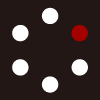
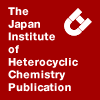
HETEROCYCLES
An International Journal for Reviews and Communications in Heterocyclic ChemistryWeb Edition ISSN: 1881-0942
Published online by The Japan Institute of Heterocyclic Chemistry
e-Journal
Full Text HTML
Received, 7th December, 2015, Accepted, 18th January, 2016, Published online, 2nd February, 2016.
DOI: 10.3987/COM-15-13387
■ An Efficient and Convenient Synthesis of Acyl CoA: Monoacylglycerol Acyltransferase 2 Inhibitor, 2-[2-(4-tert-Butylphenyl)ethyl]-N-[4-(3-cyclopentylpropyl)-2-fluorophenyl]-1,2,3,4-tetrahydroisoquinoline-6-sulfonamide
Tsuyoshi Busujima* and Hiroaki Tanaka
Medicinal Chemistry 3, Taisho Pharmaceutical Co., Ltd., 1-403 Yoshino-cho Kita-ku Saitama 331-9530, Japan
Abstract
An efficient and convenient synthesis of MGAT2 inhibitor, 2-[2-(4-tert-butylphenyl)ethyl]-N-[4-(3-cyclopentylpropyl)-2-fluorophenyl]-1,2,3,4-tetrahydroisoquinoline-6-sulfonamide (1), is reported. The optimized route consists of an effective chlorosulfonylation and debromination, resulting in an increase in the total yield from 6.8% to 45%, compared with our previous method. This synthetic approach enabled the synthesis of 1 to be scaled-up to a multi-gram scale.introduction
The 1,2,3,4-tetrahydroisoquinoline scaffold is one of the most important heterocycles in natural and pharmaceutical products. So far, many kinds of tetrahydroisoquinoline derivatives have been reported to show pharmacological activities, including antitumor,1 antibacterial,2 antihypertensive,3 CNS depressant activities,4 and antimalarial actions.5
Through our investigation to identify a novel acyl CoA:monoacylglycerol acyltransferase 2 (MGAT2) inhibitor, 2-[2-(4-tert-butylphenyl)ethyl]-N-[4-(3-cyclopentylpropyl)-2-fluorophenyl]-1,2,3,4-tetrahydro- isoquinoline-6-sulfonamide (1) was identified as a potential anti-obesity agent that demonstrated a significant suppression of fat absorption in an oral lipid tolerance test performed in a mouse model at an oral dose of 3 mg/kg (Figure 1).6 MGAT2 catalyzes the synthesis of diacylglycerol from monoacylglycerol and acyl-CoA, playing a crucial role in triglyceride (TG) re-synthesis in the small intestine.7 Based on its mechanism and the phenotype of MGAT2 deficient mice,8 the inhibition of MGAT2 is thought to be an attractive mechanism for reducing fat absorption and treating obesity and obesity-associated metabolic disorders. Indeed, several kinds of MGAT2 inhibitors have already been reported.9-10 Recently, we also reported a potent and orally available MGAT2 inhibitor.6 With this background in mind, we expect compound 1 to be of value in further pharmacology and toxicological studies.
Our previously reported primary synthetic route11 for compound 1 has a critical issue that hinders multi-gram synthesis. Specifically, the total yield of that procedure was only 6.8% because of the low regioselectivity of the chlorosulfonylation step (from compound 3 to 4), as shown in Scheme 1. In addition, the low selectivity meant that a laborious separation of compounds 4 and 5 using column chromatography was necessary.
Herein, we describe improved synthetic routes that enabled the preparation of compound 1 in multi-gram quantities.
RESULTS AND DISCUSSION
First, to consider the regioselectivity of chlorosulfonylation for compound 3, the electric density of the benzene ring was calculated using a Natural Population Analysis12 (Figure 2). As a result, no significant difference in the electric densities was found between the 6-position and the 7-position of compound 3.
Indeed, the desired chlorosulfonylated compound 4 was obtained in only 14% after a laborious silica-gel chromatography procedure to separate it from its regioisomer 5. The structures of 4 and 5 were determined by NOE experiments, respectively. NOEs were observed between the aromatic hydrogens of 4 (Ha) or 5 (Hb) and the hydrogens of the methylene chain at 1-position (Figure 3).
Because of the low selectivity of the chlorosulfonylation of 3, the total yield of compound 1 was very low (6.8% in 6 steps). These results suggested that it was difficult to control the regioselectivity of the chlorosulfonylation by simply tuning the reaction conditions.
To obtain the desired intermediate 4 more effectively, 7-bromo-1,2,3,4-tetrahydroisoquinoline 8, which was commercially available, was selected as a starting material instead of tetrahydroisoquioline 2. As a model case, we attempted to optimize the synthetic route for the preparation of the 4-fluorophenyl- sulfonamide derivative 12 as shown in Scheme 2.
After compound 8 was converted to its trifluoroacetamide derivative 9, chlorosulfonylation was performed at 60 °C to afford 10 at a 72% yield. Compound 10 was condensed with 4-fluoroaniline to yield 11 in a high yield (93%). The hydrogenolysis of 11 to remove the bromo group proceeded smoothly to produce 12 (98% yield). These results indicated that the alteration of the starting material worked well, and we next considered applying this methodology to the preparation of compound 1.
In the above-described synthetic route, however, the debromination by hydrogenolysis required a 50 wt% amount of palladium on a carbon catalyst, which could be a potential issue in terms of safety for a multi-gram scale of synthesis and/or the presence of residual metals in the final product. Therefore, a means of reducing the amount of palladium catalyst was examined. The results are shown in Table 1.
Although the hydrogenolysis proceeded smoothly to afford 12 in a high yield (98% isolated yield, Entry 1) using a 50 wt% amount of palladium catalyst, the reduction of the amount of palladium catalyst to 10 wt% resulted in a reduced yield (63%, Entry 2) because the reaction was not completed. On the other hand, the use of triethylamine promoted the debromination of 11 to provide 12 in a quantitative yield (Entry 3).13
This reaction condition was applicable for the debromination of other tetrahydroisoquinoline derivatives (Table 2). As shown in entry 2, the debromination of the compound with the substituent of compound 1 afforded the desired product 6 at an 89% yield.
