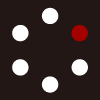
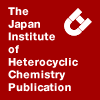
HETEROCYCLES
An International Journal for Reviews and Communications in Heterocyclic ChemistryWeb Edition ISSN: 1881-0942
Published online by The Japan Institute of Heterocyclic Chemistry
e-Journal
Full Text HTML
Received, 16th November, 2015, Accepted, 27th January, 2016, Published online, 5th February, 2016.
DOI: 10.3987/COM-15-13368
■ Facile and Efficient Synthesis of 2-Aminoquinoline Derivatives Reduced by Zn/AcOH
Guolan Dou,* Deming Wang, and Daqing Shi
School of Safety Engineering, China University of Mining & Technology, Xuzhou 221116, China
Abstract
In this paper, a simple and efficient method for one-pot synthesis of 2-aminoquinolines was accomplished in good yields via reductive cyclization of nitro and cyano groups mediated by zinc/acetic acid is reported.Nitrogen-containing heterocycles are abundant in nature and are of great significance to life because their structural subunits exist in many natural products. Quinoline derivatives occur in a large number of natural products1 and drug-like compounds.2 2-Aminoquinoline derivatives are especially important because of their pharmaceutical activities and applications, including antiprotozals,3 antidepressants,4 antihypertensives,5 anti-HIV,6 anti-Alzheimer disease,7 melanin-concentrating hormone receptor antagonists8 and Src kinase inhibitors.9
There has been a large amount of effort devoted to developing efficient methods for the preparation of substituted 2-aminoquinolines. Srinivasan et al.10 reported the synthesis of 2-aminoquinoline-3-carbonitrile from the reaction of arylidenemalononitriles with enaminone. Wilson et al.11 described the synthesis of 2-aminoquinolines using microwave-assisted synthesis. Basic condensations of aromatic ketones with (dimethylamino)propionitrile,12 Frielander’s approach13 and nucleophilic substitution of chloroquinoline14 have also been used to synthesize 2-aminoquinolines. However, these methods have disadvantages such as forcing conditions, unsatisfactory yields, long reaction times, high temperatures, complex manipulations, and commercially unavailable starting materials. Zhou et al.15,16 obtained 2-aminoquinoline-3-carboxylic acid derivatives rapidly in good yields by treating nitrocyano olefins with a low-valent titanium reagent in dry THF at room temperature. The same group also reported the intramolecular reductive coupling of cyano and nitro groups induced by SmI2 to produce 2-aminoquinoline in good yields.17 However, the requirement of anhydrous and/or anaerobic conditions detracts from the usefulness of these methods. Coupling between cyano and nitro groups can also be achieved using tin dichloride,18 however, the reaction time is long, involving heating under reflux for 6 h. Reductive coupling of cyano and nitro groups can be achieved using iron and acetic acid, but yields are generally moderate.19-21 Therefore, more efficient methods to prepare these kinds of compounds with mild reaction conditions and high yields are desirable. The zinc-acetic acid (Zn/AcOH) couple is a well-known reducing reagent, capable of a wide range of reactions,22,23 among which reductions of nitro, carbonyl and activated alkenes are the most common. We found that 2-amino-3-cyanoquinoline can be obtained by Zn/AcOH-mediated cyano–nitrile coupling in a study optimizing the synthesis of substituted quinoline-1-oxides.24 In this study, we further explore the potential of Zn/AcOH-mediated cyano–nitrile coupling and describe a convenient one-pot reductive cyclization protocol for the synthesis of 2-aminoquinolines.
Isopropyl-2-cyano-3-(2-nitrophenyl)acrylate (1a) was used as a model substrate to optimize the experimental conditions for the proposed reductive cyclization reaction (Scheme 1). The results are summarized in Table 1.
The ratio of 1a to Zn/AcOH was evaluated between the range of 1:1 and 1:5. The yield of 2-aminoquinoline 2a increased as the ratio of 1a to Zn/AcOH was increased from 1:1 to 1:4 (Table 1, entries 1–4). Further increasing this ratio to 1:5 resulted in a decrease in yield compared with that obtained using a ratio of 1:4 (Table 1, entry 5). Therefore, 1:4 was chosen for all further reactions. Reaction temperature was investigated next; heating to reflux resulted in the highest yield (Table 1, entries 6–8). Solvent screening revealed that EtOH was preferable to the other solvents tested (Table 1, entries 8–11). We concluded that the optimal conditions are 4 equivalents of Zn/AcOH in EtOH heated at reflux.
