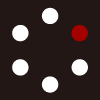
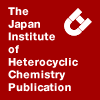
HETEROCYCLES
An International Journal for Reviews and Communications in Heterocyclic ChemistryWeb Edition ISSN: 1881-0942
Published online by The Japan Institute of Heterocyclic Chemistry
e-Journal
Full Text HTML
Received, 1st December, 2015, Accepted, 22nd December, 2015, Published online, 15th January, 2016.
■ Design, Synthesis and Evaluation of an L-Dopa-Derived Macrocyclic Hexaoxazole (6otd) as a G-Quadruplex-Selective Ligand
Takahiro Nakamura, Yue Ma, Keisuke Iida, Terumi Ohtake, Hiroyuki Seimiya, and Kazuo Nagasawa*
Biotechnology and Life Science, Graduate School of Engineering, Tokyo University of Agriculture and Technology, 2-24-16, Naka-cho, Koganei, Tokyo 184-8588, Japan
Abstract
G-Quadruplex (G4) structures in guanine-rich oligonucleotides are involved in replication, transcription and translation processes in cells, and G4 dysfunction is associated with various diseases. Since G4 stabilization is believed to induce growth inhibition, senescence or apoptosis of cancer cells, various G4-stabilizing agents (G4 ligands) have been synthesized, including our recently developed series of macrocyclic polyoxazoles (OTDs). Among OTD derivatives, those bearing side-chain functional groups that interact with phosphate of the DNA backbone show potent G4-stabilizing ability. Here, we report synthesis of a new macrocyclic hexaoxazole bearing two catechol side chains, i.e., D2H4–6M(4)OTD, based upon our previously reported procedure. D2H4–6M(4)OTD showed moderate and selective stabilizing ability towards G4-forming oligonucleotides without altering their topology. It also showed moderate growth-inhibitory activity towards several cancer cell lines.introduction
Nucleic acids containing guanine-rich regions are found throughout the genome1 and transcriptome,2 as well as in non-coding RNAs.3 These guanine-rich regions form non-canonical three-dimensional structures called G-quadruplexes (G4s),4 which comprise stacked planar G-quartets formed from four guanines, held in place by loops consisting of sugar-phosphate backbones. G4 structures are implicated in control of various biological events, including replication,5 transcription6 and translation.7 Also, malformation or collapse of these structures is associated with various diseases, including cancer and neurodegenerative diseases.8 Therefore, there is great interest in G4 ligands, both to elucidate the mechanisms of the biological functions of G4s, and as candidates for treating G4-related diseases. Indeed, some G4-stabilizing agents show significant growth-inhibitory activity against cancer cells in vitro9 as well as in vivo.10 Among these compounds, telomestatin (1), a natural product isolated in 2001, is one of the most potent G4 ligands.11 It also shows significant telomerase-inhibitory activity (IC50: 5 nM). Furthermore, 1 shows specific antitumor activity towards glioma stem cells in vitro as well as in vivo, and hence it is a promising lead compound for novel anticancer agents.12 We have synthesized a series of macrocyclic polyoxazole compounds (OTDs) as telomestatin derivatives.13 In the course of SAR studies, we synthesized two characteristic hexaoxazoles (6OTDs), one bearing two p-hydroxybenzyl groups (Y2H2–6M(4)OTD, 2a), and the other bearing two nitroveratryl-protected p-hydroxybenzyl groups (Y2Nv2-6M(4)OTD, 2b) (Figure 1). Y2H2–6M(4)OTD (2a) showed much potent G4- stabilizing activity than Y2Nv2-6M(4)OTD (2b),14 and therefore we considered that the phenolic hydroxy groups might play a key role in stabilizing G4 structures through hydrogen bonding with phosphate residues. Thus, we newly designed a 6OTD with four phenolic hydroxy group on the aromatic side chains, i.e., D2H4–6M(4)OTD (3), aiming to further improve the G4-stabilizing ability. In this paper, we described synthesis of D2H4–6M(4)OTD (3), and evaluation of its ability to stabilize several G4-forming oligonucleotides (GFOs), compared with that of 2a. We also examined its antiproliferative activity against several cancer cell lines.
