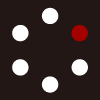
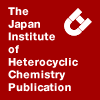
HETEROCYCLES
An International Journal for Reviews and Communications in Heterocyclic ChemistryWeb Edition ISSN: 1881-0942
Published online by The Japan Institute of Heterocyclic Chemistry
e-Journal
Full Text HTML
Received, 9th November, 2015, Accepted, 26th November, 2015, Published online, 8th December, 2015.
DOI: 10.3987/COM-15-13363
■ Antioxidant Effects of the Hydroxy Groups in the Simple Phenolic Carbazoles
Yuhzo Hieda, Noriyuki Hatae, Makoto Anraku, Nobuyasu Matsuura, Kazuhide Uemura, Satoshi Hibino, Tominari Choshi,* Hisao Tomida, Osamu Hori, and Haruto Fujioka*
Faculty of Pharmacy and Pharmaceutical Sciences, Fukuyama University, Fukuyama, Hiroshima 729-0292, Japan
Abstract
Antioxidant activities of the simple phenolic carbazoles 5-11 were evaluated by 2,2-diphenyl-1-picrylhydrazyl and 2,2’-azinobis-(3-ethylbenzthiazoline-6-sulfonate)+ radical scavenging assays. The simple phenolic carbazoles 5-7, 9, and 11 exhibited stronger antioxidant activities than α-tocopherol, and similar antioxidant activities as phenolic carbazole alkaloids carazostatin (1), and carbazomadurins A (3) and B (4). Bond dissociation energies and highest occupied molecule orbital energy levels of a series of phenolic carbazoles including phenolic carbazole alkaloids were calculated. The reducing ability of the phenolic carbazole core could be important role for the antioxidant activity of carbazole alkaloids 1, 3, and 4.Reactive oxygen species (ROS) are highly reactive small substances with an important role in the initiation of various diseases such as carcinogenesis, drug-associated toxicity, inflammation, and atherogenesis as well as aging in aerobic organisms. Natural antioxidants, including ascorbic acid, α-tocopherol (VE), ubiquinol, uric acid, polyphenols and their analogs have been intensive investigation for clinical applications.1 Edaravone (3-methyl-N-phenylpyrazolin-5-one) scavenges free radicals and protects the brain against ischemic lesions after stroke, thus improving the clinical outcomes for patients with acute ischemic damage.2 Synthetic and/or medicinal chemists are interested in obtaining novel antioxidant substances as an important approach to treating oxidative stress-related diseases.1,2
Carbazole alkaloids are a well-known class of natural products possessing a benzo[b]indole ring system.3 These heterocyclic natural products have a wide variety of bioactivities, such as antibacterial, antibiotic,4 antimalarial, antitumor,5,6 cardiotonic,7 anti-inflammatory,8 free radical scavenging and neuronal cell-protecting9-13 activities. Due to their interesting biologic effects, carbazole alkaloids and their related compounds constitute an important class of natural products.
In 1989, the 3-hydroxycarbazole alkaloid carazostatin (1) was isolated from Streptomyces chromofuscus as a free radical-scavenging substance.9a This alkaloid exhibits strong inhibitory activity against free radical–induced lipid peroxidation and stronger antioxidant activity in liposomal membranes than VE.9b Furthermore, the antioxidant activity of 1 and 2-hydoxycarbazole (8) was assessed in the oxidation of methyl linolate induced by aza radical initiator.10 Few years later, 3,8-dihydroxycarbazoles carbazomadurins A (3) and B (4) were isolated from Actinomadula madurae 2808-SV-1.11 The antioxidative activity of these alkaloids was evaluated based on their inhibition of L-glutamate toxicity in N18-RE-195 cells. In addition, the first example of carbazole-3,4-quinone alkaloid carquinostatin A was isolated in 1993 from Streptomyces exfoliates 2419-SVT2.12a This natural product is a potent neuronal cell-protecting substance and exhibits free radical scavenging activity.12b On the other hand, 3-hydroxycarbazole lipocarbazoles A1-A4, closely related to carazostatin, were found in Tsukamurella pseudospumae Acta 1857.13 The antioxidant activity of lipocarbazole A3 was reported to be more active than ascorbic acid in a 2,2-diphenyl-1-picrylhydrazyl (DPPH) radical scavenging assay.13
These 3-hydroxycarbazole, 3,8-dihydroxycarbazole and carbazole-3,4-quinone alkaloids were independently evaluated in a bioassay system during isolation, but have not yet been evaluated comprehensively. We recently reported that 3-hydroxycarbazole carazostatin (1), 3,8-dihydroxycarbazole alkaloids carbazomadurins A (3) and B (4), and related carbazoles, except carbazole-3,4-quinone alkaloid carquinostatin A, exhibit strong antioxidant effects based on a comprehensive evaluation in several assay systems using propyl galate (PG), VE and edaravone as standards.14 Recently, the radical scavenging activities by antioxidants were rationalized based on their chemical properties using quantum chemical calculations. The scavenging activities of free radicals by lipocarbazoles were well evaluated by the O-H and N-H bond dissociation enthalpies.15 The glutathione peroxidase-like antioxidant activities of aliphatic selenides were consistent with their highest occupied molecule orbital (HOMO) energy levels (EHOMO).16
In the present paper, we describe the relationship between the calculated structure and antioxidant activity of simple oxygenated carbazoles 5-11 in comparison with carazostatin (1) and carbazomadurins A (3) and B (4) (Figure 1).
