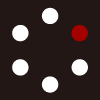
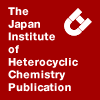
HETEROCYCLES
An International Journal for Reviews and Communications in Heterocyclic ChemistryWeb Edition ISSN: 1881-0942
Published online by The Japan Institute of Heterocyclic Chemistry
e-Journal
Full Text HTML
Received, 16th November, 2015, Accepted, 4th December, 2015, Published online, 16th December, 2015.
DOI: 10.3987/COM-15-13366
■ Radical Scavenging Activity of Ascorbic Acid Analogs Containing a Carbonyl Conjugated Ene-Diol Structure
Shogo Nomura, Keiko Inami, and Masataka Mochizuki*
Faculty of Pharmaceutical Sciences, Tokyo University of Science, 2641 Yamazaki, Noda-shi, Chiba 278-8510, Japan
Abstract
Small molecule antioxidants such as ascorbic acid (AscH2) prevent the oxidative damage of biological molecules by scavenging reactive oxygen species. A carbonyl-conjugated ene-diol structure is essential for the antioxidant activity of AscH2. As such, we synthesized novel AscH2 analogs containing a carbonyl conjugated ene-diol structure and evaluated their radical scavenging activities. When the 1,2-dihydroxyethyl group was removed, radical scavenging activity equal to AscH2 was observed. Analogs containing an endocyclic nitrogen atom instead of a ring oxygen displayed higher radical scavenging activities than AscH2. Therefore, the electron donating effect of the carbonyl conjugated ene-diol structure greatly increased the radical scavenging activity of AscH2 analogs.INTRODUCTION
The human body is susceptible to attack by reactive oxygen species (ROS), resulting in adverse health effects.1 ROS generated by the transfer of single electrons to O2 are capable of damaging cellular components, such as DNA, proteins, and lipids.1,2 These ROS are controlled by an endogenous defense system, such as enzymatic and chemical reduction.1,3 As such, antioxidants are of great interest due to their involvement in biological processes, such as cancer, cardiovascular disease, and inflammation caused by ROS.1-4 Hence, a balance between ROS and antioxidants is necessary for the management of human health.5-7 To accomplish this balance, a continuing objective of many academic and industrial scientists is the design and development of novel antioxidants based on natural antioxidants, such as cinnamic acid,8 resveratrol,9 α-tocopherol,10 and ascorbic acid (AscH2).11,12 In particular, many derivatives of AscH2 have been developed to improve their stability and lipophilicity in cosmetics and food additives.11-14 However, the antioxidant activity of AscH2 analogs, which including a nitrogen atom instead of an endocyclic oxygen atom, have not been reported. Since the fact that the polar antioxidants are more effective in less polar media, the knowledge of the chemistry of AscH2 or AscH2 analogs in nonaqueous media is vital to rationalize of their role.15,16
In this study, we prepared compounds 1–3 and synthesized novel compounds 4–6 containing a carbonyl conjugated ene-diol structure, which is the key structural moiety for the antioxidant activity of AscH2 (Figure 1).17 The antioxidant activities of the synthesized AscH2 analogs were evaluated in a nonaqueous system using the galvinoxyl radical.18
RESULTS AND DISCUSSION
Chemistry
The antioxidant active site of AscH2 is a carbonyl conjugated ene-diol structure within the five-membered ring (Figure 2). As such, we synthesized AscH2 analogs that maintained this active site.
To clarify the effect of the side chain on antioxidant activity, compound 1 was prepared according to the method reported by Ramage19,20 and investigated for its radical scavenging activity. Compounds 2 and 3, wherein the endocyclic oxygen atom in compound 1 was changed to a carbon and nitrogen atom, respectively, were synthesized to compare their antioxidant activities to that of AscH2.
Carbocyclic ring compound 2 was prepared according to the method reported by Hesse,21 with the exception of the use of bromine as opposed to chlorine to simplify the operation. Compound 3 was prepared according to the method reported by Lowe and Stachel.22,23 To ensure easy handling, diazotization reagent methanesulfonyl azide was converted to p-toluenesulfonyl azide. In addition, a sealed tube was used in place of an autoclave. N-Substituted AscH2 analogs (4–6) were synthesized to obtain a more powerful antioxidant effect than compound 3, which provided the highest radical scavenging activity among compounds 1–3.
Compounds 4 and 5 were prepared by reaction with N-ethylglycine ethyl ester24-26 and N-cyanomethylglycine ethyl ester,27 respectively, using a method similar to that previously reported,22,23 and were expected to induce electron donating and electron withdrawing effects, respectively, through the nitrogen atom in the five-membered ring. Conversely, compound 6 contained an acetyl group and was synthesized from compound 10c (Scheme 1).28 This resulted in a decrease in the number of electrons in the conjugated system.