RESULTS AND DISCUSSION
D2H4–6M(4)OTD (3) was synthesized based upon our previously reported procedure (Scheme 1).13g Trioxazole 4, which was synthesized from l-dopa, l-serine and l-threonine, was converted to amine 5 and carboxylic acid 6, which were coupled in the presence of N-methylmorpholine (NMM) and 4-(4,6-dimethoxy-1,3,5-triazin-2-yl)-4-methylmorpholinium chloride (DMT-MM) and to give bis- trioxazole 7 in 95% yield from 4. After deprotection of the Cbz group with hydrogen in the presence of Pd(OH)2, followed by hydrolysis of the methylester with lithium hydroxide at room temperature, the resulting amino acid was intramolecularly condensed to give macrocyclic hexaoxazole 8. Finally, the methoxymethyl (MOM) groups on the phenolic hydroxy groups were deprotected with trifluoroacetic acid (TFA) to give D2H4–6M(4)OTD (3).
We evaluated the G4-stabilizing ability of D2H4–6M(4)OTD (3) by means of fluorescence resonance energy transfer (FRET) melting assay.15 In this assay, we used the following five G4-forming oligonucleotides (GFOs): telo21 (5’-FAM-d[GGG TTA GGG TTA GGG TTA GGG]-TAMRA-3’), bcl-2 (5’-FAM-d[GGG CGC GGG AGG AAG GGG GCG GG]-TAMRA-3’), c-kit (5’-FAM-d[GGG AGG GCG CTG GGA GGA GGG]-TAMRA-3’), c-myc (5’-FAM-d[GAG GGT GGG GAG GGT GGG GAA G]-TAMRA-3’) and k-ras (5’-FAM-d[AGG GCG GTG TGG GAA GAG GGA AGA GGG GGA GG]-
TAMRA-3’). We also prepared a non-GFO (dsDNA (5’-FAM-d[TAT AGC TAT ATT TTT TTA TAG CTA TA]-TAMRA-3’)) control sequence. The Tm value of each DNA sequence was measured by FRET assay in the presence of 60 mM potassium chloride and 1.0 μM 3 or 2a, and the ΔTm values are summarized in Table 1 (entries 1–6). Compounds 3 and 2a showed high ΔTm values with every GFO (entries 1–5). Thus, these G4 ligands stabilized all G4s tested. In contrast, the ΔTm values for dsDNA in the presence of these G4 ligands were almost zero, indicating that these G4 ligands do not stabilize non-GFO (entry 6). Thus, 3 and 2a are G4-selective ligands, like other OTD derivatives. However, 2a has a higher ΔTm value than 3 for every GFO, which indicates that 3 has less potent G4-stabilizing ability than 2a.16
Next, we evaluated the antiproliferative activities of 3 and 2a against A549 (lung cancer cell line), DU145 (prostate cancer cell line), Hela (cervical cancer cell line) and MCF7 (breast cancer cell line) by means of MTT assay. These cells were incubated with various concentrations of 3 and 2a, and growth was examined after 6 days. All the cell lines were sensitive to 3, with IC50 values of ca. 900 to 4000 nM; however, 3 was less potent than 2a (Figure 2), contrary to our expectation. A possible explanation for this result would be the occurrence of intramolecular hydrogen bonding interaction between adjacent phenolic hydroxy groups in 3 (Figure 3), which would reduce the ability to interact with phosphate moieties in the DNA backbone.17 Further SAR studies are planned.18
In conclusion, we synthesized D2H4-6M(4)OTD (3), which has four phenolic groups, as a new OTD derivative and found that it showed weaker G4-stabilizing ability than 2a, which has two hydroxy groups, towards several GFOs. It also showed weaker growth-inhibitory activity than 2a towards several cancer cell lines. The weaker activities of 3 as compared with 2a may be due to intramolecular hydrogen bonding of adjacent phenolic hydroxy groups in 3, decreasing the potential for interaction with G4 phosphate groups. Further SAR studies are planned.