Having optimized the reaction conditions, we attempted to synthesize compound 1 on a multi-gram scale, as summarized in Scheme 3. Starting with 100 g of 7-bromo-1,2,3,4-tetrahydroisoqinoline 8, the protection of the secondary amine using trifluoroacetic anhydride converted 8 to 9. The chlorosulfonylation of 9 was performed to afford 110 g of intermediate 10, which was isolated by precipitation with ethyl acetate and diisopropyl ether. The condensation reaction of 10 with 13 prepared from allyl cyclopropane proceeded smoothly to provide 14 without purification. The following debromination reaction of 14 using the same reaction condition as that mentioned above afforded 89.4 g of intermediate 6 in a high yield (97% in 2 steps). This intermediate was purified using silica gel column chromatography. The deprotection of the trifluoroacetyl group of 6 under a basic condition afforded 7 in a quantitative yield, followed by N-alkylation through reductive amination with 2-(4-(tert-butyl)phenyl)acetaldehyde to provide 84.5 g of 1.
The overall yield of compound 1 from the starting material 8 was 45% in 7 steps, which was 7-fold larger than the yield of the initial synthetic route (6.8% in 6 steps).
In summary, the multi-gram scale synthesis of compound 1, which is a potent MGAT2 inhibitor, was achieved by changing the starting material and optimizing of the reaction conditions for the debromination step. This new synthetic route enabled the production of compound 1 sufficient to enable toxicological profiling as well as further in vivo pharmacology studies.
EXPERIMENTAL
All commercially available starting materials and reagents were used without further purification unless otherwise noted. Thin layer chromatography was performed to monitor the reactions using Merck silica gel 60 F254 plates or Fuji Silysia chromatorex NH plates. Silica gel column chromatography was performed using Wakogel® C‒200, or NH‒silica gel Fuji Silysia chromatorex® DM1020, or an appropriately sized pre-packed silica cartridge on a Biotage system. Melting points were determined using a Yanako micro melting point apparatus and were not corrected. The 1H NMR spectra were determined using TMS as an internal reference with a JEOL ECA‒600 NMR spectrometer operating at 600 MHz. The 13C NMR spectra were determined using TMS as an internal reference with a JEOL ECA‒600 NMR spectrometer operating at 151 MHz. The mass spectra (MS) were recorded using a Shimadzu LCMS-2010EV mass spectrometer with an electrospray ionization (ESI)/atmospheric pressure chemical ionization (APCI) dual source. High resolution mass spectra (HRMS) were recorded using a Shimadzu LCMS-IT-TOF mass spectrometer with an ESI/APCI dual source or a Micromass GCT mass spectrometer with an electron ionization (EI). Elemental analyses were performed using a Perkin–Elmer 2400II, and the results were within ±0.4% of the calculated values.
1-(3,4-Dihydroisoquinolin-2(1H)-yl)-2,2,2-trifluoroethanone (3). Pyridine (6.47 mL, 80.0 mmol) and 4-N,N-dimethylaminopyridine (244 mg, 2.00 mmol) were added to a solution of 1,2,3,4-tetrahydroisoquinoline (5.00 mL, 40 mmol) in CHCl3 (100 mL) at room temperature. The mixture was cooled to 0 °C, and trifluoroacetic anhydride (6.67 mL, 48.0 mmol) was added in a dropwise manner to the mixture. After the addition, the reaction mixture was warmed to room temperature and stirred for 6 h. The reaction mixture was concentrated under reduced pressure, and the residue was diluted with EtOAc. The mixture was then washed with 1 mol/L hydrochloric acid, saturated aqueous NaHCO3, and brine. The organic layer was dried over anhydrous MgSO4, filtered, and then concentrated under reduced pressure. The resulting residue was purified using a silica gel column eluted with 10% EtOAc/n-hexane to afford 3 (8.16 g, 89%); a colorless oil; 1H NMR (600 MHz, CDCl3) δ 2.92‒2.98 (m, 2H), 3.81‒3.91 (m, 2H), 4.71‒4.82 (m, 2H), 7.08‒7.30 (m, 4H); 13C NMR (151 MHz, CDCl3) δ 27.8, 29.1, 41.8, 43.3, 45.5, 46.9, 113.6, 115.5, 117.4, 119.4, 126.0, 126.5, 126.8, 126.9, 127.1, 127.5, 128.6, 128.8, 131.4, 133.2, 134.0, 155.9, 156.0. HRMS (EI) m/z Calcd for C11H10F3NO: M+, 229.0714. Found: 229.0701.
2-(Trifluoroacetyl)-1,2,3,4-tetrahydroisoquinoline-6-sulfonyl chloride (4) and 2-(trifluoroacetyl)-1,2,3,4-tetrahydroisoquinoline-7-sulfonyl chloride (5). A solution of 3 (200 mg, 0.873 mmol) in CHCl3 (3 mL) was cooled at 0 °C, and chlorosulfonic acid (379 µL, 5.70 mmol) was added in a dropwise manner. The reaction mixture was warmed to room temperature and stirred overnight. The reaction mixture was poured into ice water. The aqueous layer was extracted twice with CHCl3, and the combined organic layers were washed with water, dried over anhydrous MgSO4, filtered, and concentrated under reduced pressure. The resulting residue was purified using a silica gel column eluted with 20% EtOAc/n-hexane to afford 4 (39.3 mg, 14%) and 5 (169 mg, 59%).