Galvinoxyl radical scavenging activity of AscH2 analogs
The antioxidant activities of AscH2 analogs were evaluated in methanol using the galvinoxyl radical, as this is one of the simplest assays to evaluate reactivity with ROS in vitro.18,29,30 The rate of the galvinoxyl radical scavenging reaction with AscH2 analogs was measured by monitoring the decrease in absorbance at 429 nm using a stopped-flow technique (Scheme 2).
The radical scavenging reactions of AscH2 analogs with the galvinoxyl radical were compared and followed by the initial rate method.31,32 When the initial rate method was used, the results were uncomplicated by the effect of the reverse or side reactions. Provided the initial data can be extended to the first 10% of the reaction, all effects are minimized. Initial reaction rates (ki) were calculated from the drop in initial absorbance over the linear reaction regions, as determined by regression equations with R2 > 0.9 (Figures 3 and 4).
A plot of lnki versus ln[AscH2 analogs] demonstrated a linear correlation with a positive slope (approximately 0.5), indicating that the reaction between AscH2 analogs and the galvinoxyl radical proceeded via a similar mechanism (Table 1).
The kinetic experiment is given by the equation
The reaction between AscH2 and the galvinoxyl radical, which generates dehydroascorbic acid (dehydroAsc) is illustrated below:
As reaction 5 does not occur, it can be ignored. Assuming reaction 3 is the rate determining step,
If reaction 4 is regarded as an equilibrium,
Therefore, equation 7 can be substituted into equation 6 to give
Thus, the concentration of dehydroAsc can be regarded as a constant, with the exception of the AscH2 initial concentration in the initial rate method, giving
Therefore, equation 8 can be simplified to
Equations 1 and 9 indicated that reaction 3 was the rate determining step of the reaction between AscH2 and the galvinoxyl radical.
The radical scavenging activity increased in the following order: AscH2 ≤ 1 ≤ 2 << 6 < 3 < 5. The radical scavenging activity of compound 4 could not be estimated as it decomposed even when deaerated using argon gas. The radical scavenging activity of compounds 1 and 2 was comparable to that of AscH2. Therefore, the side chain of AscH2 and the endocyclic oxygen atom are not necessary for the radical scavenging reaction. Conversely, compounds 3, 5, and 6, which contained a nitrogen atom instead of the endocyclic oxygen atom of compound 1, demonstrated significantly increased radical scavenging activities. A nitrogen atom, being more electron donating than an oxygen atom, better stabilizes the electron-poor intermediate radical of AscH2 analogs, weakening the O–H bond and accelerating the rate of the reaction.33 The radical scavenging activity of compound 6, which contained an electron withdrawing acetyl group that provides a resonance effect, was weaker than compound 3. Thus, the resonance effect of the acetyl group reduced the electron donating effect of the nitrogen atom in the ring.
CONCLUSION
ROS induce oxidative damage of DNA, biomembranes, and proteins, resulting in the onset of various diseases. AscH2 has a powerful antioxidant activity that protects the body from oxidative stress. In this paper, to increase the antioxidant activity of AscH2, we synthesized AscH2 analogs containing a carbonyl conjugated ene-diol structure, which is essential for antioxidant activity, and evaluated their radical scavenging activities. The radical scavenging activity of compounds 1 and 2 was equal to that of AscH2; thus, the side chain of AscH2 and the endocyclic oxygen atom were not necessary for radical scavenging activity. Compounds 5 and 6, which included a nitrogen atom in the five-membered ring, demonstrated significantly increased radical scavenging activities. Therefore, the electron donating effect of the endocyclic nitrogen atom is the key to increasing the radical scavenging activity of AscH2 analogs.
EXPERIMENTAL
Reagents
Acetic anhydride, bromine, tert-butyl alcohol, dicyclohexylcarbodiimide, 4-dimethylaminopyridine, diisopropylamine, glycolic acid, potassium bicarbonate, sodium acetate, sodium azide, triethylamine, and trifluoroacetic acid were obtained from Wako Pure Chemical Industries, Ltd. (Osaka, Japan). Ammonium sulfate, tert-butyl alcohol, n-butyllithium, cyclopentanone, and sodium iodide were obtained from Kanto Chemical Co., Inc. (Tokyo, Japan). Chloroacetonitrile, cyclohexanone, ethylbenzylamine, ethyl bromoacetate, glycine ethyl ester hydrochloride, rhodium(II) acetate dimer, methylbenzylamine, thioglycolic acid, p-toluenesulfonic acid, and p-toluenesulfonyl chloride were obtained from Tokyo Chemical Industry Co., Ltd. (Tokyo, Japan). Ethyl hydrogen malonate was obtained from Alfa Aesar (Heysham, UK). L-Ascorbic acid was obtained from Nikko Pharmaceutical Co., Ltd. (Gifu, Japan). All reagents and solvents used were of the best commercially available quality and were not further purified unless otherwise noted.