To evaluate the antioxidant activities of 3-hydroxycarbazole alkaloid, carazostatin (1) was prepared by an allene-mediated electrocyclic reaction involving the indole 2,3-bond according to the previously reported procedure (Scheme 1).17 Treatment of the indole-2-propargyl ether 14, derived from 3-iodoindole-2-carbaldehyde 12 in three steps, with t-BuOK afforded tri-substituted carbazole 15. Cleavage of the phenylsulfonyl group of 15 with NaOH, followed by treatment of TMS-I afforded the 1-hydroxy-3-ethoxycarbazole 6. Treatment of 6 with Tf2O gave the O-triflate 17, which was subjected to a Pd-catalyzed cross-coupling reaction with 9-hexyl-9-BBN to give the 1-hexylcarbazole 2. Subsequent treatment of 2 with BBr3 provided carazostatin (1). In addition, 1,3-dihydroxycarbazole 5 was prepared in two steps from 17. Carazostatin (1), 1-hexyl-3-ethoxycarbazole 2, 1,3-dihydroxycarbazole 5, and 1-hydroxy-3-ethoxycarbazole 6 were used to evaluate antioxidant activities. 3,8-Dihydroxycarbazole alkaloids carbazomadurins A (3) and B (4) were prepared according to our reported procedure and used in radical scavenging assays.18
Simple hydroxycarbazoles 7, 9 and 11 were prepared by the reported procedures19 as follows (Scheme 2). Buchwald-Hartwig amination of bromobenzene (19a) with o-anisidine (18a) or p-anisidine (18b) gave the diphenylamine 20a and 20b, respectively. Similarly, amination of 4-bromoanisole (19b) with o-anisidine (18a) afforded the diphenylamine 20c. Diphenylamines 20a, 20b and 20c were subjected to a palladium(II)-catalyzed oxidative cyclization to produce corresponding methoxycarbazoles 21a,20 21b21 and 21c,22 which were treated with BBr3 to provide three hydroxycarbazoles 7, 9 and 11, respectively. 1-Hydroxycarbazole (7),20 3-hydroxycarbazole (9)23 and 1,6-dihydroxycarbazole (11) were used in our assays. Commercially available 2-hydroxycarbazole (8) and 4-hydroxycarbazole (10) were used.24
The structures of all synthesized compounds (Figure 1), except commercially available 8 and 10, were elucidated on the basis of 1H and 13C NMR spectra. Antioxidant activities against DPPH,25 and 2,2’azinobis-(3-ethylbenzthiazoline-6-sulfonate) cations (ABTS+)26 were measured.
Evaluation of DPPH radical scavenging activity is a rapid and convenient method for screening the antioxidant activity. In the DPPH radical scavenging assay (Table 1),25 hydroxycarbazoles 1, 3, 4, 5, 6, 7, 9 and 11 exhibited higher radical-scavenging activities than VE (15.4) and edaravone (19.7). Among them, simple 1-hydroxycarbazole (7) (10.4) and 3-hydroxycarbazole (9) (9.4) displayed the same potent scavenging activity as carbazomadurins A (3) (9.9) and B (4) (9.8)14 as reported previously. Compared to carazostatin (1), 3-hydroxycarbazole (9) exhibited the more potent radical scavenging activity. In addition, simple 1,6-dihydroxycarbazole (11) (8.0), the core structure of 3,8-dihydroxycarbazole carbazomadurins A (3) and (B) (4), exhibited the strongest radical scavenging activity of all tested compounds compared with PG (6.1). Furthermore, 1,3-dihydroxycarbazole 5 (13.7) and 1-hydroxy-3-ethoxycarbazole 6 (13.2) exhibited better radical scavenging activity than VE or edaravone. O-Ethylcarazostatin (2) and
2-hydroxycarbazole (8), however, exhibited weak antioxidant activities in this assay because they could not be transformed to a suitable iminoquinone form.
The ABTS+ radical assay is a conventional and excellent model for assessing the antioxidant activities of hydrogen-donation and chain-breaking antioxidants. In this assay, ABTS+ radicals were produced by reacting ABTS with potassium persulfate in sodium phosphate buffer solution.26 As shown in Table 1, all oxygenated carbazoles 1, 3-7, and 9-11, except O-ethylcarazostatin (2) and 2-hydroxycarbazole (8), exhibited better ABTS+ radical scavenging activities than the strong antioxidants PG (188) with better IC50 values.
Among them, seven hydroxycarbazoles 3 (112), 4 (116), 5 (116), 6 (121), 7 (118), 10 (116), and 11 (102) exhibited stronger ABTS+ radical scavenging activities than PG. Simple 1,3-dihydroxycarbazole 5, 1-hydroxycarbazole (7), and 4-hydroxycarbazole (10) possessed strong antioxidant activities similar to 3,6-dihydroxycarbazole alkaloids carbazomadurins A (3) and B (4). Based on this assay, the simple 1,6-dihydroxycarbazole (11) (102), the core structure of carbazomadurins A (3) and B (4), had the strongest antioxidant activity of all tested compounds. Although O-ethylcarazostatin (2) displayed weak DPPH radical scavenging activity, the ABTS+ radical scavenging activity of 2 (196) was between that of VE (211) and PG (188). The simple 2-hydroxycarbazole (8) exhibited the lowest ABTS+ radical scavenging activity in this assay.
