run the yield dropped to 45%.
Based on the proposed mechanisms in the literature,27 a reasonable mechanism for the DMBA-catalyzed one-pot, three-component synthesis of the corresponding pyran-annulated heterocycles is depicted in Scheme 2. Probably, the malonate carbanions A were formed via deprotonation of the active methylene nitriles (3a-b), which is likely; facilitate the Knoevenagel condensation between these carbanions and aldehydes (2) to afford the arylidene nitrile intermediates D (Knoevenagel adducts). On the other hand, the role of DMBA in aiding enol-keto tautomerism in the C―H enolizable compounds (1 and 5) to E is also envisioned, which undergoes Michael-type addition with the Knoevenagel adducts D to give intermediates F, which is protonated to in-situ yield ketenimine intermediates G (Michael adducts). Furthermore, the intramolecular O-attack heterocyclization (Thorpe-Ziegler type reaction) of H results in the give intermediates I, followed protonation afford the final heterocyclic products 4.
A comparative study on the catalytic performance of DMBA with other reported catalysts for the one-pot, three-component synthesis of 4d as typical example are shown in Table 3. The present method is better or comparable with others in terms of the yields and reaction times.
EXPERIMENTAL
All the reagents were obtained from commercial sources and used without further purification. Melting points were measured on a Buchi 510 melting point apparatus and are uncorrected. 1H NMR and 13C NMR spectra were recorded at ambient temperature on a BRUKER AVANCE DRX-400 and 500 MHz spectrometer using CDCl3 or DMSO-d6 as the solvent. The purity of synthesized compounds as well as a the development of the reactions was monitored by thin layer chromatography (TLC) analysis on Merck pre-coated silica gel 60 F254 aluminum sheets, visualized by UV light. Elemental microanalyses were performed on an Elementar Vario EL III analyzer. All of the targeted products are reported in the literature and are characterized by comparison of their spectral and physical data on the basis of literature descriptions.
General Procedure for the synthesis of tetrahydrbenzo[b]pyrans (4a-y). To a magnetically stirring mixture of aldehyde (2, 1 mmol), dimedone (1, 1 mmol), malononitrile/ethyl cyanoacetate (3, 1 mmol) in EtOH (5 mL), DMBA (25 mol%) was added. The mixture was heated at 45 °C for the required time indicated in Table 2. The progress of the reaction was monitored by TLC analysis. After completion of the reaction, the reaction mixture was gradually cooled to room temperature and the resulting precipitates were collected by filtration, washed with cold EtOH and air-dried to give the pure corresponding products. The filtrate containing DMBA was used as such for exploring the reusability of the catalyst. If necessary, the solid products can be recrystallized from hot EtOH. The identity of the known products was confirmed by comparison of their spectroscopic data and physical properties with those described in the respective literature. Spectral data for 4a, 4d and 4o were as follows:
2-Amino-7,7-dimethyl-5-oxo-4-phenyl-5,6,7,8-tetrahydro-4H-chromene-3-carbonitrile (4a). IR (KBr, cm-1): ν = 3385, 3315, 2971, 2195, 1670, 1372, 1215; 1H NMR (400 MHz, CDCl3): δ = 1.08 (s, 3H), 1.15 (s, 3H), 2.22-2.30 (m, 2H), 2.49 (m, 2H), 4.44 (s, 1H), 4.59 (s, 2H), 7.22-7.35 (m, 5H); 13C NMR (100 MHz, CDCl3): δ = 28.4, 29.2, 32.1, 35.8, 41.4, 51.1, 63.8, 114.3, 119.8, 127.5, 127.9, 129.1, 143.8, 158.1, 162.3, 199.5. Anal. Calcd for C18H18N2O2: C, 73.45; H, 6.16; N, 9.52. Found: C, 73.42; H, 6.19; N, 9.50.
2-Amino-7,7-dimethyl-4-(3-nitrophenyl)-5-oxo-5,6,7,8-tetrahydro-4H-chromene-3-carbonitrile (4d). IR (KBr, cm-1): ν = 3361, 3340, 2945, 2208, 1675, 1655, 1520, 1330, 1195; 1H NMR (500 MHz, DMSO-d6): δ = 1.09 (s, 3H), 1.16 (s, 3H), 2.27 (m, 2H), 2.54 (m, 2H), 4.56 (s, 1H), 4.79 (s, 2H), 7.52 (t, J = 7.9 Hz, 1H), 7.71 (d, J = 7.7 Hz, 1H), 8.07-8.13 (m, 2H). Anal. Calcd for C18H17N3O4 (%): C, 63.71; H, 5.05; N, 12.38. Found: C, 63.74; H, 5.08; N.