Reaction progression was monitored using thin-layer chromatography (TLC) on silica gel 60 F254 plates (0.25 mm, Merck). Column chromatography was performed on silica gel 60 (0.063–0.200 mm, Merck). Melting points were recorded with a Yanako MP-J3 apparatus (Kyoto, Japan). Infrared spectra were recorded on a Perkin-Elmer FT-IR/Spectrum 100 spectrometer (MA, USA). 1H NMR spectra were recorded with a JOEL RESONANCE JNM-LA 400 spectrometer (Tokyo, Japan). Chemical shifts were expressed in terms of ppm shifted downfield from tetramethylsilane (s = singlet, d = doublet, t = triplet, m = multiplet, br = broad peak). Mass spectra were determined using JEOL JMS-700 (Tokyo, Japan) and VARIAN 910-MS spectrometers (CA, USA).
Chemistry
General procedure 1
We synthesized N-substituted (ethoxycarbonylacetyl)glycine ethyl esters 8a and 8b. A solution of N-substituted glycine ethyl ester (1 equiv) and ethyl hydrogen malonate (1 equiv) in CH2Cl2 was cooled to 0 °C. A solution of N,N’-dicyclohexylcarbodiimide (DCC) in CH2Cl2 was then added drop-by-drop and stirred for 30 min at 0 °C; then, the reaction mixture was warmed to room temperature and stirred for 2–3 h. The white precipitate was filtered to remove N,N’-dicyclohexylurea, washed with water, and dried over Na2SO4. The extract was filtered and concentrated under reduced pressure. The crude product was purified by column chromatography (EtOAc: n-hexane = 1: 1).
General procedure 2
We synthesized N-substituted 3-diazopyrrolidine-2,4-diones 10a and 10b. N-Substituted (ethoxycarbonylacetyl)glycine was dissolved in benzene at 60 °C. A sodium methoxide methanol solution (1 equiv) was then added drop-by-drop to the mixture, refluxed for 1–2 h, and cooled to room temperature. The reaction mixture was extracted with water and treated with conc. HCl(aq). The precipitated product was filtered and washed with water. The resultant solid was dissolved in dry MeCN and refluxed overnight. The reaction mixture was then cooled to room temperature and concentrated under reduced pressure. p-Toluensulfonyl azide (1.1 equiv) was added to the residue and cooled to 0 °C, followed by the drop-by-drop addition of triethylamine (1 equiv) at 0 °C for 30 min. After 30 min, the reaction mixture was stirred at room temperature for 30–60 min. The solution was then evaporated to give a red gum. The residue was washed with CCl4, extracted with CHCl3, dried over Na2SO4, filtered, and evaporated. The crude product was purified by column chromatography (5–10% MeOH-CHCl3).
General procedure 3
We synthesized N-substituted 3-tert-butoxy-4-hydroxy-2(5H)-pyrrol-2-ones 11a–c. To a solution of N-substituted 3-diazopyrrolidine-2,4-dione in tert-butyl alcohol, we added rhodium(II) acetate (0.1 mol%) at 60 °C and the resulting mixture was refluxed overnight. The reaction mixture was evaporated and recrystallized from EtOH to isolate the desired product.
General procedure 4
We synthesized N-substituted 3,4-dihydroxy-2(5H)-pyrrol-2-ones 4–6. To a solution of N-substituted 3-tert-butoxy-4-hydroxy-2(5H)-pyrrol-2-one in dioxane, we added dry p-toluenesulfonic acid (5 mol%) at 60 °C and the resulting mixture was refluxed overnight. The reaction mixture was then slowly cooled to room temperature, and the precipitated solid was filtered to yield the desired product.
Synthesis of 2-acetoxycyclopent-2-en-1-one.21
A solution of cyclopentanone (4.43 mL, 50 mmol) in acetic acid (40 mL) was heated to 30 °C. A solution of bromine (5.01 mL, 100 mmol) in acetic acid (10 mL) was then added drop-by-drop and stirred for 30 min at 30–40 °C; then, sodium acetate (16.4 g, 200 mmol) was added and stirred for 2 h at 70 °C. The reaction mixture was filtered to remove excess sodium acetate, washed with acetic acid, and dried over Na2SO4. The extract was filtered and concentrated under reduced pressure. The crude product was purified by column chromatography (n-hexane: EtOAc = 3: 2), yielding pale yellow oil (1.40 g, 20%). 1H NMR (400 MHz, CDCl3, δ): 7.41 (t, 1H, J = 2.9 Hz), 2.65-2.71 (m, 2H), 2.46-2.48 (m, 2H), 2.27 (s, 3H).