EXPERIMENTAL
General:
Flash chromatography was performed on Silica gel 60 (spherical, particle size 0.040–0.100 mm; Kanto). Optical rotations were measured on a JASCO P-2200 polarimeter, using the sodium lump (589 nm). 1H and 13C NMR spectra were recorded on JEOL JNM-AL 300 and JEOL JNM-ECX 400. The spectra are referenced internally according to residual solvent signals of CDCl3 (1H NMR; δ = 7.26 ppm, 13C NMR; δ = 77.0 ppm) and DMSO-d6 (1H NMR; δ = 2.50 ppm, 13C NMR; δ = 39.5 ppm). Data for 1H NMR are recorded as follows; chemical shift (δ, ppm), multiplicity (s, singlet; d, doublet; dd, double doublet; m, multiplet; br, broad), integration, coupling constant (Hz). Data for 13C NMR are recorded in terms of chemical shift (δ, ppm). Infrared (IR) spectra were recorded on a JASCO FT/IR-420 Fourier Transform Infrared Spectrophotometer and were reported in wavenumbers (cm-1). Mass spectra were recorded on JEOL JMS- T100LC spectrometer with ESI-MS mode using MeOH or MeOH-CHCl3 (1:1).
Synthesis of 5: To a solution of 4 (1.1 g, 1.70 mmol) in MeOH–THF (5:4, 90 mL) was added palladium hydroxide 20% on carbon (300 mg), and the mixture was stirred at room temperature under an atmosphere of hydrogen gas (balloon). After 20 min, the mixture was filtrated through a pad of Celite, and the filtrates were concentrated in vacuo to give amine 5, which was used without further purification.
Synthesis of 6: To a solution of 4 (1.0 g, 1.5 mmol) in THF–H2O (3:1, 60 mL) was added lithium hydroxide monohydrate (127 mg, 3.0 mmol) and the mixture was stirred at room temperature for 1 h. To the mixture was added 3 N HCl to give carboxylic acid 6 as a solution.
Synthesis of 7: To a resulting solution of carboxylic acid 6 was added the amine 5, NMM (564 μL, 5.1 mmol) and DMT–MM (1.5 mg, 5.1 mmol), and this mixture was stirred at room temperature. After 2.5 h, 1 N HCl was added to the reaction mixture, and the organic layer was extracted with EtOAc. The extracts were washed with brine, dried over MgSO4, filtrated and concentrated in vacuo. The residue was purified by silica gel column (CHCl3– EtOAc = 4:1) to give 7 (1.7 g, 95% yield, 2 steps). Spectral data for 7: [α]25D +12.1 (c 0.1, CHCl3); 1H NMR (300 MHz, CDCl3) δ 8.29 (1H, s), 8.25 (1H, s), 7.54 (1H, d, J = 6.5 Hz), 7.33 (5H, m), 7.02 (3H, m), 6.96 (1H, d, J = 1.4 Hz), 6.83 (1H, s), 6.79–6.77 (1H, dd, J = 1.5, 6.3 Hz), 6.64 (1H, d, J = 6.2 Hz), 5.64 (1H, dd, J = 5.2, 11.7 Hz), 5.44 (1H, m), 5.24–5.06 (12H, m), 3.93 (3H, s), 3.48 (3H, s), 3.47 (3H, s), 3.39 (6H, s), 3.33 (1H, m), 2.79 (3H, s), 2.77 (3H, s), 2.71 (3H, s), 2.69 (3H, s); 13C NMR (100 MHz, CDCl3) δ 161.8, 161.3, 161.2, 159.6, 156.4, 155.5, 155.3, 154.5, 154.5, 150.6, 150.4, 147.1, 147.0, 146.0, 143.2, 140.8, 136.3, 136.0, 133.9, 129.7, 129.5, 128.2, 127.8, 127.7, 125.2, 124.4, 124.2, 123.0, 117.5, 117.3, 116.5, 95.1, 66.7, 55.9, 55.7, 51.9, 50.2, 47.9, 39.0, 38.9, 11.6, 11.5; HRMS (ESI, M + Na+) calcd for C57H58N8NaO19 1181.3716, found 1181.3687.