2-(Trifluoroacetyl)-1,2,3,4-tetrahydroisoquinoline-6-sulfonyl chloride (4); a colorless amorphous; 1H NMR (600 MHz, CDCl3) δ 3.06‒3.14 (m, 2H), 3.90‒4.00 (m, 2H), 4.85‒4.94 (m, 2H), 7.37‒7.46 (m, 1H), 7.85‒7.94 (m, 2H); 13C NMR (151 MHz, CDCl3) δ 27.9, 29.2, 41.0, 42.7, 45.4, 46.9, 115.3, 117.2, 125.3, 127.3, 127.6, 128.1, 135.4, 136.3, 139.2, 139.6, 143.1, 143.5. HRMS (ESI/APCI dual) m/z Calcd for C11H9ClF3NO3S: (M−H)−, 325.9871. Found: 325.9899.
2-(Trifluoroacetyl)-1,2,3,4-tetrahydroisoquinoline-7-sulfonyl chloride (5); a colorless amorphous; 1H NMR (600 MHz, CDCl3) δ 3.04‒3.13 (m, 2H), 3.87‒4.01 (m, 2H), 4.83‒4.94 (m, 2H), 7.40‒7.49 (m, 1H), 7.80‒7.95 (m, 2H); 13C NMR (151 MHz, CDCl3) δ 28.2, 29.6, 40.9, 42.6, 45.2, 46.7, 115.3, 117.2, 124.8, 125.4, 125.4, 125.9, 130.2, 130.5, 133.4, 133.7, 141.5, 142.4, 142.9, 143.0. HRMS (ESI/APCI dual) m/z Calcd for C11H9ClF3NO3S: (M+Cl)−, 361.9638. Found: 361.9667.
N-[4-(3-Cyclopentylpropyl)-2-fluorophenyl]-2-(trifluoroacetyl)-1,2,3,4-tetrahydroisoquinoline-6-sulfonamide (6). Pyridine (49.5 µL, 0.615 mmol) and 4-N,N-dimethylaminopyridine (3.75 mg, 0.0307 mmol) were added to a solution of 4-(3-cyclopentylpropyl)-2-fluoroaniline hydrochloride 13 (83.2 mg, 0.323 mmol) in CHCl3 (3 mL) at room temperature. The mixture was cooled to 0 °C, and 4 (101 mg, 0.307 mmol) was added to the mixture. The reaction mixture was warmed to room temperature and stirred for 19 h. The reaction mixture was concentrated under reduced pressure, and the residue was diluted with EtOAc. The mixture was washed with 1 mol/L hydrochloric acid, and then with brine. The organic layer was dried over anhydrous MgSO4, filtered, and concentrated under reduced pressure. The resulting residue was purified using a silica gel column eluted with 20% EtOAc/n-hexane to afford 6 (150 mg, 95%); a colorless amorphous; 1H NMR (600 MHz, CDCl3) δ 0.98‒1.09 (m, 2H), 1.25‒1.32 (m, 2H), 1.45‒1.61 (m, 6H), 1.69‒1.77 (m, 3H), 2.52 (t, J = 7.8 Hz, 2H), 2.91‒2.98 (m, 2H), 3.81‒3.91 (m, 2H), 4.74‒4.84 (m, 2H), 6.63‒6.69 (m, 1H), 6.76‒6.82 (m, 1H), 6.89‒6.95 (m, 1H), 7.16‒7.23 (m, 1H), 7.42‒7.49 (m, 1H), 7.55‒7.64 (m, 2H); 13C NMR (151 MHz, CDCl3) δ 14.2, 21.0, 25.1, 27.7, 29.1, 30.2, 32.6, 35.5, 35.6, 39.9, 41.2, 42.9, 45.4, 46.7, 115.2, 115.3, 121.3, 121.4, 124.1, 124.2, 124.7, 125.5, 125.5, 126.8, 127.3, 127.5, 127.7, 134.4, 135.2, 136.6, 137.0, 137.9, 138.4, 142.7, 153.5, 153.6, 155.2, 155.9. HRMS (ESI/APCI dual) m/z Calcd for C25H28F4N2O3S: (M+H)+, 513.1830. Found: 513.1821.
N-[4-(3-Cyclopentylpropyl)-2-fluorophenyl]-1,2,3,4-tetrahydroisoquinoline-6-sulfonamide (7). An aqueous solution (2 mL) of potassium hydroxide (88.0 mg, 1.56 mmol) was added to a suspension of 6 (401 mg, 0.782 mmol) in EtOH (8 mL), and the mixture was stirred at room temperature for 4 h. The mixture was concentrated under reduced pressure, and the resulting residue was diluted with water. After cooling to 0 °C, the resulting mixture was acidified by the addition of 1 mol/L hydrochloric acid, and saturated aqueous NaHCO3 was added to adjust the pH to 7 to 8. The resulting precipitates were collected by filtration to afford 7 (313 mg, 96%); a colorless powder; mp 176‒178 °C; 1H NMR (600 MHz, DMSO-d6) δ 0.96‒1.05 (m, 2H), 1.20‒1.27 (m, 2H), 1.41‒1.58 (m, 6H), 1.65‒1.76 (m, 3H), 2.44‒2.51 (m, 2H), 2.69 (t, J = 5.9 Hz, 2H), 2.94 (t, J = 5.9 Hz, 2H), 3.88 (s, 2H), 6.87‒6.91 (m, 1H), 6.93‒6.98 (m, 1H), 7.09 (t, J = 8.3 Hz, 1H), 7.14‒7.18 (m, 1H), 7.37‒7.44 (m, 2H); 13C NMR (151 MHz, DMSO-d6) δ 24.7, 28.3, 29.7, 32.2, 34.5, 35.0, 42.6, 47.4, 115.4, 115.5, 123.5, 124.2, 126.0, 126.8, 127.0, 135.8, 138.1, 141.0, 141.4, 154.7, 156.3; MS (ESI/APCI dual) m/z 417 (M+H)+. HRMS (ESI/APCI dual) m/z Calcd for C23H29FN2O2S: (M+H)+, 417.2007. Found: 417.1993.