Synthesis of 3-bromo-2-hydroxycyclopent-2-en-1-one.21
To a solution of 2-acetylcyclopent-2-en-1-one (1.40 g, 10 mmol) in Et2O (40 mL), was added bromine (0.551 mL, 11 mmol) in CCl4 (5.0 mL) and stirred for 30 min at room temperature. The reaction mixture was concentrated under reduced pressure, and added CCl4 (5 mL), filtered and washed with cooled CCl4 to yield the desired product, yielding brown needle (328 mg, 19%). 1H NMR (400 MHz, CDCl3, δ): 6.03 (s, 1H), 2.84-2.87 (m, 2H), 2.58-2.60 (m, 2H), 2.27 (s, 3H).
Synthesis of 2,3-dihydroxycyclopent-2-en-1-one (reductic acid, 2).21
A solution of 3-bromo-2-hydroxycyclopent-2-en-1-one (124 mg, 0.70 mmol) in 5% sulfuric acid (5.0 mL) was refluxed for 3 h. The resulting mixture was added Et2O, saturated with ammonium sulfate, washed with Et2O and extracted with EtOAc. The extract was dried over Na2SO4, filtered and concentrated under reduce pressure. The crude product was recrystallized from EtOAc, yielding colorless prism (9.19 mg, 12%). mp 211.0–212.0 °C (dec). 1H NMR (400 MHz, DMSO-d6, δ): 10.97 (s br, 1H), 8.08 (s br, 2H), 2.49 (s, 4H).
Synthesis of N-(ethoxycarbonylacetyl)glycine ethyl ester.22
A solution of glycine ethyl ester hydrogen chloride (6.98 g, 50 mmol), trimethylamine (6.78 mL, 50 mmol) and ethyl hydrogen malonate (5.90 g, 50 mmol) in CH2Cl2 (100 mL) was cooled to 0 °C. A solution of DCC (10.32 g, 50 mmol) in CH2Cl2 (35 mL) was then added drop-by-drop and stirred for 1.5 h at 0 °C; then, the reaction mixture was warmed to room temperature and stirred for 3 h. The white precipitate was filtered to remove N,N’-dicyclohexylurea, washed with water, and dried over Na2SO4. The extract was filtered and concentrated under reduced pressure. The crude oil was solidified from a mixture of acetone 5 mL and n-hexane 2 mL to isolate desired product, yielding colorless needle (8.51 g, 78%). 1H NMR (400 MHz, CDCl3, δ): 4.22 (q, 2H, J = 7.3 Hz), 4.22 (q, 2H, J = 7.3 Hz), 4.10 (s, 2H, H1a), 3.37 (s, 2H), 1.30 (t, 3H, J = 7.3 Hz), 1.30 (t, 3H, J = 7.3 Hz).
Synthesis of 3-diazopyrrolidine-2,4-dione.22,23
N-(Ethoxycarbonylacetyl)glycine (8.47 g, 39 mmol) was dissolved in benzene at 60 °C. A 28% sodium methoxide MeOH solution (8.00 mL, 40 mmol) was added drop-by-drop to the mixture, refluxed for 1.5 h, and cooled to room temperature. The reaction mixture was extracted with water and treated with conc. HCl(aq). The precipitate was filtered and washed with water. The resultant solid was dissolved in dry MeCN and refluxed overnight. The reaction mixture was then cooled to room temperature and concentrated under reduced pressure. p-Toluensulfonyl azide (3.94 g, 20 mmol) was added to the residue and cooled to 0 °C, followed by the drop-by-drop addition of triethylamine (2.77 mL, 20 mmol) at 0 °C for 30 min. After 30 min, the reaction mixture was stirred at room temperature for 1 h. The solution was then evaporated to give a red gum. The residue was washed with CCl4, extracted with CHCl3, dried over Na2SO4, filtered, and evaporated. The crude product was purified by column chromatography (EtOAc), yielding yellow oil (1.72 g, 76%). 1H NMR (400 MHz, CDCl3, δ): 5.01 (s br, 1H), 3.95 (s, 2H).
Synthesis of 3-tert-butoxy-4-hydroxy-2(5H)-pyrrol-2-one.23
A solution of 3-diazopyrrolidine-2,4-dione (1.63 g, 13 mmol) in tert-butyl alcohol (100 mL) put into a sealed vessel, was added rhodium(II) acetate (15 mg), and heated at 150 °C for 2 h. The reaction mixture was evaporated, purified by column chromatography (15% MeOH-CHCl3) and recrystallized from dioxane, yielding colorless needles (622 mg, 28%). 1H NMR (400 MHz, CD3OD, δ): 3.87 (s, 2H), 1.31 (s, 9H).