Synthesis of 8: To a solution of 7 (1.5 g, 1.3 mmol) in THF–MeOH (7:4, 20 mL) was added palladium hydroxide 20% on carbon (300 mg), and the mixture was stirred for 3.5 h at room temperature under an atmosphere of hydrogen gas (balloon). The reaction mixture was filtrated through a pad of Celite and the filtrates were concentrated in vacuo to give amine. The amine was dissolved in THF–H2O (3:1, 80 mL), and lithium hydroxide monohydrate (109 mg, 2.6 mmol) was added at room temperature, and the mixture was stirred for 30 min. To the reaction mixture was added 1 N HCl at 0 °C, and the mixture was concentrated in vacuo to give amino acid. To a solution of amino acid in DMF–CH2Cl2 (1:2, 195 mL) was added 4-dimethylaminopyridine (DMAP, 317 mg, 2.6 mmol), iPr2EtN (884 μL, 5.2 mmol), and diphenylphosphoryl azide (DPPA, 2.8 mL, 13 mmol). The mixture was heated at 70 °C for 16 h. The reaction mixture was extracted with CHCl3, washed with NaHCO3 aq. and brine, dried over MgSO4, filtrated, and concentrated in vacuo. The residue was purified by silica gel column (CHCl3– EtOAc = 3:2) to give 8 (595 mg, 0.60 mmol, 46%, 3 steps). Spectral data for 8: [α]25D +38.5 (c 0.8, CHCl3); 1H NMR (300 MHz, CDCl3) δ 8.32 (2H, d, J = 7.2 Hz), 8.26 (2H, s), 6.96 (2H, d, J = 8.2 Hz), 6.69 (2H, d, J = 2.1 Hz), 6.55 (2H, dd, J = 2.1, 8.3 Hz), 5.47 (2H, dd, J = 6.5, 11.7 Hz), 5.18–4.99 (8H, m), 3.47 (6H, s), 3.38–3.24 (10H, m), 2.71 (6H, s), 2.63 (6H, s); 13C NMR (75 MHz, CDCl3) δ 161.2, 160.9, 159.9, 159.8, 155.7, 154.7, 150.0, 149.8, 149.7, 146.8, 146.1, 145.3, 144.3, 140.5, 136.5, 129.5, 127.2, 125.5, 124.5, 123.9, 123.4, 117.3, 116.5, 116.2, 115.1, 95.8, 95.2, 95.1, 56.1, 56.0, 55.9, 55.7, 49.4, 39.8, 39.2, 29.5, 11.6, 11.4; HRMS (ESI, M + Na+) calcd for C48H48N8NaO16 1015.3086, found 1015.3104.
Synthesis of D2H4-6M(4)OTD (3): To a solution of 8 (15 mg, 15 μmol) in CH2Cl2 (1.0 mL) was added TFA (1.0 mL) and the mixture was stirred for 2 h at room temperature. The reaction mixture was concentrated in vacuo to give D2H4–6M(4)OTD (3) as a wihte powder (12.2 mg, 15 μmol, >99%). Spectral data for 3: [α]25D +46.7 (c 0.3, DMF); IR (neat) 3186, 3016, 1669 cm-1; 1H NMR (300 MHz, DMSO-d6) δ 8.85 (2H, s), 8.70 (4H, br), 8.21 (2H, d, J = 7.5 Hz), 6.52 (2H, d, J = 7.9 Hz), 6.33 (2H, s), 6.19 (2H, d, J = 8.3 Hz), 5.46–5.40 (2H, m), 3.20–3.03 (4H, m), 2.73 (6H, s), 2.69 (6H, s); 13C NMR (75 MHz, DMSO-d6) δ 161.7, 158.9, 155.5, 154.3, 150.9, 150.8, 145.0, 144.1, 142.1, 136.0, 126.4, 124.7, 123.7, 120.1, 116.7, 115.6, 48.8, 11.4; HRMS (ESI, M + Na+) calcd for C40H32N8NaO12 839.2037, found 839.2038.
Cell lines and cell culture
A549, DU145, Hela and MCF7 cells were cultured in Dulbecco’s modified Eagle Medium (Nacalai Tesque) supplemented with 10% (v/v) fetal bovine serum (Cellgro) and 0.1 mg/mL kanamycin sulfate (Meiji-Seika Pharma).