For scavenging activity of the free radicals by hydroxycarbazoles, the O-H and N-H groups provided efficient DPPH scavenging activity.27-29 Both O-H and N-H bond dissociation energies, examined by density functional theory at ωB97X-D/6-31+G(d), were calculated for all of the tested compounds (Table 2). Among all of the carbazoles, except compound 2, the bond dissociation energies of the O-H groups were lower than those of the N-H group.
In conclusion, the simple phenolic carbazoles 5-11 were subjected to the evaluation of antioxidant activities by DPPH radical and ABTS+ radical scavenging assays in comparison with potent antioxidant alkaloids carazostatin (1), and carbazomadurins A (3) and B (4). Among them, the simple phenolic carbazoles 5-7, 9, and 11 exhibited stronger antioxidant activities than α-tocopherol, and similar antioxidant activities as phenolic carbazole alkaloids 1, 3, and 4. As a result, three simple phenolic carbazoles 7, 9, and 11 exhibited the strong antioxidant activities similar to the potent antioxidant alkaloids 3 and 4. 1-Hydroxycarbazole (7), 3-hydroxycarbazole (9) and 1,6-dihydroxycarbazole (11) are the minimum core structures of alkaloids 1, 3 and 4, respectively. Consequently, the existence of one or two hydroxy groups at least at the 1-, 3-, 6-, or 8-positions of the carbazole ring is essential to the iminoquinone form. By contrast, it is considered that the weak antioxidant activities of O-ethylcarazostatin (2) and 2-hydroxycarbazole (8) cannot be transformed to a suitable iminoquinone form through a radical scavenging process. Furthermore, the antioxidant activity by hydroxy groups in simple phenolic carbazoles 7-10 could be correlated with their EHOMO levels, but not their bond dissociation energies. Based on these results, the reducing ability of the phenolic carbazole core could be important role for the ability of carbazole alkaloids 1, 3, and 4.
EXPERIMENTAL
All non-aqueous reactions were carried out under nitrogen in dried glassware, unless otherwise noted. Solvents were dried and distilled according to standard protocols. Analytical thin layer chromatography was performed with silica gel 60PF254 (Merck). Silica gel column chromatography was performed with silica gel 60N (63-210 μm, Kanto Chemical Co., Inc.). All melting points were determined with a Yanagimoto micro melting point apparatus. The ultraviolet (UV) spectra were measured by a Shimadzu UV-2550 spectrophotometer. The proton nuclear magnetic resonance (1H NMR) spectroscopic data were recorded with a JEOL AL-300 at 300 MHz and a JEOL JMN-LA500 at 500 MHz. Chemical shifts are reported relative to Me4Si (δ = 0.00 ppm). The NMR spectroscopic data were recorded using CDCl3, unless otherwise noted. Multiplicities are indicated by s (singlet), d (doublet), t (triplet), q (quartet), m (multiplet), and br. (broad). The carbon nuclear magnetic resonance (13C NMR) spectroscopic data were recorded with a JEOL AL-300 at 75 MHz and JEOL JMN-LA500 at 125 MHz. Chemical shifts are reported relative to CDCl3 (δ = 77.0 ppm) and DMSO-d6 (δ = 39.7 ppm). Infrared spectra were recorded using the attenuated total reflectance (ATR) method with a Shimadzu FTIR-8000 spectrophotometer and Technologies DuraScop. Low and high resolution mass spectra were recorded with JEOL JMS-700 spectrometers by using a direct inlet system.
Synthesis of carbazoles
1,3-Dihydroxy-2-methylcarbazole (5): To a stirred solution of O-triflate-carbazole 1717 (100 mg, 0.29 mmol) in CH2Cl2 (4 mL) was added BBr3 (85 μL, 0.87 mmol) at -78 oC. After stirring at an ambient temperature for 12 h, the reaction mixture was poured into an ice water. The mixture was extracted with EtOAc. The EtOAc phase was washed with water and brine, dried with Na2SO4, and concentrated under reduced pressure. The residue was used for the next step without further purification. To a solution of the residue in THF (2 mL) was added LiAlH4 (33.0 mg, 0.87 mmol) at -78 oC. After stirring at rt for 5 h, the reaction mixture was quenched with EtOAc and water. The mixture was filtered off using Celite pad, and then the Celite pad washed with EtOAc. The filtrate was extracted with EtOAc. The EtOAc phase was washed with water and brine, dried with Na2SO4, and concentrated under reduced pressure. The residue was purified by column chromatography (silica gel, 20 g; EtOAc/hexane, 1/4 v/v) to give the 1,3-dihydroxycarbazole (5) (37 mg, 0.17 mmol, 60%) as a dark solid, mp 196-198 oC (EtOAc-hexane). IR (ATR): 3456, 3221 cm-1. 1H NMR (300 MHz, DMSO-d6): δ 2.16 (s, 3H), 6.97 (s, 1H), 7.01 (t, J = 7.7 Hz, 1H), 7.24 (t, J = 7.5 Hz, 1H), 7.40 (d, J = 7.7 Hz, 1H), 7.83 (d, J = 7.7 Hz, 1H), 8.76 (br. s, 1H), 8.79 (br. s, 1H), 10.33 (br. s, 1H). 13C NMR (75 MHz, DMSO-d6): δ 9.91, 96.35, 110.18, 111.45, 118.15, 120.01, 120.93, 123.16, 124.73, 124.95, 139.74, 140.64, 149.76. MS (EI): m/z 213[M]+. HRMS (EI): calcd. for C13H11NO2 213.0790; found 213.0754.