Synthesis of 3,4-dihydroxy-2(5H)-pyrrol-2-one (3).23
3-tert-Butoxy-4-hydroxy-2(5H)-pyrrol-2-one (431 mg, 2.5 mmol) was dissolved in cooled trifluoroacetic acid (5 mL) and stirred at room temperature. The reaction mixture was added CHCl3 (5 mL) and concentrated under reduce pressure. The precipitated solid was washed with acetone and filtered to yield the desired product, yielding colorless solid (267 mg, 93%). mp 248.0–249.0 °C (dec). 1H NMR (400 MHz, CD3OD, δ): 3.87 (s, 2H).
Synthesis of N-ethyl(ethoxycarbonylacetyl)glycine ethyl ester (8a).
N-Ethyl glycine ethyl ester (2.36 g, 18 mmol) in CH2Cl2 (60 mL) was combined with a solution of ethyl hydrogen malonate (2.13 g, 18 mmol) and DCC (3.71 g, 18 mmol) in CH2Cl2 (20 mL), according to general procedure 1, yielding 8a as a colorless oil (3.41 g, 77%). 1H NMR (400 MHz, CDCl3, δ, mixture of rotamers: a, major; b, minor): 4.22 (q, 2H, J = 7.3 Hz, H3), 4.19 (q, 2H, J = 7.3 Hz, H8), 4.08 (s, 2H, H1a), 4.06 (s, 2H, H1b), 3.51 (s, 2H, H6a), 3.48 (q, 3H, J = 7.3 Hz, H10b), 3.43 (q, J = 7.3 Hz, H10a), 3.39 (s, 2H, H6b), 1.30 (t, 6H, J = 7.3 Hz, H4b, H9b), 1.28 (t, 6H, J = 7.3 Hz, H4a, H9a), 1.21 (t, 3H, J = 7.3 Hz, H11a), 1.12 (t, 3H, J = 7.3 Hz, H11b). 13C NMR (100 MHz, CDCl3, δ, mixture of rotamers: a, major; b, minor): 169.00 (C7), 167.36 (C2), 166.25 (C5), 61.79 (C3b, C8b), 61.53 (C3a, C8a), 61.22 (C3a, C8a), 49.85 (C1b), 47.04 (C1a), 44.30 (C10a), 42.52 (C10b), 41.53 (C6b), 40.91 (C6a), 14.15 (C3a, C8a), 14.12 (C3a, C8a), 14.09 (C3b, C8b), 13.64 (C11a), 12.35 (C11b). MS (EI+, m/z): 245.2, 200.1, 172.1, 130.1, 58.1. HRMS-EI (m/z): calcd for C11H19NO5, 245.1263; found, 245.1263.
Synthesis of 3-diazo-1-ethylpyrrolidine-2,4-dione (10a).
Compound 8a (3.19 g, 13 mmol) in benzene (80 mL) was combined with a 28% sodium methoxide MeOH solution (2.60 mL, 13 mmol), p-toluenesulfonyl azide (2.17 g, 11 mmol), and triethylamine (1.52 mL, 11 mmol), according to general procedure 2, yielding 10a as yellow oil (1.28 g, 71%). 1H NMR (400 MHz, CDCl3, δ): 3.78 (s, 2H, H5), 3.45 (q, 2H, J = 7.3 Hz, H6), 1.13 (t, 3H, J = 7.3 Hz, H7). 13C NMR (100 MHz, CDCl3, δ): 185.94 (C4), 161.33 (C2), 65.77 (C3), 53.77 (C5), 37.44 (C6), 12.72 (C7). MS (EI+, m/z): 153.0, 97.0, 57.0, 42.0, 18.0. HRMS-EI (m/z): calcd for C6H7N3O2, 153.1410; found, 153.0539.
Synthesis of 3-tert-butoxy-1-ethyl-4-hydroxy-2(5H)-pyrrol-2-one (11a).
Compound 10a (1.23 g, 8.0 mmol) in tert-butyl alcohol (80 mL) was combined with rhodium(II) acetate (8.00 mg, cat), according to general procedure 3, yielding 11a as colorless needles (631 mg, 40%). mp 144.5–146.5 °C (sublimation). 1H NMR (400 MHz, CD3OD, δ): 3.82 (s, 2H, H5), 3.39 (q, 2H, J = 7.3 Hz, H6), 1.30 (s, 9H, H9), 1.13 (t, 3H, J = 7.3 Hz, H7). 13C NMR (100 MHz, CD3OD, δ): 173.02 (C2), 157.74 (C4), 121.80 (C3), 82.24 (C8), 47.45 (C5), 37.85 (C6), 28.84 (C9), 13.70 (C7). MS (FAB+, m/z): 200.1, 144.1. HRMS-FAB (m/z): calcd for C10H18NO3, 200.1281; found, 200.1286.