With the optimized conditions in hand, we then examined the applicability of the process for the synthesis of other 2-aminoquinolines (Scheme 2).
We found that the method was applicable to a broad selection of substrates (Table 2), with a range of groups in the R position tolerated including esters and substituted phenyls. Good or acceptable yields were obtained when a 5-Cl-, 4,5-OCH2O- or 4,5-dimethoxy-substituted phenyl ring was present (entries 5–10). All the products were characterized by IR, HRMS and 1H NMR spectroscopy.
In summary, a series of 2-aminoquinolines were synthesized by Zn/AcOH reaction of nitro and cyano groups. This protocol is advantageous compared with other published methods because it uses readily accessible materials, requires simple manipulations, and allows facile isolation of products via simple recrystallization.
EXPERIMENTAL
Commercial solvents and reagents were used as received. Melting points are uncorrected. IR spectra were recorded on Varian F-1000 spectrometer in KBr with absorptions in cm-1. 1H NMR was determined on Varian-300 MHz spectrometer in DMSO-d6 solution. J values are in Hz. Chemical shifts are expressed in ppm downfield from internal standard TMS. MS data were obtained using microma GCT-TOF instrument (EI+). The synthesis of the reactant 1 is according to the report literature.25
General procedure for the synthesis of 2 is represented as follows.
To a solution of 3-(2-nitrophenyl)acrylonitriles 1 (1 mmol) and Zn (4 mmol) in 5 mL of EtOH was added 0.2 mL AcOH, then the mixture was stirred at reflux for 1-2 h. After this period, the TLC analysis of the mixture showed the reaction to be completed. The reaction mixture was quenched with 30 mL of water and extracted with CHCl3 (3 × 20 mL). The combined extracts were washed with water (3 × 20 mL) and dried over anhydrous Na2SO4. After evaporation of the solvent under reduced pressure, the crude product was purified by recrystallization from 95% EtOH.
Isopropyl 2-aminoquinoline-3-carboxylate (2a): white solid, mp 156-158 oC. IR (KBr) ν: 3417, 3146, 2831, 1669, 1611, 1515, 1446, 1409, 1267, 1163, 986, 828, 715, 688 cm-1. 1H NMR (300 MHz, DMSO-d6) (δ, ppm): 1.36 (6H, d, J = 6.0 Hz, 2×CH3), 5.18 (1H, m, OCH), 7.19 (3H, br s, NH2+ ArH), 7.48 (1H, d, J = 8.4 Hz, ArH), 7.61 (1H, t, J = 7.8 Hz, ArH) 7.84 (1H, d, J = 8.1 Hz, ArH), 8.71 (1H, s, ArH). HRMS [Found: m/z 230.1058 (M+), calcd for C13H14N2O2: M, 230.1055]. Anal. Calcd for C13H14N2O2: C, 67.81; H, 6.13; N, 12.17. Found: C, 67.71; H, 6.29; N, 12.28%.
Methyl 2-aminoquinoline-3-carboxylate (2b): white solid, mp 120-122 oC. IR (KBr) ν: 3427, 3157, 2956, 1701, 1631, 1565, 1437, 1291, 1203, 1132, 1078, 954, 800, 748, 597 cm-1. 1H NMR (300 MHz, DMSO-d6) (δ, ppm): 3.88 (3H, s, OCH3), 7.19 (3H, br s, NH2+ ArH), 7.48 (1H, s, ArH), 7.62 (1H, s, ArH), 7.83 (1H, s, ArH), 8.75 (1H, s, ArH). HRMS [Found: m/z 202.0743 (M+), calcd for C11H10N2O2: M, 202.0742]. Anal. Calcd for C11H10N2O2: C, 65.34; H, 4.98. N, 13.85; Found: C, 65.45; H, 4.87; N, 13.97%.