General procedure for the synthesis of N-phenylanilines
2-Methoxy-N-phenylaniline (20a): To a stirred solution of o-anisidine (18a) (1 g, 8.12 mmol) in dry toluene (10 mL) was added to a solution of bromobenzene (19a) (1.27 g, 8.12 mmol), Pd(OAc)2 (73 mg, 0.32 mmol), rac-BINAP (202 mg, 0.32 mmol) and K2CO3 (1.57 g, 11.37 mmol) at rt, and then the mixture was heated at 140 oC for 12 h. After cooling to an ambient temperature, the mixture was quenched with water. The resulting mixture was extracted with EtOAc. The EtOAc phase was washed with water and brine, dried with Na2SO4, and concentrated under reduced pressure. The residue was purified by column chromatography (silica gel, 20 g; EtOAc/hexane, 1/19 v/v) to give the 2-methoxy-N-phenylaniline (20a) (1.60 g, 8.03 mmol, 99%) as a colorless oil. IR (ATR): 3406 cm-1. 1H NMR (300 MHz, CDCl3): δ 3.90 (s, 3H), 6.15 (br. s, 1H), 6.82-6.97 (m, 4H), 7.14-7.17 (m, 2H), 7.25-7.32 (m, 3H). 13C NMR (75 MHz, CDCl3): δ 55.59, 110.50, 114.65, 118.55, 119.86, 120.78, 121.11, 129.24, 132.96, 142.69, 148.25. MS (EI): m/z 199 [M]+. HRMS (EI): calcd. for C13H13NO: 199.0997; found 197.0951.
4-Methoxy-N-phenylaniline (20b): The same procedure as above was carried out using p-anisidine (18b) (500 mg, 4.06 mmol), bromobenzene (19a) (637 mg, 4.06 mmol), Pd(OAc)2 (36 mg, 0.16 mmol), rac-BINAP (101 mg, 0.16 mmol) and K2CO3 (786 mg, 5.68 mmol) to give the 4-methoxy-N-phenylaniline (20b) (670 mg, 3.36 mmol, 83%) as a white solid, mp 105-107 oC (Et2O-hexane) (Lit.,30 mp 104-106 oC). IR (ATR): 3386 cm-1. 1H NMR (300 MHz, CDCl3): δ 3.85 (s, 3H), 5.54 (br. s, 1H), 6.86-6.97 (m, 5H), 7.10-7.15 (m, 2H), 7.24-7.30 (m, 2H). 13C NMR (75 MHz, CDCl3): δ 55.56, 114.64, 115.61, 119.54, 122.18, 129.28, 135.70, 145.14, 155.25. MS (EI): m/z 199 [M]+. HRMS (EI): calcd. for C13H13NO: 199.0997; found 197.0971.
2-Methoxy-N-(4-methoxyphenyl)aniline (20c): The same procedure as above was carried out using p-anisidine (18b) (500 mg, 4.06 mmol), o-bromoanisole (19b) (759 mg, 4.06 mmol), Pd(OAc)2 (36 mg, 0.16 mmol), rac-BINAP (101 mg, 0.16 mmol) and K2CO3 (786 mg, 5.68 mmol) to give the 2-methoxy-N-(4-methoxyphenyl)aniline (20c) (720 mg, 3.21 mmol, 79%) as a white solid, mp 70-71 oC (Et2O-hexane) (Lit.,31 mp 70-71 oC). IR (ATR): 3379 cm-1. 1H NMR (300 MHz, CDCl3): δ 3.81 (s, 3H), 3.91 (s, 3H), 5.98 (br. s, 1H), 6.75-6.91 (m, 5H), 7.05 (dd, J = 1.8, 9.0 Hz, 1H), 7.13 (d, J = 9.0 Hz, 2H). 13C NMR (75 MHz, CDCl3): δ 55.52(×2), 110.16, 112.55, 114.50, 118.50, 120.88, 122.71, 135.00, 135.37, 147.33, 155.25. MS (EI): m/z 229 [M]+. HRMS (EI): caclcd. for C14H15NO2: 229.1103; found 229.1112.
General procedure for the synthesis of carbazoles
1-Methoxycarbazole (21a): To a stirred solution of 2-methoxy-N-phenylaniline (20a) (200 mg, 1.00 mmol) in acetic acid (5 mL) was added to a solution of Pd(OAc)2 (270 mg, 1.21 mmol) at rt, and then the mixture was heated at 130 oC for 12 h. After cooling the reaction to an ambient temperature, the mixture was quenched with water. The resulting mixture was extracted with EtOAc. The EtOAc phase was washed with water and brine, dried with Na2SO4, and concentrated under reduced pressure. The residue was purified by column chromatography (silica gel, 20 g; EtOAc/hexane, 3/97 v/v) to give the 1-methoxycarbazole (21a) (120 mg, 0.61 mmol, 61%) as a white solid, mp 71-72 oC (Et2O-hexane) (Lit.,20 mp 70-72 oC). IR (ATR): 3413 cm-1. 1H NMR (300 MHz, CDCl3): δ 4.00 (s, 3H), 6.90 (d, J = 7.9 Hz, 1H), 7.17 (t, J = 7.9 Hz, 1H), 7.21-7.27 (m, 1H), 7.39-7.46 (m, 2H), 7.70 (d, J = 7.9 Hz, 1H), 8.07 (d, J = 7.9 Hz, 1H), 8.28 (br. s, 1H). 13C NMR (75 MHz, CDCl3): δ 55.46, 105.89, 110.89, 112.83, 119.32, 119.70, 120.51, 123.61, 124.29, 125.64, 129.75, 139.14, 145.63. MS (EI): m/z 197 [M]+. HRMS (EI): caclcd. for C13H11NO: 197.0841; found 199.0817.