Synthesis of 1-ethyl-3,4-dihydroxy-2(5H)-pyrrol-2-one (4).
Compound 12a (199 mg, 1.0 mol) in dioxane (3 mL) was combined with p-toluenesulfonic acid (2.00 mg, 12 mmol), according to general procedure 4, yielding 4 as colorless needles (62.8 g, 44%). mp 141.0–142.0 °C (dec). 1H NMR (400 MHz, DMSO-d6, δ): 9.84 (s br, 1H, OH4), 7.95 (s br, 1H, OH3), 3.62 (s, 2H, H5), 3.24 (q, 2H, J = 7.3 Hz, H6), 1.00 (t, 3H, J = 7.3 Hz, H7). 13C NMR (100 MHz, DMSO-d6, δ): 168.39 (C2), 143.17 (C4), 121.62 (C3), 45.71 (C5), 35.88 (C6), 13.40 (C7).
Synthesis of N-cyanomethyl(ethoxycarbonylacetyl)glycine ethyl ester (8b).
N-Cyanomethyl glycine ethyl ester (2.32 g, 13 mmol) in CH2Cl2 (40 mL) was combined with a solution of ethyl hydrogen malonate (1.77 mL, 15 mmol) and DCC (3.09 g, 15 mmol) in CH2Cl2 (10 mL), according to general procedure 1, yielding 8b as a colorless oil (3.01 g, 90%). 1H NMR (400 MHz, CDCl3, δ, mixture of rotamers: a, major; b, minor): 4.47 (s, 2H, H10b), 4.45 (s, 2H, H10a), 4.28 (q, 2H, J = 7.3 Hz, H3), 4.27 (s, 2H, H1b), 4.24 (s, 2H, H1a), 4.21 (q, 2H, J = 7.3 Hz, H8), 3.60 (s, 2H, H6b), 3.46 (s, 2H, H6a), 1.33 (t, 6H, J = 7.3 Hz, H4b, H9b), 1.29 (t, 6H, J = 7.3 Hz, H4a, H9a). 13C NMR (100 MHz, CDCl3, δ, mixture of rotamers: a, major; b, minor): 167.94 (C7b), 167.66 (C7a), 166.23 (C2a), 165.70 (C2b), 114.69 (C11a), 114.34 (C11b), 62.31 (C3a, C8a), 62.11 (C3b, C8b), 61.93 (C3a, C8a), 61.76 (C3b, C8b), 49.61 (C1a), 47.19 (C1b), 41.13 (C10b), 42.52 (C10b), 41.53 (C6b), 40.91 (C6a), 37.72 (C6b), 34.61 (C6a), 14.02 (C3b, C8b), 13.96 (C3a, C8a). MS (EI+, m/z): 256.0, 142.0, 115.0, 69.0, 29.0. HRMS-EI (m/z): calcd for C11H16N2O5, 256.1059; found, 256.1058.
Synthesis of 3-diazo-1-cyanomethylpyrrolidine-2,4-dione (10b).
Compound 8b (3.08 g, 12 mmol) in benzene (60 mL) was combined with a 28% sodium methoxide MeOH solution (3.00 mL, 15 mmol), p-toluenesulfonyl azide (1.58 g, 8.0 mol), and trimethylamine (1.11 mL, 8.0 mmol), according to general procedure 2, yielding 10b as yellow oil (426 mg, 23%). 1H NMR (400 MHz, CDCl3, δ): 4.43 (s, 2H, H6), 4.00 (s, 2H, H5). 13C NMR (100 MHz, CDCl3, δ): 183.30 (C4), 161.95 (C2), 113.61 (C7), 66.29 (C3), 30.55 (C6). MS (EI+, m/z): 163.9. HRMS-EI (m/z): calcd for C6H4N4O2, 164.0334; found, 164.0334.
Synthesis of 3-tert-butoxy-1-cyanomethyl-4-hydroxy-2(5H)-pyrrol-2-one (11b).
Compound 10b (410 mg, 2.5 mmol) in tert-butyl alcohol (50 mL) was combined with rhodium(II) acetate (5.00 mg, cat), according to general procedure 3, yielding 11b as colorless crystals (205 mg, 39%). mp 175.0–178.0 °C (sublimation). 1H NMR (400 MHz, DMSO-d6, δ): 10.89 (s br, 1H, OH4), 4.38 (s, 2H, H6), 3.84 (s, 2H, H5), 1.21 (s, 9H, H9). 13C NMR (100 MHz, DMSO-d6, δ): 170.16 (C2), 157.66 (C4), 119.62 (C3), 116.71 (C7), 79.95 (C8), 46.83 (C5), 30.45 (C6), 28.33 (C9). MS (FAB+, m/z): 155.0.
