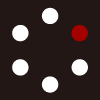
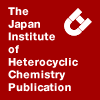
HETEROCYCLES
An International Journal for Reviews and Communications in Heterocyclic ChemistryWeb Edition ISSN: 1881-0942
Published online by The Japan Institute of Heterocyclic Chemistry
e-Journal
Full Text HTML
Received, 18th November, 2015, Accepted, 27th January, 2016, Published online, 5th February, 2016.
DOI: 10.3987/COM-15-13371
■ Catalytic Cyclization of 2,3-Dibromopropionates with Benzyl Azides to Afford 1-Benzyl-1,2,3-triazole-4-carboxylate: The Use of a Nontoxic Bismuth Catalyst
Hong-bin Sun,* Dong Li, Weiping Xie, and Xinlin Deng
Department of Chemistry, Northeastern University of China, Wenhua Road 3-11, Shenyang 110819, China
Abstract
We synthesized 1,2,3-triazoles via the cyclization of 2,3-dibromopropionates with benzyl azides. Bismuth chloride is an efficient catalyst, and the reaction is accelerated by weak bases such as sodium acetate. A variety of functional groups are compatible with this catalytic protocol, and it takes advantage of oxygen stable catalyst and substrates because the reactive dibromides are saturated.To expand the substrate scope of the known transformations is an attractive topic in organic synthesis. The development of the new applications for existing catalysts is an important aspect of catalytic chemistry. In recent years, bismuth compounds have been well developed as catalysts in various transformations for their air-stability, bio-non-toxic nature and their tolerance to small amounts of moisture.1 In previous work, we have reported several applications of bismuth catalyst,2 and now extend our research to bismuth-catalyzed heterocycles of interest to pharmaceutical chemistry. In this paper, we report new development in BiCl3-catalyzed synthesis of 1,2,3-triazoles.
The construction of 1,2,3-triazoles by alkyne-azide cycloaddition (AAC) has long been known as an example of “click chemistry”.3 The copper-promoted AAC (CuAAC) has been applied in various field including synthetic chemistry,4 medicinal chemistry,5 polymer/materials science6 and the life sciences.7 It offers high yield and tolerance to many functional groups. However, the bio-toxicity of copper sometimes limits applications in biological and clinical applications. In addition, the existence of residual copper may cause unpredictable reactions.8 Many efforts have been devoted to eliminate the drawback of metal residual by verifying the catalysts’ construction,9 and tremendous improvement have been achieved in recent years.10 Nevertheless, the development of copper-free synthetic methodology is still appealing.11 Alternatives such as palladium,12 ruthenium,13 silver14 and other metal compounds15 have been developed to replace copper. Furthermore, metal-free catalysts16 and non-catalytic procedures17 are also emerging. In addition, non-alkyne-based substrates have been applied to the synthesis of 1,2,3-triazoles12a,15,18 as an alternative to the alkynes that are usually used in AAC reaction. Here, we investigated the cyclization of non-alkyne-based substrates in the presence of catalytic amounts of BiCl3 to develop new synthetic methods for 1,2,3-triazoles using simple and readily available catalysts and substrates. We found that the 1,2,3-triazoles could be obtained via cyclization of the azides with 2,3-dibromopropionates.
Table 1 shows that the catalytic activity of bismuth chloride is superior to other inorganic metal salt. Of the tested catalysts, bismuth trichloride is the most appropriate although it does not provide the best yield. For example, it is well-known that Cu(I) is active in AAC reaction, but surprisingly, the CuCl has no obvious catalytic activity in this reaction. Both ZnSO4 and ZrOCl2 promote a reaction with slightly lower yields than BiCl3, but their bio-capability is not as good as BiCl3 whose residue is harmless especially if the product is prepared for pharmaceutical use. We also found that DMF is a better solvent than DMSO. Moreover, bases bestow obvious benefits to catalytic reactions because they can absorb the resulting and harmful HBr. It is interesting that the weak base produces better results than the strong one. Sodium acetate is an excellent additive (Table 1, entry 15). The BiCl3 does not act as a simple Lewis acid for the complexation of BiCl3 with Et3N and does not eliminate its catalytic activity19 (Table 1, entry 13). The yield is 68% in the presence of triethylamine in contrast to 34% under base-free conditions (Table 1, entry 12).