3-Methoxycarbazole (21b): The same procedure as above was carried out using 4-methoxy-N-phenylaniline (20b) (300 mg, 1.51 mmol), Pd(OAc)2 (405 mg, 1.81 mmol) to give the 3-methoxycarbazole (21b) (93 mg, 0.47 mmol, 31%) as a white solid, mp 151-153 oC (EtOAc-hexane) (Lit.,21 mp 146-148 oC). IR (ATR): 3421 cm-1. 1H NMR (300 MHz, CDCl3): δ 7.07 (dd, J = 2.5, 7.9 Hz, 1H), 7.18-7.24 (m, 1H), 7.34 (d, J = 7.9 Hz, 1H), 7.40 (d, J = 2.5 Hz, 1H), 7.56 (d, J = 2.5 Hz, 1H), 7.92 (br. s, 1H), 8.03 (d, J = 7.9 Hz, 1H). 13C NMR (75 MHz, CDCl3): δ 56.07, 103.18, 110.72, 111.27, 115.05, 119.04, 120.24, 123.35, 123.79, 125.78, 134.36, 140.27, 153.89. MS (EI): m/z 197 [M]+. HRMS (EI): calcd. for C13H11NO: 197.0841; found 199.0860.
1,6-Dimethoxycarbazole (21c): The same procedure as above was carried out using 4-methoxy-N-(2-methoxyphenyl)aniline (20c) ( (300 mg, 1.31 mmol), Pd(OAc)2 (352 mg, 1.57 mmol) to give the 1,6-dimethoxycarbazole (21c) (147 mg, 0.65 mmol, 49%) as a white solid, mp 120-121 oC (EtOAc-hexane) (Lit.,23 mp 118-120 oC). IR (ATR): 3410 cm-1. 1H NMR (300 MHz, CDCl3): δ 3.90 (s, 3H), 4.01 (s, 3H), 6.89 (d, J = 7.9 Hz, 1H), 7.06 (dd, J = 2.5, 7.9 Hz, 1H), 7.13 (t, J = 7.9 Hz, 1H), 7.36 (d, J = 7.9 Hz, 1H), 7.52 (d, J = 2.5 Hz, 1H), 7.63 (d, J = 7.9 Hz, 1H), 8.12 (br. s, 1H). 13C NMR (75 MHz, CDCl3): δ 103.18, 105.74, 111.62, 112.75, 115.08, 119.32, 124.05, 124.27, 130.61, 134.11, 145.75, 153.81. MS (EI): m/z 227 [M]+. HRMS (EI): calcd. for C14H13NO2: 227.0946; found 227.0980.
General procedure for the synthesis of hydroxycarbazoles
1-Hydroxycarbazole (7): To a solution of 1-methoxycarbazole (21a) (85 mg, 0.43 mmol) in CH2Cl2 (2 mL) was added BBr3 (169 μL, 1.72 mmol) at -78 oC. After stirring at rt for 5 h, the reaction mixture was poured into ice water. The mixture was extracted with EtOAc. The EtOAc phase was washed with water and brine, dried with Na2SO4, and concentrated under reduced pressure. The residue was purified by column chromatography (silica gel, 20 g; EtOAc/hexane, 1/4 v/v) to give the 1-hydroxycarbazole (7) (51 mg, 0.28 mmol, 65%) as a white solid, mp 165-167 oC (CHCl3) (Lit.,20 mp 163-165 oC). IR (ATR): 3429, 3197 cm-1. 1H NMR (300 MHz, CDCl3): δ 4.99 (br. s, 1H), 6.83 (d, J = 7.9 Hz, 1H), 7.08 (t, J = 7.9 Hz, 1H), 7.21-7.26 (m, 1H), 7.40-7.48 (m, 2H), 7.69 (d, J = 7.9 Hz, 1H), 8.06 (d, J = 7.9 Hz, 1H), 8.24 (br. s, 1H). 13C NMR (75 MHz, CDCl3): δ 110.63, 110.94, 113.25, 119.44, 119.68, 120.59, 123.57, 125.34, 125.93, 128.95, 139.41, 141.00. MS (EI): m/z 183 [M]+. HRMS (EI): calcd. for C12H9NO: 183.0684; found 183.0706.
3-Hydroxycarbazole (9): The same procedure as above was carried out using 3-methoxycarbazole (21b) (90 mg, 0.46 mmol), BBr3 (179 μL, 1.83 mmol) to give the 3-hydroxycarbazole (9) (50 mg, 0.27 mmol, 60%) as a brown solid, mp 234-236 oC (EtOAc-hexane) (Lit.,23 mp 260-261 oC). IR (ATR): 3398, 3151 cm-1. 1H NMR (300 MHz, CDCl3): δ 6.87 (dd, J = 2.6, 7.8 Hz, 1H), 7.05 (t, J = 7.8 Hz, 1H), 7.25-7.32 (m, 2H), 7.37-7.40 (m, 2H), 7.96 (d, J = 7.8 Hz, 1H), 8.90 (br. s, 1H), 10.85 (br. s, 1H). 13C NMR (75 MHz, CDCl3), δ 104.83, 110.81, 111.28, 114.99, 117.70, 120.06, 122.27, 123.03, 125.18, 133.74, 140.39, 150.37. MS (EI): m/z 183 [M]+. HRMS (EI): calcd. for C12H9NO: 183.0684; found 183.0666.