3-(4-Chlorophenyl)quinolin-2-amine (2c): white solid, mp 206-208 oC. IR (KBr) ν: 3438, 3126, 2757, 1643, 1568, 1496, 1434, 1200, 1126, 1089, 1016, 906, 838, 755, 688 cm-1. 1H NMR (300 MHz, DMSO-d6) (δ, ppm): 6.09 (2H, s, NH2), 7.18 (1H, m, ArH), 7.51 (5H, m, ArH), 7.68 (2H, d, J = 7.8 Hz, ArH), 7.82 (1H, s, ArH). HRMS [Found: m/z 254.0612 (M+), calcd for C15H11N235Cl: M, 254.0611]. Anal. Calcd for C15H11ClN2: C, 70.73; H, 4.35; N, 11.00. Found: C, 70.61; H, 4.48; N, 11.15%.
Ethyl 2-aminoquinoline-3-carboxylate (2d): white solid, mp 124-126 oC. IR (KBr) ν: 3418, 3275, 3148, 2979, 1697, 1626, 1566, 1482, 1439, 1290, 1204, 1137, 1083, 1015, 960, 802, 750 cm-1. 1H NMR (300 MHz, DMSO-d6) (δ, ppm): 1.34 (3H, t, J = 4.2 Hz, CH3), 4.34 (2H, m, OCH2), 7.19 (3H, br s, NH2+ ArH), 7.48 (1H, d, J = 8.1 Hz, ArH), 7.61 (1H, t, J = 6.0 Hz, ArH), 7.83 (1H, d, J = 7.2 Hz, ArH), 8.73 (1H, s, ArH). HRMS [Found: m/z 216.0901 (M+), calcd for C12H12N2O2: M, 216.0899]. Anal. Calcd for C12H12N2O2: C, 66.65; H, 5.59; N, 12.96. Found: C, 66.78; H, 5.45; N, 12.84%.
Methyl 2-amino-6-chloroquinoline-3-carboxylate (2e): white solid, mp 184-186 oC. IR (KBr) ν: 3427, 3268, 3127, 2900, 1705, 1628, 1561, 1483, 1440, 1362, 1273, 1185, 1082, 930, 822, 792, 667 cm-1. 1H NMR (300 MHz, DMSO-d6) (δ, ppm): 3.88 (3H, s, OCH3), 7.30 (2H, s, NH2), 7.47 (1H, d, J = 8.7 Hz, ArH), 7.60 (1H, d, J = 7.8 Hz, ArH), 7.97 (1H, s, ArH), 8.75 (1H, s, ArH). HRMS [Found: m/z 236.0351 (M+), calcd for C11H9N2O235Cl: M, 236.0353]. Anal. Calcd for C11H9ClN2O2: C, 55.83; H, 3.83; N, 11.84. Found: C, 55.96; H, 3.71; N, 11.72%.
Ethyl 2-amino-6-chloroquinoline-3-carboxylate (2f): white solid, mp 186-188 oC. IR (KBr) ν: 3422, 3274, 3141, 2993, 1701, 1633, 1563, 1490, 1360, 1277, 1191, 1086, 963, 827, 798, 696, 668 cm-1. 1H NMR (300 MHz, DMSO-d6) (δ, ppm): 1.34 (3H, t, J = 7.2 Hz, CH3), 4.34 (2H, m, OCH2), 7.32 (2H, s, NH2), 7.46 (1H, d, J = 9.0 Hz, ArH), 7.59 (1H, dd, J1 = 2.1 Hz, J2 = 9.0 Hz, ArH), 7.98 (1H, s, ArH), 8.74 (1H, s, ArH). HRMS [Found: m/z 250.0508 (M+), calcd for C12H11N2O235Cl: M, 250.0509]. Anal. Calcd for C12H11ClN2O2: C, 57.49; H, 4.42; N, 11.17. Found: C, 57.60; H, 4.37; N, 11.31%.
Isopropyl 2-amino-6-chloroquinoline-3-carboxylate (2g): white solid, mp 206-208 oC. IR (KBr) ν: 3423, 3274, 3149, 2974, 1700, 1636, 1560, 1486, 1412, 1276, 1238, 1190, 1070, 820, 799, 668 cm-1. 1H NMR (300 MHz, DMSO-d6) (δ, ppm): 1.34 (6H, d, J = 6.0 Hz, 2×CH3), 5.16 (1H, m, OCH), 7.32 (2H, s, NH2), 7.47 (1H, d, J = 9.0 Hz, ArH), 7.60 (1H, dd, J1 = 2.4 Hz, J2 = 9.0 Hz, ArH), 7.99 (1H, d, J = 2.1 Hz, ArH), 8.72 (1H, s, ArH). HRMS [Found: m/z 264.0663 (M+), calcd for C13H13N2O235Cl: M, 264.0666]. Anal. Calcd for C13H13ClN2O2: C, 58.99; H, 4.95; N, 10.58. Found: C, 58.89; H, 5.06; N, 10.69%.