2-Amino-4-(furan-2-yl)-7,7-dimethyl-5-oxo-5,6,7,8-tetrahydro-4H-chromene-3-carbonitrile (4o). IR (KBr, cm-1): ν = 3352, 3205, 2940, 2208, 1680, 1650, 1195; 1H NMR (400 MHz, DMSO-d6): δ = 1.01 (s, 3H), 1.05 (s, 3H), 2.20 (m, 2H), 2.48 (m, 2H), 4.35 (s, 1H), 6.07 (d, J = 3.9 Hz, 1H), 6.31 (dd, J = 3.9, 1.8 Hz, 1H), 7.06 (s, 2H), 7.50 (d, J = 3.9 Hz, 1H); 13C NMR (100 MHz, DMSO-d6): δ = 27.3, 29.5, 31.2, 32.7, 50.9, 58.8, 114.0, 120.5, 124.6, 125.2, 127.8, 150.3, 159.8, 163.5, 196.4. Anal. Calcd for C16H16N2O3 (%): C, 67.59; H, 5.67; N, 9.85. Found: C, 67.77; H, 5.66; N, 9.83.
ACKNOWLEDGEMENTS
The authors are grateful to the Research Council of Damghan University.
References
1. A. Dömling, W. Wang, and K. Wang, Chem. Rev., 2012, 112, 3083. CrossRef
2. I. Ugi, Pure Appl. Chem., 2001, 73, 187. CrossRef
3. A. Domling and I. Ugi, Angew. Chem. Int. Ed., 2000, 39, 3168. CrossRef
4. Z. Q. Liu, Curr. Org. Synth., 2015, 12, 20. CrossRef
5. J. Zhu, Q. Wang, and M. X. Wang, Multicomponent Reactions in Organic Synthesis, Wiley-VCH, Weinheim, 2015.
6. F. M. Abdelrazeka, P. Metza, and E. K. Farrag, Arch. Pharm. Pharm. Med. Chem., 2004, 337, 482. CrossRef
7. D. Kumar, V. B. Reddy, S. Sharad, U. Dube, and S. Kapur, Eur. J. Med. Chem., 2009, 44, 3805. CrossRef
8. R. M. Mohareb, N. S. Abbas, and M. A. Abdelaziz, Steroids, 2014, 86, 45. CrossRef
9. T. H. V. Huynh, I. Shim, H. Bohr, B. Abrahamsen, B. Nielsen, A. A. Jensen, and L. Bunch, J. Med. Chem., 2012, 55, 5403. CrossRef
10. G. Brahmachari, Green Synthetic Approaches for Biologically Relevant Heterocycles, Elsevier, Amsterdam, Netherlands, 2015, ch 8. CrossRef
11. S. Banerjee and A. Saha, New J. Chem., 2013, 37, 4170. CrossRef
12. P. L. Anandgaonker, S. Jadhav, S. T. Gaikwad, and A. S. Rajbhoj, J. Clust. Sci., 2014, 25, 483.
13. H. Hu, F. Qiu, A. Ying, J. Yang, and H. Meng, Int. J. Mol. Sci., 2014, 15, 6897. CrossRef
14. D. Fang, H. B. Zhang, and Z. L. Liu, J. Heterocycl. Chem., 2010, 47, 63.
15. F. N. Sadeh, M. T. Maghsoodlou, N. Hazeri, and M. Kangani, Res. Chem. Intermed., 2014, 41, 5907. CrossRef
16. M. Moghaddas and A. Davoodnia, Res. Chem. Intermed., 2014, 41, 4373. CrossRef
17. B. Boumoud, A. Debbache, T. Boumoud, R. Boulcina, and A. Debache, Lett. Org. Chem., 2014, 11, 475. CrossRef
18. H. A. Oskooie, M. M. Heravi, N. Karimi, and M. E. Zadeh, Synth. Commun., 2011, 41, 436. CrossRef
19. G. Sabitha, K. Arundhathi, K. Sudhakar, B. S. Sastry, and J. S. Yadav, Synth. Commun., 2009, 39, 433. CrossRef
20. M. Abdollahi-Alibeik and N. Sadeghi-Vasafi, Reac. Kinet. Mech. Cat., 2014, 112, 511. CrossRef
21. M. Abdollahi-Alibeik and F. Nezampour, Reac. Kinet. Mech. Cat., 2013, 108, 213. CrossRef
22. D. M. Pore, K. A. Undale, B. B. Dongare, and U. V. Desai, Catal. Lett., 2009, 132, 104. CrossRef
23. V. M. Joshi, R. L. Magar, P. B. Throat, S. U. Tekale, B. R. Patil, M. P. Kale, and R. P. Pawar, Chin. Chem. Lett., 2014, 25, 455. CrossRef