Under the optimized conditions, a variety of reactions in which azides react with 2,3-dibromopropionates are carried out using BiCl3 as a catalyst. The results are listed in Table 2. Of note, the acid and base-labile ester group remains undisturbed. As the length of the ester's alkyl chain increases, the yield first increases and then decreases. The electro-deficient 2,3-dibromopropanoic acid is low yield (Table 2, entry 9), and the unhindered substrate 2,3-dibromopropanenitrile offers a standard yield (Table 2, entry 10). No reaction occurred with 2,3-dibromopropanamide—neither did dibromides such as 2,3-dibromopropanol nor styrene dibromide transform to 1,2,3-triazole.
Under our experimental conditions, the reaction exhibits excellent selectivity of 1,4-subtituted 1,2,3-triazole, and 1,5-isomer is detectable in no cases. We suspect that there should be a coordination intermediate generated from the 2,3-dibromo ester with bismuth in the reaction pathway. The crowding of bromo and ester groups restricts the closure of the azide group to the ester. The formation of the 1,5-isomer is then difficult. This hypothesis is based on the benzyl 2,3-dibromopropionate result, which is a steric substrate and gives a low yield of triazole.
Besides the benzyl azide, the substituted benzyl azides are investigated in this catalytic procedure. These reactions provide a variety of 1, 4-substituted 1,2,3-triazoles with excellent tolerance to the tested functionalities. It is clear from Table 2 that few substituents show significant impediment to this catalytic protocol. Halogen atoms as well as alkyl, ether or nitro groups exhibit little influence on the yield. Benzyl azides bearing electron sufficient group such as methoxy and tert-butyl react smoothly with the 2,3-dibromopropionate to give the corresponding triazoles; electron-deficient substrates also work well.
The sterically strict ortho-substituted benzyl azides give average yields (Table 2, entry 12, 13), due to the sufficient distance between the hindered group and the reacting moiety. Only strong electron withdrawing group NO2 gives a slightly decreased yield of 72% (Table 2, entry 17). In another case, triazole is produced efficiently from the cyclization of electro-deficient heterocyclic substrate pyridin-2-ylmethyl azide (Table 2, entry 21). Once again, the reaction employed a non-aromatic substrate methyl azidoacetate 1m and produces triazole 3v in very high yield (Table 2, entry 22).
Furthermore, reactive groups such as cyano survive the bismuth-catalyzed conditions. It is noticeable that the mono cycloaddition product 3n is obtained when the 1,4-bis(azidomethyl)benzene reacted with equivalent amount of dibromide. This means that the selectivity is readily controlled by simply adjusting the amount of the other dibromide reactants.
The reaction mechanism of AAC is clear, but for this reaction of azide with 2,3-dibromopropionate, we do not suggest that the propiolate should be considered as the intermediate according to the current experimental result. The elimination of the first molecule of HBr from the dibromopropionate is accessible with weak base, but it is implausible for the second HBr. A more plausible pathway of the 1,2,3-triazole formation is described below. Moreover, the weak base NaOAc shows better promotion than K2CO3 or Et3N, and the reaction even occurred in the absence of base.
As illustrated in Scheme 1, after the removal of one molecule of HBr, the BiCl3 catalyst reacts with 2-bromoacrylate to form a bismuth complex. This is nucleophilically attacked by the benzyl azide via Michael addition-type reaction. The cycloaddition of 2-bromoacrylate with azide then occurs, and the cyclization-aromatization by HBr elimination generates the 1,2,3-triazole. The ester group plays an important role, and we have found that styrene dibromide does not react with benzyl azide. This explains the product selectivity because bismuth reagents would activate the 1,4-addition of organic azides to 2-bromoacrylates.
In conclusion, we have developed a novel copper free procedure for the synthesis of 1,2,3-triazole with good to high yield. The BiCl3 catalyst and 2,3-dibromopropionate substrates are cheap and readily available, and the reaction conditions are relatively mild and environmental friendly. This cyclization has good selectivity, and is compatible with a variety of functional groups. Moreover, as an alternative to the ordinary alkyne, the 2,3-dibromopropionates are relatively easy to handle.