1,6-Dihydroxycarbazole (11): The same procedure as above was carried out using 1,6-dimethoxycarbazole (21c) (147 mg, 0.65 mmol), BBr3 (253 μL, 2.59 mmol) to give the 1,6-dihydroxycarbazole (11) (63 mg, 0.28 mmol, 49%) as a dark soliid, mp 200-202 oC (EtOAc-hexane). IR (ATR): 3413, 3278 cm-1. 1H NMR (300 MHz, DMSO-d6): δ 6.74 (d, J = 7.5 Hz, 1H): 6.81-6.88 (m, 2H), 7.25 (d, J = 7.5 Hz, 1H), 7.31 (d, J = 2.0 Hz, 1H), 7.39 (d, J = 7.5 Hz, 1H), 8.84 (br. s, 1H), 9.62 (br. s, 1H), 10.61 (br. s, 1H). 13C NMR (75 MHz, DMSO-d6): δ 105.47, 110.56, 112.12, 112.67, 115.59, 119.57, 124.22, 124.55, 130.75, 134.45, 143.48, 150.50. MS (EI): m/z 199 [M]+. HRMS (EI): calcd. for C12H9NO2: 199.0633; found 199.0627.
Radical scavenging assays
DPPH (047-04051) and ABTS (018-10311) were purchased from Wako Pure Chemical Industries, Ltd. All the data were statistically analyzed with student’s test, and the data, whose association was calculated less than 0.01 of p score, took significantly difference each other. Inhibition concentrations at which 50% of the radicals were scavenged (IC50 values) were calculated to evaluate the antioxidant activity.
DPPH radical scavenging assay:25 Stock solutions were prepared for DPPH (200 µM) and the test samples (100 µM) in MeOH. All solutions were kept cool and in the dark until use. For each sample different aliquots of the stock solutions were taken and the volume was adjusted to 3 mL in MeOH. The reaction was started by adding 1 mL of 200 µM DPPH stock solution (final DPPH concentration 50 µM). After shaking for 30 min at ambient temperature in the dark, the absorbance was measured at 517 nm in MeOH using a Shimadzu UV-2550 spectrophotometer. DPPH radical scavenging capacity was expressed as μM, the results were expressed as means ± S.E. (n = 3 or higher, and the results of one of at least three similar experiments are shown). DPPH radical scavenging (capacity) (%) = (Acontrol –Asample)/ Acontrol x 100%, where Acontrol is the absorbance of the control (DPPH radical without test sample) and Asample is the absorbance of the test sample (DPPH radical with test sample). The control contain all reagents except the scavenger.
ABTS+ radical scavenging assay:26 The procedure to prepare the ABTS+ radical stock solution was modified slightly.26 Sufficient amounts of the diammonium salts of ABTS and K2S2O8 were dissolved in 5.00 mL H2O to achieve concentrations of 7.00 and 1.40 mM, respectively. This solution was kept in the dark for at least 16 h to form ABTS+ radical, then diluted to 100 mL with MeOH so that the solution had an absorbance or Acontrol of ~0.80 at 734 nm using a Shimadzu UV-2550 spectrophotometer. Various concentrations of carbazoles were added to ABTS+ radical solution at ambient temperature to reach a stable absorbance (Acontrol). Then, the percentage of ABTS+ radical scavenged was calculated as follows. ABTS+ radical scavenging (capacity) (%) = Acontrol –Asample)/ Acontrol x 100%, where Acontrol is the absorbance of the control (ABTS+ radical without test sample) and Asample is the absorbance of the test sample (ABTS+ radical with test sample). The control contain all reagents except the scavenger. The concentration-percentage of scavenged ABTS+ radical relationships for each reagent, which were derived from their IC50 values, were fitted to a four-parameter logistic equation using a non-linear curve-fitting program (KaleidaGraph; Synergy Software, Reading, PA). Where appropriate, the results were expressed as means ± S.E. (n = 3 or higher, and the results of one of at least three similar experiments are shown).
Bond dissociation (BD) energy and HOMO energy35
Carbazoles and their corresponding radicals were calculated by density functional theory (DFT) at ωB97X-D/6-31+G(d). The bond dissociation energies (BD energies) were calculated as the following energies: BD energies = Energy of antioxidant radical + Energy of H radical - Energy of antioxidant.
ACKNOWLEDGEMENTS
This work was partly supported by a Grant-in Aid for Scientific Research (C) (No. 17590025) of the Japan Society for the Promotion of Science (JSPS).