24. G. Brahmachari and B. Banerjee, ACS Sustain. Chem. Eng., 2014, 2, 411. CrossRef
25. J. Albadi, M. Fadaeian, and F. Akbari Balout-Bangan, Iran. J. Org. Chem., 2013, 5, 1089.
26. S. S. Mansoor, K. Logaiya, K. Aswin, and P. N. Sudhan, J. Taibah Univ. Sci., 2015, 9, 213.
27. D. Tahmassebi, J. A. Bryson, and S. I. Binz, Synth. Commun., 2011, 41, 2701. CrossRef
28. N. Montazeri, T. Noghani, M. Ghorchibeigy, and R. Zoghi, J. Chem., 2014, Article ID 596171, 5 pages, 2014, doi:10.1155/2014/596171. CrossRef
29. R. S. Bhosale, C. V. Magar, K. S. Solanke, S. B. Mane, S. S. Choudhary, and R. P. Pawar, Synth. Commun., 2007, 37, 4353. CrossRef
30. H. Hagiwara, A. Numamae, K. Isobe, T. Hoshi, and T. Suzuki, Heterocycles, 2006, 68, 889. CrossRef
31. N. M. Abd El-Rahman, A. A. El-Kateb, and M. F. Mady, Synth. Commun., 2007, 37, 3961. CrossRef
32. J.-T. Li, W.-Z. Xu, L.-C. Yang, and T.-S. Li, Synth. Commun., 2004, 34, 4565. CrossRef
33. O. H. Qareaghaj, S. Mashkouri, M. R. Naimi-Jamal, and G. Kaupp, RSC Adv., 2014, 4, 48191.
34. L. Fotouhi, M. M. Heravi, A. Fatehi, and K. Bakhtiari, Tetrahedron Lett., 2007, 48, 5379. CrossRef
35. S. B. Bandgar, B. P. Bandgar, B. L. Korbad, J. V. Totre, and S. Patil, Aust. J. Chem., 2007, 60, 305. CrossRef
36. S. Khaksar, A. Rouhollahpour, and S. Mohammadzadeh Talesh, J. Fluorine Chem., 2012, 141, 11. CrossRef
37. K. Singh, J. Singh, and H. Singh, Tetrahedron, 1996, 52, 14273. CrossRef
38. H. Kiyani and F. Ghorbani, J. Saudi Chem. Soc., 2014, 18, 689.
39. H. Kiyani and F. Ghorbani, Chem. Pap., 2014, 68, 1104. CrossRef
40. H. Kiyani and F. Ghorbani, Res. Chem. Intermed., 2015, 41, 4031. CrossRef
41. H. Kiyani and F. Ghorbani, Res. Chem. Intermed., 2015, 41, 7847. CrossRef
42. S. Kolay, N. Ghavale, A. Wadawale, D. Das, and V. K. Jain, Phosphorus, Sulfur Silicon Relat. Elem., 2013, 188, 1449. CrossRef
43. A. R. Petrov, O. Thomas, K. Harms, K. A. Rufanov, and J. Sundermeyer, J. Organomet. Chem., 2010, 695, 2738. CrossRef
44. R. Feng, J. Yao, Z. Liang, Z. Liu, and Y. Zhang, J. Org. Chem., 2013, 78, 3688. CrossRef
45. G. Cai, Y. Fu, Y. Li, X. Wan, and Z. Shi, J. Am. Chem. Soc., 2007, 129, 7666. CrossRef
46. L. V. A. Hale, K. A. McGarry, M. A. Ringgold, and T. B. Clark, Organometallics, 2015, 34, 51. CrossRef
47. H. Li, G. X. Cai, and Z. J. Shi, Dalton Trans., 2010, 39, 10442. CrossRef
48. W. P. Mai, G. Song, J. W. Yuan, L. R. Yang, G. C. Sun, Y. M. Xiao, P. Mao, and L. B. Qu, RSC Adv., 2013, 3, 3869.
49. J. L. Gong, X. Qi, D. Wei, J. B. Feng, and X. F. Wu, Org. Biomol. Chem., 2014, 12, 7486. CrossRef
50. C. R. Sanders and J. H. Prestegard, J. Am. Chem. Soc., 1991, 113, 1987. CrossRef
51. F. Stiemke, M. Gjikaj, and D. E. Kaufmann, J. Organomet. Chem., 2009, 694, 5. CrossRef
52. B. Stahlbom, B. Akesson, and B. Jonsson, Int. Arch. Occup. Environ. Health, 1997, 70, 393. CrossRef
53. D. Braun, H. Cherdron, M. Rehahn, H. Ritter, and B. Voit, Polymer Synthesis: Theory and Practice Fundamentals, Methods, Experiments, Fifth Edition, Springer-Verlag Berlin Heidelberg, 2013. CrossRef
54. S. N. Khot, J. J. Lascala, E. Can, S. S. Morye, G. I. Williams, G. R. Palmese, S. H. Kusefoglu, and R. P. Wool, J. Appl. Polym. Sci., 2001, 82, 703. CrossRef