2-[2-(4-tert-Butylphenyl)ethyl]-N-[4-(3-cyclopentylpropyl)-2-fluorophenyl]-1,2,3,4-tetrahydroisoquinoline-6-sulfonamide (1). To a solution of 7 (100 mg, 0.240 mmol) in THF (8 mL) were added a solution of 2-(4-(tert-butyl)phenyl)acetaldehyde (47.0 mg, 0.264 mmol) in THF (2 mL), and the mixture was stirred at room temperature for 5 min. To the mixture was added sodium triacetoxyborohydride (76.0 mg, 0.360 mmol), and the mixture was stirred at room temperature for 15 h. Saturated aqueous NaHCO3 and water were added to the reaction mixture, and the mixture was extracted 3 times with CHCl3. The organic layer was washed with brine, dried over anhydrous MgSO4, filtered, and concentrated under reduced pressure. The resulting residue was purified using a silica gel column eluted with 30% EtOAc/n-hexane to afford 1 (96 mg, 69%) as a colorless gum.
To a solution of 1 in EtOAc (5 mL) was added 4 mol/L hydrogen chloride in EtOAc (1 mL), and the mixture was stirred at room temperature for 15 h. After the volatiles were removed by rotary evaporation, 20% EtOAc/n-hexane was added to the residue. The resulting precipitates were collected by filtration to afford the monohydrochloride salt of 1 (89 mg, 87%); a colorless powder; mp 195‒197 °C; 1H NMR (600 MHz, DMSO-d6) δ 0.94‒1.06 (m, 2H), 1.20‒1.29 (m, 2H), 1.27 (s, 9H), 1.41‒1.59 (m, 6H), 1.66‒1.76 (m, 3H), 2.49‒2.54 (m, 2H), 3.06‒3.19 (m, 3H), 3.28‒3.45 (m, 4H), 3.73‒3.81 (m, 1H), 4.39‒4.48 (m, 1H), 4.66‒4.75 (m, 1H), 6.92‒6.97 (m, 1H), 6.98‒7.03 (m, 1H), 7.08‒7.13 (m, 1H), 7.22 (d, J = 8.3 Hz, 2H), 7.34‒7.42 (m, 3H), 7.57‒7.65 (m, 2H), 10.12 (s, 1H), 11.64 (br s, 1H); 13C NMR (151 MHz, DMSO-d6) δ 24.7, 24.8, 28.9, 29.7, 31.1, 32.2, 34.1, 34.6, 35.1, 48.2, 51.2, 56.1, 115.6, 115.7, 121.4, 121.5, 124.4, 124.5, 125.4, 126.6, 126.8, 127.5, 128.4, 132.8, 133.6, 133.9, 139.5, 142.7, 142.7, 149.2, 154.9, 156.6; MS (ESI/APCI dual) m/z 577 (M+H)+, 575 (M-H)-. Anal. Calcd for C35H45FN2O2S HCl: C, 68.55; H, 7.56; N, 4.57. Found: C, 68.46; H, 7.57; N, 4.55. HRMS (ESI/APCI dual) m/z Calcd for C35H45FN2O2S: (M+H)+, 577.3259. Found: 577.3248.
1-(7-Bromo-3,4-dihydroisoquinolin-2(1H)-yl)-2,2,2-trifluoroethanone (9). Pyridine (3.79 mL, 47.1 mmol) and 4-N,N-dimethylaminopyridine (58.0 mg, 0.471 mmol) were added to a solution of 7-bromo-1,2,3,4-tetrahydroisoquinoline (5.00 g, 23.6 mmol) in CHCl3 (80 mL). After cooling to 0 °C, trifluoroacetic acid anhydride (3.59 mL, 25.9 mmol) was added in a dropwise manner to the mixture. The mixture was warmed to room temperature and stirred for 14 h. The mixture was concentrated under reduced pressure, and the resulting residue was diluted with EtOAc. The mixture was washed with 1 mol/L hydrochloric acid, saturated aqueous NaHCO3 solution and then brine, dried over anhydrous MgSO4, filtered, and concentrated under reduced pressure. The resulting residue was purified using a silica gel column eluted with 10% to 25% EtOAc/n-hexane to afford 9 (6.97 g, 96%); a pale yellow oil; 1H NMR (600 MHz, CDCl3) δ 2.86‒2.96 (m, 2H), 3.80‒3.90 (m, 2H), 4.69‒4.79 (m, 2H), 7.01‒7.08 (m, 1H), 7.24‒7.38 (m, 2H); 13C NMR (151 MHz, CDCl3) δ 27.3, 28.8, 41.6, 43.1, 45.0, 46.5, 115.4, 117.3, 120.3, 120.4, 128.9, 129.4, 129.9, 130.2, 130.5, 130.6, 132.2, 133.5, 155.8, 156.1. HRMS (EI) m/z Calcd for C11H9BrF3NO: M+, 306.9820. Found: 306.9792.
7-Bromo-2-(trifluoroacetyl)-1,2,3,4-tetrahydroisoquinoline-6-sulfonyl chloride (10). Chlorosulfonic acid (20.0 mL, 301 mmol) was added in a dropwise manner to a solution of 9 (4.95 g, 16.1 mmol) in CHCl3 (15 mL) at 0 °C, and the mixture was stirred at room temperature for 1 h and at 60 °C for 2 h. After cooling to room temperature, the reaction mixture was added in a dropwise manner to ice water, and the mixture was extracted four times with CHCl3. The organic layer was dried over anhydrous MgSO4, filtered, and concentrated under reduced pressure. The resulting residue was purified using a silica gel column eluted with 10% to 34% EtOAc/n-hexane, and the fractions incruding product were collected and evaporated by rotary evaporation. To the residue was added Et2O, and the resulting precipitates were collected by filtration to afford 10 (3.14 g, 48%) as a colorless powder. The filtrate was concentrated under reduced pressure, and the resulting residue was purified using a silica gel column eluted with 10% to 34% EtOAc/n-hexane, and the fractions incruding product were collected and evaporated by rotary evaporation. To the residue was added Et2O, and the resulting precipitates were collected by filtration to afford 10 (1.56 g, 24%) (Total 4.70 g, 72%); a colorless powder; mp 103‒105 °C; 1H NMR (600 MHz, CDCl3) δ 2.97‒3.08 (m, 2H), 3.87‒4.00 (m, 2H), 4.79‒4.91 (m, 2H), 7.62‒7.69 (m, 1H), 7.99‒8.05 (m, 1H); 13C NMR (151 MHz, CDCl3) δ 27.4, 28.8, 40.9, 42.7, 44.8, 46.3, 115.2, 117.2, 118.3, 131.0, 131.3, 133.8, 133.9, 134.3, 134.7, 140.0, 140.5, 141.6. HRMS (ESI/APCI dual) m/z Calcd for C11H8BrClF3NO3S: (M−H)−, 403.8976. Found: 403.8994.