6,7-Dimethoxy-3-phenylquinolin-2-amine (2h): white solid, mp 204-206 oC. IR (KBr) ν: 3451, 3294, 3164, 2999, 1637, 1624, 1500, 1460, 1395, 1247, 1166, 1013, 849, 785, 755, 610 cm-1. 1H NMR (300 MHz, DMSO-d6) (δ, ppm): 3.79 (3H, s, OCH3), 3.84 (3H, s, OCH3), 5.61 (2H, s, NH2), 6.94 (1H, s, ArH), 7.13 (1H, s, ArH), 7.40 (1H, s, ArH), 7.48 (4H, d, J = 3.6 Hz, ArH), 7.69 (1H, s, ArH). HRMS [Found: m/z 280.1209 (M+), calcd for C17H16N2O2: M, 280.1212]. Anal. Calcd for C17H16N2O2: C, 72.84; H, 5.75; N, 9.99. Found: C, 72.72; H, 5.88; N, 9.87%.
7-(4-Bromophenyl)-[1,3]dioxolo[4,5-g]quinolin-6-amine (2i): brown solid, mp 192-194 oC. IR (KBr) ν: 3477, 3457, 3161, 2923, 1630, 1602, 1490, 1410, 1226, 1034, 937, 829, 744, 589 cm-1. 1H NMR (300 MHz, DMSO-d6) (δ, ppm): 6.11 (2H, s, OCH2O), 6.31 (2H, s, NH2), 7.00 (1H, s, ArH), 7.19 (1H, s, ArH), 7.44 (2H, d, J = 7.5 Hz, ArH), 7.68 (2H, d, J = 7.5 Hz, ArH), 7.80 (1H, s, ArH). HRMS [Found: m/z 342.0003 (M+), calcd for C16H11N2O279Br: M, 342.0004]. Anal. Calcd for C16H11BrN2O2: C, 56.00; H, 3.23; N, 8.16. Found: C, 56.12; H, 3.11; N, 8.27%.
7-Phenyl-[1,3]dioxolo[4,5-g]quinolin-6-amine (2j): white solid, mp 114-116 oC. IR (KBr) ν: 3445, 3299, 3151, 2892, 1632, 1602, 1485, 1410, 1231, 1122, 1040, 946, 836, 779, 701, 587 cm-1. 1H NMR (300 MHz, DMSO-d6) (δ, ppm): 5.71 (2H, s, NH2), 6.06 (2H, s, OCH2O), 6.92 (1H, s, ArH), 7.12 (1H, s, ArH), 7.45 (5H, m, ArH), 7.68 (1H, s, ArH). HRMS [Found: m/z 264.0898 (M+), calcd for C16H12N2O2: M, 264.0899]. Anal. Calcd for C16H12N2O2: C, 72.72; H, 4.58; N, 10.60. Found: C, 72.84; H, 4.44; N, 10.69%.
ACKNOWLEDGEMENTS
We are grateful to financial support from the Fundamental Research Funds for the Central Universities (2014QNB01).