FRET melting assay
FRET melting assay were carried out as reported methods. The dual fluorescently labeled oligonucleotides of telo21 (5’-FAM-d[GGG TTA GGG TTA GGG TTA GGG]-TAMRA-3’), bcl-2 (5’-FAM-d[GGG CGC GGG AGG AAG GGG GCG GG]-TAMRA-3’), c-kit (5’-FAM-d[GGG AGG GCG CTG GGA GGA GGG]-TAMRA-3’), c-myc (5’-FAM-d[GAG GGT GGG GAG GGT GGG GAA G]-TAMRA-3’), k-ras (5’-FAM-d[AGG GCG GTG TGG GAA GAG GGA AGA GGG GGA GG]-TAMRA-3’) and dsDNA (5’-FAM-d[TAT AGC TAT ATT TTT TTA TAG CTA TA]-TAMRA-3’) were purchased from Sigma® Genosys. All nucleotides were dissolved as a 10 μM stock solution in MilliQ water to be used without further purification. Further dilutions of the oligonucleotides were performed with FRET buffer (60 mM potassium cacodylate buffer (pH 7.4)), and dual-labeled DNAs at 400 nM were annealed by heating at 96 °C for 5 min, and cooled to room temperature. Ligand 2a and 3 (1.0 mM in DMSO) were diluted to 200 μM using DMSO. These ligands were further diluted to 2.0 μM with FRET buffer. Annealed DNA (20 μL) and compound solution (20 μL) were distributed across 96-well plates (Roche), with 200 nM of labeled oligonucleotide and 1.0 μM of the compounds (a total volume of 40 μL) and these mixtures were incubated at 4 °C for 16 h. Measurements were carried out in triplicate with an excitation wavelength of 470 nm and a detection wavelength of 514 nm using a LightCycler® 96 Real-Time PCR System (Roche). The change in melting temperature at a 1.0 μM ligand concentration (ΔTm (1.0 μM)) was calculated from three experiments by subtraction of the blank from the averaged 1.0 μM ligand melting temperature.
CD spectra
CD spectrum was recorded with a J–720 spectropolarimeter (JASCO) in a quartz cell of 1.0 mm optical path length. Telo24 (5’-d[TTA GGG TTA GGG TTA GGG TTA GGG]-3’, 1.0 mM) was diluted with Tris–HCl buffer (pH 7.2, 50 mM) containing 100 mM KCl to 10 μM (200 μL). This oligonucleotide was denatured at 95 °C for 5 min and cooled room temperature. Subsequently, 1.0 μL of ligand (10 mM in DMSO) was added to this solution and incubated for 16 h at 4 °C. The CD spectrum was obtained with an instrument scanning speed of 100 nm/min and a response time of 1 s, over the wavelength range of 230–320 nm. The spectrum is representative of ten averaged scans taken at 25 °C.
Cellular proliferation assay
Eighty micro litter of 125 cells/well (Hela) or 250 cells/well (A549, DU145 and MCF7) were seeded into the wells of 96-welll microplates and incubated for 16 h. These cells were treated with 20 μL of 2a or 3 at increasing concentrations and were further incubated for 6 days. Subsequently, these cells were added 10 μL of MTT (3-(4,5-dimethylthiazol-2-yl)-2,5-diphenyltetrazolium bromide, Sigma-Aldrich) in PBS(–) (5.0 mg/mL) and incubated for 4 h at 37 °C. After removing the solvent, these cells were added 100 μL of DMSO (Nacalai tesque). Colorimetric reading at 570 nm and 630 nm (as a control) was performed with a microplate reader (xMark Microplate Reader, Bio-Rad). The results are expressed as the mean percentages of cellular growth inhibition from triplicate cultures.
ACKNOWLEDGEMENTS
This work was supported in part by Grants-in-Aid for Scientific Research (B) from JSPS (26282214). T.N. is grateful for financial support in the form of JSPS Predoctoral Fellowships for Young Scientists (13J07907).
References
1. (a) A. P. Bird, Nature, 1986, 321, 209; CrossRef (b) F. Antequera and A. Bird, Proc. Natl. Acad. Sci. USA, 1993, 90, 11995; CrossRef (c) E. Besnard, A. Babled, L. Lapasset, O. Milhavet, H. Parrinello, C. Dantec, J. M. Marin, and J. M. Lemaitre, Nat. Struct. Mol. Biol., 2012, 19, 837; CrossRef (d) C. Cayrou, D. Gregoire, P. Coulombe, E. Danis, and M. Mechali, Methods, 2012, 57, 158; CrossRef (e) J. C. Cadoret, F. Meisch, V. Hassan-Zadeh, I. Luyten, C. Guillet, L. Duret, H. Quesneville, and M. N. Prioleau, Proc. Natl. Acad. Sci. USA, 2008, 105, 15837; CrossRef (f) Z. Du, Y. Zhao, and N. Li, Nucleic Acids Res., 2009, 37, 6784; CrossRef (g) V. S. Chambers, G. Marsico, J. M. Boutell, M. Di Antonio, G. P. Smith, and S. Balasubramanian, Nat. Biotechnol., 2015, 33, 877.