Synthesis of 1-cyanomethyl-3,4-dihydroxy-2(5H)-pyrrol-2-one (5).
Compound 12b (126 mg, 0.60 mol) in dioxane (8.0 mL) was combined with p-toluenesulfonic acid (2.00 mg, 12 mmol), according to general procedure 4, yielding 5 as a colorless solid (50.3 mg, 54%). mp 145.0–145.5 °C (dec). 1H NMR (400 MHz, DMSO-d6, δ): 10.36 (s br, 1H, OH4), 8.22 (s br, 1H, OH3), 4.39 (s, 2H, H5), 3.73 (s, 2H, H6). 13C NMR (100 MHz, DMSO-d6, δ): 169.09 (C2), 143.17 (C4), 121.19 (C3), 116.66 (C7), 46.70 (C5), 30.39 (C6).
Synthesis of 1-acetyl-3-tert-butoxy-4-hydroxy-2(5H)-pyrrol-2-one (11c).
To a solution of 1-acetyl-3-diazopyrrolidine-2,4-dione (10c, 1.00 g, 6.0 mmol) in tert-butyl alcohol (30 mL) at 60 °C was added rhodium(II) acetate (3.00 mg, cat), and the resulting mixture was refluxed overnight. The reaction mixture was then evaporated, purified by column chromatography (10% MeOH-CHCl3), and washed with acetone to give 11c as a pale yellow solid (843 mg, 90%). Recrystallization from MeOH with activated carbon yielded colorless needles. mp 163.5–165.5 °C (sublimation). 1H NMR (400 MHz, DMSO-d6, δ): 11.56 (s br, 1H, OH4), 4.05 (s, 2H, H5), 2.36 (s, 3H, H7), 1.23 (s, 9H, H9). 13C NMR (100 MHz, DMSO-d6, δ): 168.45 (C6), 168.07 (C2), 160.97 (C4), 119.53 (C3), 80.42 (C8), 44.10 (C5), 28.32 (C7), 24.19 (C9). HRMS-FAB (m/z): [M + H]+ calcd for C10H15NO4, 214.1074; found, 214.1077.
Synthesis of 1-acetyl-3,4-dihydroxy-2(5H)-pyrrol-2-one (6).
To a solution of 11c (213 mg, 1.0 mmol) in dioxane (10 mL) was added p-toluenesulfonic acid (5.00 mg, 25 µmol) at 60 °C, and the resulting mixture was refluxed overnight. The reaction mixture was then slowly cooled to room temperature, and the precipitated solid was filtered to yield desired product 6 as a colorless solid (123 mg, 78%). mp 233.5–235.0 °C (dec). 1H NMR (400 MHz, DMSO-d6, δ): 11.08 (s br, 1H, OH4), 8.40 (s br, 1H, OH3), 3.96 (s, 2H, H5), 2.37 (s, 3H, H7). 13C NMR (100 MHz, DMSO-d6, δ): 167.96 (C6), 167.17 (C2), 149.85 (C4), 121.27 (C3), 43.90 (C5), 24.17 (C7). HRMS-EI (m/z): M+ calcd for C6H7NO4, 157.0375; found, 157.0375.
Kinetics
Kinetics were determined by measuring the disappearance of absorbance at 429 nm under pseudo-first-order conditions at 25 °C. Aliquots of AscH2 analogs (final concentrations: 25, 30, 35, 40, 45, and 50 µM) in deaerated methanol were mixed with the galvinoxyl radical solution (final concentration: 2.5 µM) in deaerated methanol. Changes in the UV–vis spectra following this reaction were monitored using a UNISOKU RSP-1000-02NM spectrophotometer (Osaka, Japan). Radical scavenging rates were determined by monitoring the absorbance change at 429 nm due to decrease in the concentration of the galvinoxyl radical. The reaction rate for the decrease of the concentration of the galvinoxyl radical was obtained from the initial rates method as the rate of decrease in the concentration of the galvinoxyl radical was very fast. A plot of lnki versus ln[AscH2 analogs] yielded the reaction order from the slope and the reaction rate from the y-intercept as ln k.
References
1. B. Halliwell and J. M. Gutteridge, ‘Free Radicals in Biology and Medicine’, 3rd ed., Oxford University Press, Oxford, 1999.