7-Bromo-N-(4-fluorophenyl)-2-(trifluoroacetyl)-1,2,3,4-tetrahydroisoquinoline-6-sulfonamide (11). To a solution of 10 (2.67 g, 6.57 mmol) in CHCl3 (20 mL) was added 4-fluoroaniline (0.690 mL, 7.22 mmol), pyridine (1.06 mL, 13.1 mmol) and 4-N,N-dimethylaminopyridine (80.0 mg, 0.657 mmol) at room temperature. The mixture was stirred for 3 h. The mixture was concentrated under reduced pressure, and the resulting residue was diluted with EtOAc. The mixture was washed with 1 mol/L hydrochloric acid, saturated aqueous NaHCO3 and then brine, dried over anhydrous MgSO4, filtered, and concentrated under reduced pressure. The resulting residue was purified using a silica gel column eluted with 10% to 40% EtOAc/n-hexane, and the fractions incruding product were collected and evaporated by rotary evaporation. To the residue was added 14% EtOAc/n-hexane, and the resulting precipitates were collected by filtration to afford 11 (2.94 g, 93%); a colorless powder; 1H NMR (600 MHz, CDCl3) δ 2.83‒2.92 (m, 2H), 3.79‒3.91 (m, 2H), 4.69‒4.83 (m, 2H), 6.88‒6.98 (m, 2H), 7.09‒7.19 (m, 2H), 7.47‒7.54 (m, 1H), 7.74‒7.83 (m, 1H); 13C NMR (151 MHz, CDCl3) δ 27.2, 28.6, 41.0, 42.7, 44.7, 46.1, 116.3, 116.4, 117.2, 117.3, 124.7, 124.8, 124.9, 125.0, 131.3, 132.5, 132.7, 133.0, 133.6, 138.4, 160.1, 161.8. HRMS (ESI/APCI dual) m/z Calcd for C17H13BrF4N2O3S: (M+H)+, 480.9839. Found: 480.9836.
N-(4-Fluorophenyl)-2-(trifluoroacetyl)-1,2,3,4-tetrahydroisoquinoline-6-sulfonamide (12). To a solution of 11 (900 mg, 1.87 mmol) in EtOH (15 mL) and EtOAc (15 mL) was added ten-percent palladium activated carbon (450 mg), and the mixture was stirred under a hydrogen atmosphere at room temperature for 15 h. The mixture was filtered through a pad of Celite® and the filtrate was concentrated under reduced pressure. The resulting residue was purified using a silica gel column eluted with 40% to 50% EtOAc/n-hexane to afford 12 (738 mg, 98%); a colorless powder; 1H NMR (600 MHz, CDCl3) δ 2.92‒2.98 (m, 2H), 3.82‒3.92 (m, 2H), 4.75‒4.85 (m, 2H), 6.63 (br s, 1H), 6.93‒6.99 (m, 2H), 7.01‒7.08 (m, 2H), 7.17‒7.25 (m, 1H), 7.53‒7.59 (m, 2H); 13C NMR (151 MHz, CDCl3) δ 27.8, 29.2, 41.2, 42.9, 45.4, 116.2, 116.4, 124.8, 124.9, 125.0, 125.6, 126.9, 127.5, 127.6, 127.8, 131.9, 134.5; MS (ESI/APCI dual) m/z 403 (M+H)+. HRMS (ESI/APCI dual) m/z Calcd for C17H14F4N2O3S: (M+H)+, 403.0734. Found: 403.0731.
Debromination using hydrogenolysis; General procedure
To a solution of 7-bromo-1,2,3,4-tetrahydroisoquinolne derivatives (0.312 mmol) in EtOH (2.5 mL) and EtOAc (2.5 mL) was added ten-percent palladium activated carbon (10 wt%) and triethylamine (52.1 µL, 0.374 mmol). The mixture was stirred under a hydrogen atmosphere at room temperature for 1 h. The mixture was filtered through a pad of Celite® and the filtrate was concentrated under reduced pressure. The resulting residue was purified using a silica gel column to afford the desired product.
N-[4-(3-Cyclohexylpropyl)-2-fluorophenyl]-2-(trifluoroacetyl)-1,2,3,4-tetrahydroisoquinoline-6-sulfonamide (Table 2, Entry 3): a colorless powder; 1H NMR (600 MHz, CDCl3) δ 0.76‒0.91 (m, 2H), 1.07‒1.24 (m, 6H), 1.51‒1.59 (m, 2H), 1.60‒1.74 (m, 5H), 2.47‒2.53 (m, 2H), 2.91‒2.99 (m, 2H), 3.81‒3.92 (m, 2H), 4.73‒4.85 (m, 2H), 6.72 (br s, 1H), 6.76‒6.81 (m, 1H), 6.89‒6.94 (m, 1H), 7.16‒7.23 (m, 1H), 7.42‒7.48 (m, 1H), 7.56‒7.53 (m, 2H); 13C NMR (151 MHz, CDCl3) δ 14.1, 26.3, 26.6, 27.7, 28.3, 33.3, 35.5, 36.9, 37.4, 41.2, 42.9, 45.3, 60.4, 115.2, 115.3, 117.3, 121.3, 121.4, 124.1, 124.2, 124.7, 125.4, 125.5, 126.8, 127.3, 127.5, 127.7, 134.7, 135.2, 136.6, 136.9, 137.8, 138.3, 142.6, 142.6, 142.7, 153.6, 155.2, 155.9, 156.1. HRMS (ESI/APCI dual) m/z Calcd for C26H30F4N2O3S: (M+H)+, 527.1986. Found: 527.1975.