2. K. Abdelmohsen, K. Tominaga, E. K. Lee, S. Srikantan, M. J. Kang, M. M. Kim, R. Selimyan, J. L. Martindale, X. Yang, F. Carrier, M. Zhan, K. G. Becker, and M. Gorospe, Nucleic Acids Res., 2011, 39, 8513. CrossRef
3. C. M. Azzalin, P. Reichenbach, L. Khoriauli, E. Giulotto, and J. Lingner, Science, 2007, 318, 798. CrossRef
4. J. T. Davis, Angew. Chem. Int. Ed., 2004, 43, 668. CrossRef
5. A. L. Valton, V. Hassan-Zadeh, I. Lema, N. Boggetto, P. Alberti, C. Saintomé, J. F. Riou, and M. N. Prioleau, EMBO J., 2014, 33, 732. CrossRef
6. S. Balasubramanian, L. H. Hurley, and S. Neidle, Nat. Rev. Drug Discov., 2011, 10, 261. CrossRef
7. A. L. Wolfe, K. Singh, Y. Zhong, P. Drewe, V. K. Rajasekhar, V. R. Sanghvi, K. J. Mavrakis, M. Jiang, J. E. Roderick, J. Van der Meulen, J. H. Schatz, C. M. Rodrigo, C. Zhao, P. Rondou, E. de Stanchina, J. Teruya-Feldstein, M. A. Kelliher, F. Speleman, J. A. Porco Jr., J. Pelletier, G. Rätsch, and H. G. Wendel, Nature, 2014, 513, 65. CrossRef
8. N. Maizels, EMBO Rep., 2015, 16, 910. CrossRef
9. S. Zhang, Y. Wu, and W. Zhang, ChemMedChem, 2014, 9, 899. CrossRef
10. (a) A. M. Burger, F. Dai, C. M. Schultes, A. P. Reszka, M. J. Moore, J. A. Double, and S. Neidle, Cancer Res., 2005, 65, 1489; CrossRef (b) M. Gunaratnam, C. Green, J. B. Moreira, A. D. Moorhouse, L. R. Kelland, J. E. Moses, and S. Neidle, Biochem. Pharmacol., 2009, 78, 115; CrossRef (c) C. L. Grand, H. Han, R. M. Muñoz, S. Weitman, D. D. Von Hoff, L. H. Hurley, and D. J. Bearss, Mol. Cancer Ther. 2002, 1, 565; (d) D. Drygin, A. Siddiqui-Jain, S. O'Brien, M. Schwaebe, A. Lin, J. Bliesath, C. B. Ho, C. Proffitt, K. Trent, J. P. Whitten, J. K. Lim, D. Von Hoff, K. Anderes, and W. G. Rice, Cancer Res., 2009, 69, 7653; CrossRef (e) M. Gunaratnam, M. de la Fuente, S. M. Hampel, A. K. Todd, A. P. Reszka, A. Schätzlein, and S. Neidle, Bioorg. Med. Chem., 2011, 19, 7151; CrossRef (f) M. Jeitany, J. R. Pineda, Q. Liu, R. M. Porreca, F. Hoffschir, C. Desmaze, D. C. Silvestre, P. Mailliet, M. P. Junier, A. Londoño-Vallejo, E. Ségal-Bendirdjian, H. Chneiweiss, and F. D. Boussin, Int. J. Cancer, 2015, 136, 1546; (g) Z. F. Chen, Q. P. Qin, J. L. Qin, Y. C, Liu, K. B. Huang, Y. L. Li, T. Meng, G. H. Zhang, Y. Peng, X. J. Luo, and H. Liang, J. Med. Chem., 2015, 58, 2159. CrossRef
11. K. Shin-ya, K. Wierzba, K. Matsuo, T. Ohtani, Y. Yamada, K. Furihata, Y. Hayakawa, and H. Seto, J. Am. Chem. Soc., 2001, 123, 1262. CrossRef
12. T. Miyazaki, Y. Pan, K. Joshi, D. Purohit, B. Hu, H. Demir, S. Mazumder, S. Okabe, T. Yamori, M. Viapiano, K. Shin-ya, H. Seimiya, and I. Nakano, Clin. Cancer Res., 2012, 18, 1268. CrossRef