References
1. (a) A. R. Katritzky and M. Arend, J. Org. Chem., 1998, 63, 9989; CrossRef (b) J. P. Michael, Nat. Prod. Rep., 1997, 14, 605; CrossRef (c) Y. Morimoto, F. Matsuda, and H. Shirahama, Synlett, 1991, 202. CrossRef
2. S. F. Campbell, J. D. Hardstone, and M. J. Palmer, J. Med. Chem., 1988, 31, 1031. CrossRef
3. D. G. Markees, V. C. Dewey, and G. W. Kidder, J. Med. Chem., 1970, 13, 324. CrossRef
4. (a) A. A. Alhaider, M. A. Abdelkader, and E. J. Lien, J. Med. Chem., 1985, 28, 1398; CrossRef (b) K. Hino, Y. Nagai, and H. Uno, Chem. Pharm. Bull., 1987, 35, 2819. CrossRef
5. S. F. Campbell, J. D. Hardstone, and M. J. Palmer, J. Med. Chem., 1988, 31, 1031. CrossRef
6. (a) W. D. Wilson, M. Zhao, S. E. Patterson, R. L. Wydra, L. Janda, and L. Strekowski, Med. Chem. Res., 1992, 2, 102; (b) L. Strekowski, J. L. Mokrosz, V. A. Honkan, A. Czarny, M. T. Cegla, R. L. Wydra, S. E. Patterson, and R. F. Schinazi, J. Med. Chem., 1991, 34, 1739. CrossRef
7. G. R. Proctor and A. L. Harvey, Curr. Med. Chem., 2000, 7, 295. CrossRef
8. J. Jiang, P. Lin, M. Hoang, L. Chang, C. Tan, S. Feighner, O. C. Palyha, D. L. Hreniuk, J. Pn, A. W. Sailer, N. R. Morin, D. J. MacNeil, A. D. Howard, L. H. Y. Van der Ploeg, M. T. Goulet, and R. J. Devita, Bioorg. Med. Chem. Lett., 2006, 16, 5275. CrossRef
9. D. H. Boschelli, Y. D. Wang, F. Ye, B. Wu, N. Zhang, M. Dutia, D. W. Powell, A. Wissner, J. M. Weber, and F. Boschelli, J. Med. Chem., 2001, 44, 822. CrossRef
10. A. R. Gholap, K. S. Toti, F. Shirazi, R. Kumari, M. K. Bhat, M. V. Deshpande, and K. V. Srinivasana, Bioorg. Med. Chem., 2007, 15, 6705. CrossRef
11. N. S. Wilson, C. R. Sarko, and G. P. Roth, Tetrahedron Lett., 2002, 43, 581. CrossRef
12. (a) S. Gorbadjiev, Ch. Ivanov, and B. Moskova, Synth. Commun., 1985, 15, 451; CrossRef (b) S. Gorbadjiev, Ch. Ivanov, and B. Moskova, Synth. Commun., 1987, 17, 1363. CrossRef
13. A. A. Sayed, Synth. Commun., 1991, 21, 749. CrossRef
14. F. Korodi, Synth. Commun., 1991, 21, 1841. CrossRef
15. L. H. Zhou, S. J. Tu, D. Q. Shi, and G. Y. Dai, J. Chem. Res. (S), 1998, 398.
16. L. Zhou, S. Tu, D. Shi, G. Dai, and W. Chen, Synthesis, 1998, 851. CrossRef
17. L. Zhou and Y. Zhang, J. Chem. Soc., Perkin Trans. 1, 1998, 2899. CrossRef
18. E. Rajanarendar, M. Nagi Reddy, S. Rama Krishna, K. Rama Murthy, Y. N. Reddy, and M. V. Rajam, Eur. J. Med. Chem., 2012, 55, 273. CrossRef
19. E. Campaigne and G. Randau, J. Heterocycl. Chem., 1971, 8, 111. CrossRef
20. T. H. Althuis, S. B. Kadin, L. J. Czuba, P. F. Moore, and H. H. Hess, J. Med. Chem., 1980, 23, 262. CrossRef
21. Y. D. Wang, D. H. Boschelli, S. Johnson, and E. Honores, Tetrahedron, 2004, 60, 2937. CrossRef
22. See as examples: P. Ham, In Encyclopedia of Reagents for Organic Synthesis, ed. by L. Paquette, John Wiley & Sons, New York, 1995, Vol. 8, pp. 5531-5534 and references therein.
23. J. C. Gauffre, M. G. Dubois, B. Rezzonico, and J. M. Leger, J. Org. Chem., 2002, 67, 4696. CrossRef
24. L. Fu, W. Lin, P. Xu, Y. K. Xi, M. M. Wang, and D. Q. Shi, Tetrahedron, 2012, 68, 7782. CrossRef
25. D. Q. Shi, J. Chen, Q. Y. Zhuang, X. S. Wang, and H. W. Hu, Chinese Chem. Lett., 2003, 14, 1242.