EXPERIMENTAL
Typical procedure for the synthesis of 1,2,3-triazole: formation of methyl 1-benzyl-1,2,3,-triazole-4-carboxylate (3a): A mixture of 1 mmol benzyl azide, 1.2 mmol methyl 2,3-dibromopropionate, 2.4 mmol NaOAc, and 0.05 mol BiCl3 with 1 mL DMF is stirred at 80 oC for 20 h. After cooling, 10 mg of the mixture is sampled and diluted to 5.0 mL with MeOH as determined by LC. The others are poured into 10 mL water and then extracted with EtOAc. The product is purified on silica gel by eluting with EtOAc and PE (2:3). The product 3a is obtained as a white solid with 78% yield.
ACKNOWLEDGEMENTS
Financial support from the National Natural Science Foundation of China (21003018) and the Fundamental Research Funds for the Central Universities (N130405005) are gratefully acknowledged.
References
1. a) T. Ollevier, Org. Biomol. Chem., 2013, 11, 2740; CrossRef b) J. M. Bothwell, S. W. Krabbe, and R. S. Mohan, Chem. Soc. Rev., 2011, 40, 4649; CrossRef c) J. A. R. Salvador, R. M. A. Pinto, and S. M. Silvestre, Curr. Org. Synth., 2009, 6, 426; CrossRef d) R. Hua, Curr. Org. Synth., 2008, 5, 1; CrossRef e) H. Gaspard-Iloughmane and C. Le Roux, Eur. J. Org. Chem., 2004, 2517; CrossRef f) N. M. Leonard, L. C. Wieland, and R. S. Mohan, Tetrahedron, 2002, 58, 8373. CrossRef
2. a) H.-B. Sun, B. Li, R. Hua, and Y. Yin, Eur. J. Org. Chem., 2006, 4231; CrossRef b) H.-B. Sun, R. Hua, S. Chen, and Y. Yin, Adv. Synth. Catal., 2006, 348, 1919; CrossRef c) H.-B. Sun, R. Hua, and Y. Yin, J. Org. Chem., 2005, 70, 9071. CrossRef
3. a) H. C. Kolb, M. G. Finn, and K. B. Sharpless, Angew. Chem. Int. Ed., 2001, 40, 2004; CrossRef b) J. E. Moses and A. D. Moorhouse, Chem. Soc. Rev., 2007, 36, 1249; CrossRef c) V. D. Bock, H. Hiemstra, and J. H. van Maarseveen, Eur. J. Org. Chem., 2006, 51; CrossRef d) P. Wu, A. K. Feldman, A. K. Nugent, C. J. Hawker, A. Scheel, B. Voit, J. Pyun, J. M. J. Frechet, K. B. Sharpless, and V. V. Fokin, Angew. Chem. Int. Ed., 2004, 43, 3928; CrossRef e) V. V. Rostovtsev, L. G. Green, V. V. Fokin, and K. B. Sharpless, Angew. Chem. Int. Ed., 2002, 41, 2596. CrossRef
4. a) J. E. Hein and V. V. Fokin, Chem. Soc. Rev., 2010, 39, 1302; CrossRef b) P. Anbarasan, D. Yadagiri, and S. Rajasekar, Synthesis, 2014, 46, 3004. CrossRef
5. a) G. C. Tron, T. Pirali, R. A. Billington, P. L. Canonico, G. Sorba, and A. A. Genazzani, Med. Res. Rev., 2008, 28, 278; CrossRef b) H. C. Kolb and K. B. Sharpless, Drug Discov. Today, 2003, 8, 1128; CrossRef c) W. H. Binder and R. Sachsenhofer, Macromol. Rapid Commun., 2008, 29, 952. CrossRef
6. a) W. H. Binder and R. Sachsenhofer, Macromol. Rapid Commun., 2007, 28, 15; CrossRef b) J. F. Lutz, Angew. Chem. Int. Ed., 2007, 46, 1018. CrossRef