References
1. (a) W. W. Droge, Physiol. Rev., 2002, 82, 47; CrossRef (b) J. Hardy and D. J. Selkoe, Science, 2002, 297, 296; CrossRef (c) B. D’Autreaux, Nat. Rev. Mol. Cell Biol., 2007, 8, 813; CrossRef (d) B. Uttara, A. V. Songh, P. Zamboni, and R. T. Maharajan, Curr. Neuropharmacol., 2009, 7, 65; CrossRef (e) S. Reuter, S. C. Gupta, M. M. Chaturvedi, and B. B. Aggarwal, Free Radical Biol. Med., 2010, 49, 1603; CrossRef (f) E. D. Marchi, F. Baldassari, A. Bononi, M. R. Wieckowski, and P. Pinton, Oxid. Med. Cell. Longev., 2013, 564961; (g) O. Firuzi, A. Miri, M. Tavakoli, and L. Saso, Curr. Med. Chem., 2009, 41, 1261; (h) J. Teixeira, T. Silva, P. B. Andrade, and F. Borges, Curr. Med. Chem., 2013, 18, 134; (i) S. Chakrabarti, M. Sinha, I. G. Thakurta, P. Banerjee, and M. Chattopadyay, Curr. Med. Chem., 2013, 20, 4648; CrossRef (j) J. Hardy, N. Bogdanovic, B. Wintlad, E. Portelius, N. Andreasen, A. Cedazo-Minguez, and H. Zetterberg, J. Int. Med., 2014, 275, 296. CrossRef
2. (a) K. Houkin, N. Nakayama, K. Kamada, T. Noujou, H. Abe, and T. Kashiwaba, J. Stroke Cerebrovasc. Dis., 1998, 7, 315; CrossRef (b) H. Yoshino and A. Kimura, Amyotroph. Lateral Scler., 2006, 7, 247; CrossRef (c) K. Abe, Y. Itoyama, G. Sobue, S. Tsuji, M. Aoki, M. Doyu, C. Hamada, K. Kondo, Y. Yoneoka, M. Akimoto, and H. Yoshino, Amyotroph. Lateral Scler., 2014, 15, 610. CrossRef
3. (a) U. Pindur, Chimia, 1990, 219; (b) J. Bergman and B. Pelcman, Pure Appl. Chem., 1990, 62, 1967l; CrossRef (c) D. P. Chakraborty, In The Alkaloids, ed. by G. A. Cordell, Academic Press, New York, 1993, Vol. 44, p 257; (d) H.-J. Knölker, Chem. Soc. Rev., 1999, 28, 151; CrossRef (e) H.-J. Knölker and K. R. Reddy, Chem. Rev., 2002, 102, 4303; CrossRef (f) A. W. Schmidt, K. R. Reddy, and H.-J. Knölker, Chem Rev., 2012, 112, 3193; CrossRef (g) J. K. Roy, A. K. Jana, and D. Mal, Tetrahedron, 2012, 68, 6099. CrossRef
4. (a) K. Sakano, K. Ishimaru, and S. Nakamura, J. Antibiot., 1980, 33, 683; CrossRef (b) K. Sakano and S. Nakamura, J. Antibiot., 1980, 33, 961; CrossRef (c) M. Kaneda, K. Sakano, S. Nakamura, Y. Kushi, and Y. Iitaka, Heterocycles, 1981, 15, 993; CrossRef (d) K. Yamasaki, M. Kaneda, K. Watanabe, Y. Ueki, K. Ishimaru, and S. Nakamura, J. Antibiot., 1983, 36, 552; CrossRef (e) S. Kondo, M. Katayama, and Marumo, J. Antibiot., 1986, 39, 727; CrossRef (f) T. Naid, T. Kitahara, M. Kaneda, and S. Nakamura, J. Antibiot., 1987, 40, 157; CrossRef (g) D. J. Hook, J. J. Yacobucchi, S. O’Connor, M. Lee, E. Kernes, B. Krishnan, J. Matson, and G. Hesler, J. Antibiot., 1990, 43, 1347. CrossRef
5. (a) M. Stiborova, A. Breuer, D. Aimova, M. Stiborova-Rupertova, M. Wiessler, and E. Frei, Int. J. Cancer, 2003, 107, 885; CrossRef (b) N. C. Garbett and D. E. Graves, Curr. Med. Chem., 2004, 4, 149; (c) J. Poljakova, J. Hrebackova, M. Dvorakova, T. Moserova, T. Eckschlager, J. Hrabeta, M. Gottlicherova, B. Kopejtkova, E. Frei, R. Kizek, and M. Stiborova, Neuroendocrinol. Lett., 2011, 32, 101; (d) R. Mori, A. Kato, K. Komenoi, H. Kurasaki, T. Iijima, M. Kawagoshi, Y. B. Kiran, S. Takeda, N. Sakai, and T. Konakahara, Eur. J. Med. Chem., 2014, 82, 16. CrossRef
6. (a) R. W. Rickards, J. M. Rothchiled, A. C. Willis, N. M. de Chazal, J. Kirk, K. Kirk, K. J. Saliba, and G. D. Smith, Tetrahedron, 1999, 55, 13513; CrossRef (b) P. H. Bernard, C. L. L. Chai, G. A. Heath, P. J. Mahon, G. D. Smith, P. Waring, and B. A. Wilkes, J. Med. Chem., 2004, 47, 4958; CrossRef (c) P. H. Bernard, C. L. L. Chai, M. Le Guen, G. D. Smith, and P. Waring, Bioorg. Med. Chem. Lett., 2007, 17, 82; CrossRef (d) K. Matsumoto, T. Choshi, M. Hourai, Y. Zamami, K. Sasaki, T. Abe, M. Ishikura, N. Hatae, T. Iwamura, S. Tohyama, J. Nobuhiro, and S. Hibino, Bioorg. Med. Chem. Lett., 2012, 22, 4762; CrossRef (e) N. Hatae, R. Satoh, H. Chiba, T. Osaki, T. Nishiyama, M. Ishikura, T. Abe, S. Hibino, T. Choshi, C. Okada, and E. Toyota, Med. Chem. Res., 2014, 23, 4956. CrossRef