N-[4-(2-Cyclopentylethyl)-2-fluorophenyl]-2-(trifluoroacetyl)-1,2,3,4-tetrahydroisoquinoline-6-sulfonamide (Table 2, Entry 4): a colorless powder; 1H NMR (600 MHz, CDCl3) δ 1.03‒1.16 (m, 2H), 1.43‒1.80 (m, 9H), 2.50‒2.59 (m, 2H), 2.90‒3.01 (m, 2H), 3.80‒3.92 (m, 2H), 4.74‒4.84 (m, 2H), 6.65 (br s, 1H), 6.76‒6.83 (m, 1H), 6.89‒6.96 (m, 1H), 7.14‒7.25 (m, 1H), 7.42‒7.49 (m, 1H), 7.55‒7.64 (m, 2H); 13C NMR (151 MHz, CDCl3) δ 25.2, 27.7, 29.1, 32.6, 34.5, 37.6, 39.5, 41.2, 42.9, 45.4, 46.8, 115.1, 115.3, 124.2, 124.7, 125.5, 126.9, 127.3, 127.5, 127.7, 134.4, 137.0, 137.8, 142.8. HRMS (ESI/APCI dual) m/z Calcd for C24H26F4N2O3S: (M+H)+, 499.1673. Found: 499.1665.
N-[4-(2-Cyclopropylethyl)-2-fluorophenyl]-2-(trifluoroacetyl)-1,2,3,4-tetrahydroisoquinoline-6-sulfonamide (Table 2, Entry 5): a colorless powder; 1H NMR (600 MHz, CDCl3) δ -0.04‒0.00 (m, 2H), 0.37‒0.43 (m, 2H), 0.59‒0.68 (m, 1H), 1.42‒1.49 (m, 2H), 2.61‒2.69 (m, 2H), 2.90‒3.01 (m, 2H), 3.81‒3.96 (m, 2H), 4.73‒4.89 (m, 2H), 6.70‒6.76 (m, 1H), 6.78‒6.85 (m, 1H), 6.91‒6.98 (m, 1H), 7.17‒7.25 (m, 1H), 7.43‒7.50 (m, 1H), 7.56‒7.64 (m, 2H); 13C NMR (151 MHz, CDCl3) δ 27.7, 29.1, 30.6, 31.3, 35.3, 36.2, 41.2, 42.9, 45.4, 46.8, 115.3, 115.4, 121.3, 124.1, 124.2, 124.8, 125.5, 126.8, 127.3, 127.5, 127.7, 134.4, 135.2, 136.9, 137.8, 153.5, 155.1. HRMS (ESI/APCI dual) m/z Calcd for C22H22F4N2O3S: (M+H)+, 471.1360. Found: 471.1353.
Synthesis of compound 1 on a 100 gram-scale
1-(7-Bromo-3,4-dihydroisoquinolin-2(1H)-yl)-2,2,2-trifluoroethanone (9). Pyridine (41.7 mL, 519 mmol) and 4-N,N-dimethylaminopyridine (576 mg, 4.72 mmol) were added to a solution of 7-bromo-1,2,3,4-tetrahydroisoquinoline (100 g, 472 mmol) in CHCl3 (600 mL). After cooling to 0 °C, trifluoroacetic acid anhydride (66.5 mL, 481 mmol) was added in a dropwise manner to the mixture. The mixture was warmed to room temperature and stirred for 15 h, then concentrated under reduced pressure. To the resulting residue was added 1 mol/L hydrochloric acid, and the mixture was extracted with EtOAc. The organic layer was washed with 1 mol/L hydrochloric acid and brine, dried over anhydrous MgSO4, filtered, and concentrated under reduced pressure. The resulting residue was diluted with CHCl3, and the mixture was washed with saturated aqueous NaHCO3. The organic layer was dried over anhydrous MgSO4, filtered, and concentrated under reduced pressure to afford crude 9 (143 g, 98%) as a pale yellow oil.
7-Bromo-2-(trifluoroacetyl)-1,2,3,4-tetrahydroisoquinoline-6-sulfonyl chloride (10). Chlorosulfonic acid (180 mL, 2.71 mol) was added in a dropwise manner to a solution of 9 (139 g, 452 mmol) in CHCl3 (180 mL) at room temperature, and the mixture was stirred at 60 °C for 5 h. After cooling to room temperature, the reaction mixture was added in a dropwise manner to ice water. CHCl3 (1.5 L) and water (500 mL) were added to the mixture, and the mixture was stirred at 40 °C. The mixture was separated, and the aqueous layer was extracted with CHCl3. The organic layers were combined, washed with water, and concentrated under reduced pressure. To the residue was added EtOAc (89 mL) and diisopropyl ether (520 mL), and the mixture was stirred at room temperature for 20 h. The resulting precipitates were collected by filtration to afford 10 (98.9 g, 54%) as a pale yellow powder. The filtrate was concentrated under reduced pressure, and EtOAc (40 mL) and n-hexane (120 mL) was added to the resulting residue; the mixture was then stirred at room temperature for 20 h. The resulting precipitates were collected by filtration to afford 10 (11.2 g, 6%) as a pale yellow powder (total: 110 g, 60%).