7. a) C. W. Tornoe, C. Christensen, and M. Meldal, J. Org. Chem., 2002, 67, 3057; CrossRef b) P.-C. Lin, S.-H. Ueng, M.-C. Tseng, J.-L. Ko, K.-T. Huang, S.-C. Yu, A. K. Adak, Y.-J. Chen, and C.-C. Lin, Angew. Chem. Int. Ed., 2006, 45, 4286; CrossRef c) A. E. Speers, G. C. Adam, and B. F. Cravatt, J. Am. Chem. Soc., 2003, 125, 4686; CrossRef d) F. Amblard, J. H. Cho, and R. F. Schinazi, Chem. Rev., 2009, 109, 4207; CrossRef e) A. H. El-Sagheer and T. Brown, Chem. Soc. Rev., 2010, 39, 1388. CrossRef
8. T. U. Connell, C. Schieber, I. P. Silvestri, J. M. White, S. J. Williams, and P. S. Donnelly, Inorg. Chem., 2014, 53, 6503. CrossRef
9. a) X. Xiong, H. Chen, Z. Tang, and Y. Jiang, RSC Adv., 2014, 4, 9830; b) B. Movassagh and N. Rezaei, Tetrahedron, 2014, 70, 8885; CrossRef c) Y. Jiang, Y. Wang, Q. Han, R. Zhu, and X. Xiong, Chin. J. Org. Chem., 2014, 34, 2068; CrossRef d) Y. Chen, Z.-J. Zhuo, D.-M. Cui, and C. Zhang, J. Organomet. Chem., 2014, 749, 215; CrossRef e) S. Mohammed, A. K. Padala, B. A. Dar, B. Singh, B. Sreedhar, R. A. Vishwakarma, and S. B. Bharate, Tetrahedron, 2012, 68, 8156; CrossRef f) B. S. P. A. Kumar, K. H. V. Reddy, B. Madhav, K. Ramesh, and Y. V. D. Nageswar, Tetrahedron Lett., 2012, 53, 4595; CrossRef g) J. Albadi, M. Keshavarz, F. Shirini, and M. Vafaie-nezhad, Catal. Commun., 2012, 27, 17. CrossRef
10. C. Deraedt, N. Pinaud, and D. Astruc, J. Am. Chem. Soc., 2014, 136, 12092. CrossRef
11. a) J. M. Baskin, J. A. Prescher, S. T. Laughlin, N. J. Agard, P. V. Chang, I. A. Miller, A. Lo, J. A. Codelli, and C. R. Bertozzi, Proc. Natl. Acad. Sci. U. S. A., 2007, 104, 16793; CrossRef b) J. C. Jewett and C. R. Bertozzi, Chem. Soc. Rev., 2010, 39, 1272. CrossRef
12. a) J. Barluenga, C. Valdes, G. Beltran, M. Escribano, and F. Aznar, Angew. Chem. Int. Ed., 2006, 45, 6893; CrossRef b) S. Chuprakov, N. Chernyak, A. S. Dudnik, and V. Gevorgyan, Org. Lett., 2007, 9, 2333. CrossRef
13. a) B. C. Boren, S. Narayan, L. K. Rasmussen, L. Zhang, H. T. Zhao, Z. Y. Lin, G. C. Jia, and V. V. Fokin, J. Am. Chem. Soc., 2008, 130, 8923; CrossRef b) S. Ferrini, J. Z. Chandanshive, S. Lena, M. C. Franchini, G. Giannini, A. Tafi, and M. Taddei, J. Org. Chem., 2015, 80, 25622. CrossRef
14. Y. Ning, N. Wu, H. Yu, P. Liao, X. Li, and X. Bi, Org. Lett., 2015, 17, 2198. CrossRef
15. Y.-C. Wang, Y.-Y. Xie, H.-E. Qu, H.-S. Wang, Y.-M. Pan, and F.-P. Huang, J. Org. Chem., 2014, 79, 4463. CrossRef
16. a) H. Zheng, R. McDonald, and D. G. Hall, Chem. Eur. J., 2010, 16, 5454; CrossRef b) S. W. Kwok, J. R. Fotsing, R. J. Fraser, V. O. Rodionov, and V. V. Fokin, Org. Lett., 2010, 12, 4217. CrossRef
17. a) C. R. Becer, R. Hoogenboom, and U. S. Schubert, Angew. Chem. Int. Ed., 2009, 48, 4900; CrossRef b) M. Sau, C. Rodriguez-Escrich, and M. A. Pericas, Org. Lett., 2011, 13, 5044. CrossRef
18. a) X. Z. Cheng, Y. W. Yang, C. X. Kuang, and Q. Yang, Synthesis, 2011, 2907; CrossRef b) B. Quiclet-Sire and S. Z. Zard, Synthesis, 2005, 3319. CrossRef
19. In our experience, we found that small amount of Et3N would entirely destroy the Lewis acid activity of bismuth as the Lewis acid, even BiCl3 will lose its Lewis acid property in N-containing solvent such as DMF.