7. K. Takeya, M. Itoigawa, and H. Furukawa, Eur. J. Pharmacol., 1989, 169, 137. CrossRef
8. A. Aygun and U. Pindur, J. Heterocycl. Chem., 2003, 40, 411. CrossRef
9. (a) S. Kato, H. Kawai, T. Kawasaki, Y. Toda, T. Urata, and Y. Hayakawa, J. Antibiot., 1989, 42, 1879; CrossRef (b) M. Iwatsuki, E. Niki, S. Kato, and K. Nishikori, Chem. Lett., 1992, 1735. CrossRef
10. M. Iwatsuki, E. Komuro, and E. Niki, Bull. Chem. Soc. Jpn., 1995, 68, 620. CrossRef
11. N. Kotoda, K. Shinya, K. Furihata, Y. Hayakawa, and H. Seto, J. Antibiot., 1997, 50. 770. CrossRef
12. (a) K. Shin-ya, M. Tanaka, K. Furihata, Y. Hayakawa, and H. Seto, Tetrahedron Lett., 1993, 34, 4943; CrossRef (b) K. Shin-ya, T. Kunigami, J.-S. Kim, K. Furihata, Y. Hayakawa, and H. Seto, Biosci. Biotechnol. Biochem., 1997, 61, 1768. CrossRef
13. K. Schneider, J. Nachtigall, A. Hanchen, G. Nicholson, M. Goodfellow, R. D. Sussmuth, and H.-P. Fiedler, J. Nat. Prod., 2009, 72, 1768. CrossRef
14. Y. Hieda, M. Anraku, T. Choshi, H. Tomida, H. Fujioka, N. Hatae, O. Hori, J. Hirose, and S. Hibino, Bioorg. Med. Chem. Lett., 2014, 24, 3530. CrossRef
15. G. Fabre, A. Hänchen, C. A. Calliste, K. Berka, S. Banala, M. Otyepka, R. D. Süssmuth, and P. Trouillas, Bioorg. Med. Chem., 2015, 23, 4866. CrossRef
16. K. Arai, F. Kumakura, M. Takahira, N. Sekiyama, N. Kuroda, T. Suzuki, and M. Iwaoka, J. Org. Chem., 2015, 80, 5633. CrossRef
17. (a) T. Choshi, T. Sada, H. Fujimoto, C. Nagayama, E. Sugino, and S. Hibino, Tetrahedron Lett., 1996, 37, 2593; CrossRef (b) T. Choshi, T. Sada, H. Fujimoto, C. Nagayama, E. Sugino, and S. Hibino, J. Org. Chem., 1997, 62, 2535. CrossRef
18. (a) Y. Hieda, T. Choshi, S. Kishida, H. Fujioka, and S. Hibino, Tetrahedron Lett., 2010, 51, 3593; CrossRef (b) Y. Hieda, T. Choshi, H. Fujioka, and S. Hibino, Eur. J. Org. Chem., 2013, 7391. CrossRef
19. (a) C. Borger and H.-J. Knölker, Tetrahedron, 2012, 68, 6727; CrossRef (b) K. K. Jurich-Gruner, O. Kataeva, A. W. Schmidt, and H.-J. Knölker, Chem. Eur. J., 2014, 20, 8536; CrossRef (c) F. Fumagalli and F. da S. Emery, Tetrahedron Lett., 2015, 56, 2307. CrossRef
20. T. Martin and C. J. Moody, J. Chem. Soc., Perkin Trans. 1, 1988, 235. CrossRef
21. R. B. Bedford and M. Betham, J. Org. Chem., 2006, 71, 9403. CrossRef
22. P. Bernal, A. Benavides, R. Bautista, and J. Tamaritz, Synthesis, 2007, 1943. CrossRef
23. (a) E. Bisagni, C. Ducrocq, and N. C. Hung, Tetrahedron, 1980, 36, 1327; CrossRef (b) H. McNab and R. G. Tyas, J. Org. Chem., 2007, 72, 8760. CrossRef
24. Compounds 8 and 10 were purchased from Wako Pure Chemical Industries, Ltd., Japan and Tokyo Chemical Industry Co., Ltd., Japan, respectively.
25. O. P. Sharma and T. K. Bhat, Food Chem., 2009, 113, 1202. CrossRef
26. Y.-Z. Tang and Z.-Q. Liu, J. Am. Oil Chem. Soc., 2007, 84, 1095. CrossRef
27. P. Trouillas, P. Marsal, D. Siri, R. Lazzaroni, and J.-L. Duroux, Food Chem., 2006, 97, 679. CrossRef
28. E. Anouar, C. A. Calliste, P. Kosinová, F. Di Meo, J. L Duroux, Y. Champavier, K. Marakchi, and P. Trouillas, J. Phys. Chem. A, 2009, 113, 13881. CrossRef
29. F. Di Meo, V. Lemaur, J. Cornil, R. Lazzaroni, J.-L. Duroux, Y. Olivier, and P. Trouillas, J. Phys. Chem. A, 2013, 117, 2082. CrossRef
30. J. Yu, Y. Wang, P. Zhang, and J. Wu, Synlett, 2013, 24, 1448. CrossRef
31. R. Bautista, A. V. Jerezano, and J. Tamariz, Synthesis, 2012, 44, 3327. CrossRef