4-(3-Cyclopentylpropyl)-2-fluoroaniline (13). 9-Borabicyclo[3,3,1]nonane (9-BBN) (THF solution, 0.5 mol/L, 1.81 mL, 907 mmol) was added in a dropwise manner to a solution of allylcyclopentane (100 g, 907 mmol) in THF (400 mL) at 0 °C under a nitrogen atmosphere. The mixture was warmed gradually to room temperature and stirred overnight. After the reaction mixture was cooled to 0 °C, dichloro[1,1‘-bis(diphenylphosphino)ferrocene]palladium (II) (20.2 g, 24.8 mmol), 4-bromo-2-fluoroaniline (157 g, 825 mmol), and 3 mol/L aqueous sodium hydroxide (827 mL, 2.48 mol) were added to the reaction mixture, and the mixture was heated at the reflux temperature for 2 h. After cooling to room temperature, the mixture was concentrated under reduced pressure, and the resulting residue was diluted with EtOAc. The mixture was washed with water, and the aqueous layer was filtered through a pad of Celite® and extracted with EtOAc. The combined organic layers were washed with brine, and the aqueous layer was filtered through a pad of Celite® and extracted with EtOAc. The combined organic layers were concentrated under reduced pressure, and the resulting residue was purified using a silica gel column eluted with 10% to 24% EtOAc/n-hexane. The fractions incruding product were collected and evaporated by rotary evaporation, and to the residue was added 50% EtOAc/n-hexane and NH silica gel (150 g). The mixture was stirred at room temperature for 0.5 h, filtered, and concentrated under reduced pressure to afford crude 13 (201 g) as an orange oil.
To a solution of 13 (201 g) in EtOAc (1.5 L) was added 4 mol/L hydrogen chloride in EtOAc (309 mL), and the mixture was stirred overnight at room temperature. After the volatiles were removed by rotary evaporation, EtOAc (500 mL) was added to the residue, and the resulting precipitates were collected by filtration to afford the monohydrochloride salt of 13 (137 g, 64%); a colorless powder; mp 118‒120 °C; 1H NMR (600 MHz, DMSO-d6) δ 0.97‒1.09 (m, 2H), 1.23‒1.32 (m, 2H), 1.41‒1.61 (m, 6H), 1.66‒1.79 (m, 3H), 2.58 (t, J = 7.6 Hz, 2H), 7.06‒7.11 (m, 1H), 7.20‒7.25 (m, 1H), 7.41‒7.48 (m, 1H); 13C NMR (151 MHz, DMSO-d6) δ 24.7, 29.8, 32.2, 34.6, 35.0, 115.9, 116.0, 118.8, 118.9, 124.1, 124.9, 143.6, 153.9, 155.6. HRMS (ESI/APCI dual) m/z Calcd for C14H20FN: (M+H)+, 222.1653. Found: 222.1653.
7-Bromo-N-[4-(3-cyclopentylpropyl)-2-fluorophenyl]-2-(trifluoroacetyl)-1,2,3,4-tetrahydroisoquino-line-6-sulfonamide (14). Pyridine (36.2 mL, 450 mmol) was added to a solution of 13 (47.3 g, 184 mmol) in CHCl3 (450 mL) at 0 °C. A solution of 10 (73.2 g, 180 mmol) in CHCl3 (150 mL) was added to the mixture, and the mixture was warmed to room temperature and stirred for 4 h. To the mixture was added 1 mol/L hydrochloric acid (200 mL) and 3 mol/L hydrochloric acid (150 mL) at 0 °C, and the mixture was extracted twice with CHCl3. The aqueous layer was extracted with EtOAc, and the organic layers were combined and concentrated under reduced pressure to afford crude 14 (119 g); an orange powder; 1H NMR (600 MHz, CDCl3) δ 0.98‒1.08 (m, 2H), 1.24‒1.31 (m, 2H), 1.44‒1.60 (m, 6H), 1.67‒1.76 (m, 3H), 2.50 (t, J = 7.6 Hz, 2H), 2.87‒2.93 (m, 2H), 3.80‒3.90 (m, 2H), 4.72‒4.82 (m, 2H), 6.79‒6.89 (m, 2H), 7.25 (s, 1H), 7.34‒7.40 (m, 1H), 7.48‒7.53 (m, 1H), 7.81‒7.87 (m, 1H); 13C NMR (151 MHz, DMSO-d6) δ 14.2, 21.0, 25.1, 27.3, 28.6, 30.1, 32.6, 35.5, 35.6, 39.9, 41.0, 42.8, 44.7, 46.1, 115.4, 115.5, 117.2, 117.8, 121.0, 123.4, 123.6, 124.6, 132.1, 132.3, 132.6, 133.1, 133.9, 137.0, 137.4, 137.8, 138.3, 142.5, 153.5, 155.1. HRMS (ESI/APCI dual) m/z Calcd for C25H27BrF4N2O3S: (M+H)+, 591.0935. Found: 591.0927.
N-[4-(3-Cyclopentylpropyl)-2-fluorophenyl]-2-(trifluoroacetyl)-1,2,3,4-tetrahydroisoquinoline-6-sulfonamide (6). To a solution of 14 (118 g) in EtOH (400 mL) and EtOAc (100 mL) was added ten-percent palladium activated carbon (12.0 g) and triethylamine (27.6 mL, 198 mmol). The mixture was then stirred under a hydrogen atmosphere at room temperature for 1 h. Celite® was added to the mixture, and the mixture was filtered and concentrated under reduced pressure. The resulting residue was diluted with CHCl3, and to the mixture was 1 mol/L hydrochloric acid (600 mL) at 0 °C. The mixture was extracted with CHCl3 (twice) and EtOAc. The organic layers were combined and concentrated under reduced pressure. The resulting residue was purified using a silica gel column eluted with 20% to 50% EtOAc/n-hexane to afford 6 (89.4 g, 97% in 2 steps) as a pale yellow gum.