2. A. Popa-Wagner, S. Mitran, S. Sivanesan, E. Chang, and A. M. Buga, Oxid. Med. Cell. Longevity, 2013, 2013, 963520.
3. D. P. Jones and Y. M. Go, Diabetes Obes. Metab., 2010, 12, 116. CrossRef
4. M. A. Aon, S. Cortassa, and B. O’Rouke, Biochim. Biophys. Acta, 2010, 1797, 865.
5. B. Poljsak, D. Suput, and I. Mikisav, Oxid. Med. Cell. Longevity, 2013, 2013, 966792.
6. D. M. Okamura and S. Pennathur, Redox Biology, 2015, 6, 495. CrossRef
7. M. Mittal, M. R. Siddiqui, K. Tran, S. P. Reddy, and A. B. Malik, Antioxid. Redox Signal., 2014, 20, 1126. CrossRef
8. M. Barontini, R. Bernini, I. Carastro, P. Gentili, and A. Romani, New J. Chem., 2014, 38, 809. CrossRef
9. K. Inami, Y. Iizuka, M. Furukawa, I. Nakanishi, K. Ohkubo, K. Fukuhara, S. Fukuzumi, and M. Mochizuki, Bioorg. Med. Chem., 2012, 20, 4049. CrossRef
10. S. Selvaraj, A. Mohan, S. Narayanan, S. Sethuraman, and U. M. Krishnan, J. Med. Chem., 2013, 56, 970. CrossRef
11. J. Takebayashi, A. Tai, E. Gohda, and I. Yamamoto, Biol. Pharm. Bull., 2006, 29, 766. CrossRef
12. R. Han, L. Liu, J. Li, G. Du, and J. Chen, Appl. Microbiol. Biotechnol., 2012, 95, 313. CrossRef
13. W.-Y. Huang, P.-C. Lee, L.-K. Huang, L.-P. Lu, and W. C. Liao, Bioorg. Med. Chem. Lett., 2013, 23, 1583. CrossRef
14. N. Kharrat, I. Aissa, M. Sghaier, M. Bouaziz, M. Sellami, D. Laouini, and Y. Gargouri, J. Agric. Food Chem., 2014, 62, 9118. CrossRef
15. F. Shahidi and Y. Zhong, J. Agric. Food Chem., 2011, 59, 3499. CrossRef
16. R. Amorati, G. F. Pedulli, and L. Valgimigli, Org. Biomol. Chem., 2011, 9, 3792. CrossRef
17. J. Du, J. J. Cullen, and G. R. Buettner, Biochim. Biophys. Acta, 2012, 1826, 443.
18. J. Tsuchiya, T. Yamada, E. Niki, and Y. Kamiya, Bull. Chem. Soc. Jpn., 1985, 58, 326. CrossRef
19. R. Ramage, G. J. Griffiths, and F. E. Shutt, J. Chem. Soc., Perkin Trans. 1, 1984, 1531. CrossRef
20. R. Ramage, G. J. Griffiths, and F. E. Shutt, J. Chem. Soc., Perkin Trans. 1, 1984, 1539. CrossRef
21. G. Hesse and H. Friedrich, Liebigs Ann. Chem., 1970, 736, 134. CrossRef
22. G. Lowe and H. W. Yeung, J. Chem. Soc., Perkin Trans. 1, 1973, 2907. CrossRef
23. H.-D. Stachel, H. Poschenrieder, J. Redlin, J. Schachtner, and K. Zeitler, Liebigs Ann. Chem., 1994, 2, 129. CrossRef
24. Y.-C. Jeong and M. G. Moloney, Synlett, 2009, 2487.
25. J. L. Wood, B. M. Stoltz, H.-J. Dietrich, D. A. Pflum, and D. T. Petsch, J. Am. Chem. Soc., 1997, 119, 9641. CrossRef
26. C. Mannich and R. Kuphal, Chem. Ber., 1912, 45, 314. CrossRef
27. W. R. R. Harker, P. M. Delany, M. Simms, M. J. Tozer, and J. P. A. Harrity, Tetrahedron, 2013, 69, 1546. CrossRef
28. N. V. Rostovskii, M. S. Novikov, A. F. Khlebnikov, S. M. Korneev, and D. S. Yufit, Org. Biomol. Chem., 2013, 11, 5535. CrossRef
29. K. Inami, M. Suzuki, A. Shimizu, M, Furukawa, M. Morita, and M. Mochizuki, RSC Adv., 2014, 4, 43882. CrossRef
30. I. Nakanishi, T. Kawashima, K. Okubo, H. Kanazawa, K. Inami, M. Mochizuki, K. Fukuhara, H. Okuda, T. Ozawa, S. Itoh, S. Fukuzumi, and N. Ikota, Org. Biomol. Chem., 2005, 3, 626. CrossRef
31. K. J. Hall, T. I. Quickenden, and D. W. Watts, J. Chem. Educ., 1976, 53, 493. CrossRef
32. J. Casado, M. A. Lopez-Quintela, and F. M. Lorenzo-Barral, J. Chem. Educ., 1986, 63, 450. CrossRef
33. L. Valgimigli and D. A. Pratt, Acc. Chem. Res., 2015, 48, 966. CrossRef