13. (a) M. Tera, Y. Sohtome, H. Ishizuka, T. Doi, M. Takagi, K. Shin-ya, and K. Nagasawa, Heterocycles, 2006, 69, 505; CrossRef (b) M. Tera, H. Ishizuka, M. Takagi, M. Suganuma, K. Shin-ya, and K. Nagasawa, Angew. Chem. Int. Ed., 2008, 47, 5557; CrossRef (c) M. Tera, K. Iida, H. Ishizuka, M. Takagi, M. Suganuma, T. Doi, K. Shin-ya, and K. Nagasawa, ChemBioChem, 2009, 10, 431; CrossRef (d) M. Tera, K. Iida, K. Ikebukuro, H. Seimiya, K. Shin-ya, and K. Nagasawa, Org. Biomol. Chem., 2010, 8, 2749; CrossRef (e) K. Iida, M. Tera, T. Hirokawa, K. Shin-ya, and K. Nagasawa, Chem. Commun., 2009, 6481; CrossRef (f) K. Iida, M. Tera, T. Hirokawa, K. Shin-ya, and K. Nagasawa, J. Nucleic Acids, 2010, Article ID: 217627; (g) S. Majima, M. Tera, K. Iida, K. Shin-ya, and K. Nagasawa, Heterocycles, 2011, 82, 1345; (h) K. Iida, S. Majima, T. Ohtake, M. Tera, K. Shin-ya, and K. Nagasawa, Heterocycles, 2011, 84, 401; (i) K. Iida, S. Majima, T. Nakamura, H. Seimiya, and Nagasawa, K. Molecules, 2013, 18, 4328; CrossRef (j) K. Iida, G. Tsubouchi, T. Nakamura, S. Majima, H. Seimiya, and K. Nagasawa, Med. Chem. Commun., 2013, 4, 260; CrossRef (k) K. Iida, S. Majima, T. Nakamura, H. Seimiya, and K. Nagasawa, Molecules, 2013, 18, 4328; CrossRef (l) K. Iida, T. Nakamura, W. Yoshida, M. Tera, K. Nakabayashi, K. Hata, K. Ikebukuro, and K. Nagasawa, Angew. Chem. Int. Ed., 2013, 52, 12052; CrossRef (m) K. Iida, G. Tsubouchi, T. Nakamura, and K. Nagasawa, Heterocycles, 2014, 88, 1287; CrossRef (n) S. S. Masoud, Y. Tsushima, K. Iida, and K. Nagasawa, Heterocycles, 2014, 90, 866.
14. T. Nakamura, K. Iida, M. Tera, K. Shin-ya, H. Seimiya, and K. Nagasawa, ChemBioChem, 2012, 13, 774. CrossRef
15. (a) J.-L. Mergny and J.-C. Maurizot, ChemBioChem, 2001, 2, 124; CrossRef (b) A. De Cian, L. Guittat, M. Kaiser, B. Sacca, S. Amrane, A. Bourdoncle, P. Alberti, M.-P. Teulade-Fichou, L. Lacroix, and J.-L. Mergny, Methods, 2007, 42, 183.
16. The hybrid structure of telo24 (d[TTA GGG TTA GGG TTA GGG TTA GGG]) was not transformed by 3 or 2a (50 μM) in the presence of potassium chloride (Figure S1). These results suggest that compounds 3 and 2a may stabilize the hybrid-type G4 structure of telo24.
17. The spectrum analysis of 3 on infrared spectroscopy revealed that phenolic hydroxy groups on 3 form intramolecular hydrogen bonds. IR data for 2a; (neat) 3238 (s, br), 3035, 1669 (s), 1642 (s) cm-1; IR data for 3; (neat) 3186 (br), 3016, 1669 (s) cm-1.
18. 1. Another possible explanations for showing the weaker activity of 3 would be the steric hindrance between G4 and the phenolic hydroxy groups on a meta-position in 3. To figure out the effects of additional hydroxy groups in 3, further SAR studies are planned. Moreover, docking studies of telomeric G4 and 3 are planned to obtain more detail information of their interaction manner.