N-[4-(3-Cyclopentylpropyl)-2-fluorophenyl]-1,2,3,4-tetrahydroisoquinoline-6-sulfonamide (7). An aqueous solution (100 mL) of potassium hydroxide (11.7 g, 209 mmol) was added to a suspension of 6 (89.4 g, 174 mmol) in EtOH (400 mL) at 0 °C. The mixture was warmed to room temperature and stirred for 16 h. The mixture was concentrated under reduced pressure, and the resulting residue was diluted with water (500 mL). The mixture was neutralized with an aqueous solution of KHSO4 (49.67 g, 365 mmol), and the resulting precipitates were collected by filtration to afford 7 (78.3 g, quant.) as a colorless powder.
2-[2-(4-tert-Butylphenyl)ethyl]-N-[4-(3-cyclopentylpropyl)-2-fluorophenyl]-1,2,3,4-tetrahydroisoquinoline-6-sulfonamide (1). To a solution of 7 (78.3 g, 174 mmol) in CHCl3 (500 mL) were added a solution of 2-(4-(tert-butyl)phenyl)acetaldehyde (33.8 g, 192 mmol) in CHCl3 (100 mL) and sodium triacetoxyborohydride (55.4 g, 262 mmol), and the mixture was stirred at room temperature for 4 h. To the mixture were added 2-(4-(tert-butyl)phenyl)acetaldehyde (6.61 g, 37.5 mmol) and sodium triacetoxyborohydride (18.5 g, 87.2 mmol), and the mixture was stirred at room temperature for 18 h. Saturated aqueous NaHCO3 (1 L) was added to the reaction mixture, and the mixture was extracted three times with CHCl3. The organic layer was concentrated under reduced pressure, and the resulting residue was purified using a silica gel column eluted with 30% to 50% EtOAc/n-hexane to afford 1 (88.1 g, 88%) as a pale yellow amorphous.
To a solution of 1 in EtOAc (400 mL) was added 4 mol/L hydrogen chloride in EtOAc (57.3 mL), and the mixture was stirred at room temperature for 20 h. After the volatiles were removed by rotary evaporation, EtOAc was added to the residue, and the mixture was stirred at 0 °C. The resulting precipitates were collected by filtration to afford the monohydrochloride salt of 1 (84.5 g, 90%) as a colorless powder.
ACKNOWLEDGEMENTS
We are grateful to Dr. N. Ohtake and Dr. T. Yoshizumi for their critical reading of the manuscript and valuable suggestions. We are grateful to Dr. N. Sato for helpful suggestions about compound design and synthesis. We acknowledge the computational analysis by Dr. S. Tokura, the structural determination (NMR experiments) by Dr. A. Okada, and the analytical supports (the high-resolution mass spectra) by Mr. A. Higuchi.
References
1. J. D. Scott and R. M. Williams, Chem. Rev., 2002, 102, 1669. CrossRef
2. R. K. Tiwari, D. Singh, J. Singh, A. K. Chhillar, R. Chandra, and A. K. Verma, Eur. J. Med. Chem., 2006, 41, 40. CrossRef
3. I. Gavras, D. Vlahakos, J. C. Melby, and H. Gavras, J. Clin. Pharm., 1984, 24, 343. CrossRef
4. T. Onoda, Y. Takikawa, T. Fujimoto, Y. Yasui, K. Suzuki, and T. Matsumoto, Synlett, 2009, 1041. CrossRef
5. M. S. Buchanan, R. A. Davis, S. Duffy, V. M. Avery, and R. J. Quinn, J. Nat. Prod., 2009, 72, 1541. CrossRef
6. T. Busujima, H. Tanaka, K. Iwakiri, Y. Shirasaki, E. Munetomo, M. Saito, A. Masuko, K. Kitano, F. Io, K. Kato, S. Kamigaso, A. Nozoe, and N. Sato, Chem. Pharm. Bull., 2016, in press.
7. C.-L. E. Yen and R. V. Farese Jr., J. Biol. Chem., 2003, 278, 18532. CrossRef
8. C.-L. E. Yen, M.-L. Cheong, C. Grueter, P. Zhou, J. Moriwaki, J. S. Wong, B. Hubbard, S. Marmor, and R. V. Farese Jr., Nat. Med., 2009, 15, 442; CrossRef D. W. Nelson, Y. Gao, N. M. Spencer, T. Banh, and C.-L. E. Yen, J. Lipid Res., 2011, 52, 1723; CrossRef C.-L. E. Yen, D. W. Nelson, and M.-I. Yen, J. Lipid Res., 2015, 56, 489. CrossRef
9. J. G. Barlind, L. K. Buckett, S. G. Crosby, Ö. Davidsson, H. Emtenäs, A. Ertan, U. Jurva, M. Lemurell, P. M. Gutierrez, K. Nilsson, G. O’Mahony, A. U. Petersson, A. Redzic, F. Wågberg, and Z.-Q. Yuan, Bioorg. Med. Chem. Lett., 2013, 23, 2721. CrossRef
10. K. Sato, H. Takahagi, T. Yoshikawa, S. Morimoto, T. Takai, K. Hidaka, M. Kamaura, O. Kubo, R. Adachi, T. Ishii, T. Maki, T. Mochida, S. Takekawa, M. Nakakariya, N. Amano, and T. Kitazaki, J. Med. Chem., 2015, 58, 3892; CrossRef K. Sato, H. Takahagi, O. Kubo, K. Hidaka, T. Yoshikawa, M. Kamaura, M. Nakakariya, N. Amano, R. Adachi, T. Maki, K. Take, S. Takekawa, T. Kitazaki, and T. Maekawa, Bioorg. Med. Chem., 2015, 23, 4544. CrossRef
11. T. Busujima, H. Tanaka, Y. Shirasaki, E. Munetomo, M. Saito, K. Kitano, T. Minagawa, K. Yoshida, N. Osaki, and N. Sato, Bioorg. Med. Chem., 2015, 23, 5922. CrossRef
12. A. E. Reed, L. A. Curtiss, and F. Weinhold, Chem. Rev., 1988, 88, 899. CrossRef
13. H. Sajiki, A. Kume, K. Hattori, and K. Hirota, Tetrahedron Lett., 2002, 43, 7247. CrossRef