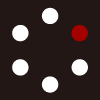
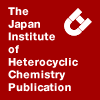
HETEROCYCLES
An International Journal for Reviews and Communications in Heterocyclic ChemistryWeb Edition ISSN: 1881-0942
Published online by The Japan Institute of Heterocyclic Chemistry
e-Journal
Full Text HTML
Received, 11th December, 2015, Accepted, 20th January, 2016, Published online, 4th February, 2016.
DOI: 10.3987/COM-15-13394
■ Inter- and Intramolecular Diels-Alder Reaction of Ethenetricarboxylate Derivatives
Shoko Yamazaki,* Hirotaka Sugiura, Mamiko Niina, Yuji Mikata, and Akiya Ogawa
Department of Chemistry, Nara University of Education, Takabatake-cho, Nara 630-8528, Japan
Abstract
Inter- and intramolecular [4+2] cycloaddition reactions of highly electron-deficient ethenetricarboxylates have been studied. Intermolecular Diels-Alder reaction of ethenetricarboxylate esters and cyclopentadiene proceeded at room temperature or -20 °C to give cycloadducts with 1:1.5-1.9 endo:exo ratio. Lewis acids such as EtAlCl2, Zn(OTf)2 and Cu(OTf)2 catalyzed reaction at room temperature or -40 °C gave cycloadducts with 3.1-5.4:1 endo:exo ratio. Reaction of N-benzyl- or N-allyl-2-furylmethylamine and 1,1-diethyl 2-hydrogen ethenetricarboxylate in the presence of EDCI/HOBt/Et3N at room temperature led directly to an intramolecular Diels-Alder adduct stereoselectively. The observed stereoselectivities were explained by the use of DFT calculations.introduction
Diels-Alder reaction is one of the most widely used synthetic tools.1 The alkene component (dienophile) is usually electron-deficient in normal electron-demand Diels-Alder reaction. In general, the greater the number of electron-withdrawing substituents on the double bond, the more reactive is the dienophile, owing to the lowering of the energy of the LUMO of the dienophile by the substituents. Ethenetricarboxylate derivatives bearing three carbonyl groups have been employed as highly electrophilic C=C components in various bond-forming reactions.2 Although an intramolecular inverse electron demand hetero Diels-Alder reaction of 1-allylic 2,2-dimethyl esters of ethene-1,2,2-tricarboxylate has been studied,3 only a few normal electron demand Diels-Alder reactions of ethenetricarboxylate related compounds as electron-deficient dienophiles have been reported.4 Ethenetricarboxylates allow for the facile derivatization at 2-carboxyl group. The electron-deficient alkene moiety is expected to work as a reactive dienophile in the intramolecular Diels-Alder reaction.
The intramolecular Diels-Alder reaction of furans is used for facile formation of multicyclic skeletons.5 Furan derivatives can be obtained from renewable resources.6 The development of new synthetic methods utilizing furans is of considerable interest.7 Lewis acid-catalyzed reaction of furan derivatives with electron-deficient alkenes also gave Friedel-Crafts/conjugate addition products.8 The diversity of the reactivity of furans is also of interest.
In this work, intermolecular and sequential intramolecular Diels-Alder reactions of ethenetricarboxylate derivatives with dienes have been studied.
RESULTS AND DISCUSSION
We have reported Lewis acid-catalyzed intermolecular reaction of ethenetricarboxylates with furan derivatives to give Friedel-Crafts/ conjugate addition products.9 Both intermolecular Diels-Alder reactions of methylenemalonates /alkylidenemalonates and dienes10 such as butadiene, cyclopentadiene or 1-methoxy-3-trimethylsiloxy-1,3-butadiene and intramolecular Diels-Alder reactions of alkylidenemalonates and dienes11 were reported previously. First, intermolecular Diels-Alder reaction of ethenetricarboxylates and reactive dienes has been examined. The reaction of ethenetricarboxylate esters 1 and cyclopentadiene 2 proceeded at room temperature or -20 °C to give cycloadducts 3 with 1:1.5-1.9 endo:exo ratio (eq 1, Table 1). Endo and exo structures were assigned by observed NOEs between C3-H and C5-H for endo and between C3-H and C7-HH for exo. Lewis acids such as Zn(OTf)2 and Cu(OTf)2 catalyzed reaction at room temperature gave cycloadducts 3 with 3.1-3.3:1 endo:exo ratio. EtAlCl2 catalyzed reaction at lower temperature increased endo:exo ratio. Thus, the uncatalyzed and Lewis acid-catalyzed Diels-Alder reactions of ethenetricarboxylates 1 and cyclopentadiene 2 showed opposite preference for stereoselectivity.
Treatment of cycloadduct 3b (1 : 1.8 endo:exo ratio) obtained by entry 8 reaction condition with Zn(OTf)2 and Cu(OTf)2 at room temperature for 17 h resulted in very little change of the endo:exo ratio. The possible epimerization of cycloadducts 3 by retro-[4+2]/[4+2]-cycloaddition10c may be very slow at room temperature in the presence of these Lewis acids.
In order to explain the observed endo:exo preferences,12 the reaction mechanism was examined using B3LYP/6-31G*13,14 calculations including the PCM15 solvent effect (solvent=CH2Cl2). TS geometry was characterized by vibrational analysis, which checked whether the obtained geometry has single imaginary frequencies (ν‡). From TSs, reaction paths were traced by the intrinsic reaction coordinate (IRC) method16 to obtain the energy-minimum geometries. Relative Gibbs free energies are of RB3LYP/6-31G* SCRF = (PCM, solvent = CH2Cl2) (T = 298.15 K, P = 1 atm).
First, the conformations of compound 1 were calculated through the use of model compound trimethyl ethenetricarboxylate 1m. Stable four conformations of 1m with respect to s-cis and s-trans of ester C=O groups were obtained by geometry optimization. In the optimized structures, 1-syn ester group to 2-ester is located almost perpendicularly to C=C plane. None of C=O of 1-syn ester on the C=C plane was obtained, probably because of steric congestion. The energy differences of C=C−C=O s-cis and s-trans are small (within +1.73 kcal/mol) (Figure 1). Using the most stable conformation of 1m(cc) (cc = 1-anti-s-cis, 2-s-cis), the endo and exo transition states of the intermolecular Diels-Alder reaction of 1m and cyclopendtadiene (2) were calculated (Figure 2). The activation energy ΔG‡ of endo transition state (TS) is 1.03 kcal/mol higher than that of exo TS. The exo preference is in accord with the experimental results.
Although 1-syn ester group is perpendicular to C=C plane, it has still an electron-withdrawing effect. C-2 carbon may interact with diene first by the slight asynchronous approach (C2-C1' = 2.168 Å, C1-C4' = 2.327 Å for 2-ester exo TS, C2-C1' = 2.219 Å, C1-C4' = 2.291 Å for 2-ester endo TS). The exo approach on 2-ester and 1-syn ester is considered to be endo approach on 1-anti ester group. The secondary orbital interaction of 1-anti ester carbonyl carbon with diene carbon in 2-ester endo TS may be slightly more effective than that of 2-ester carbonyl carbon in 2-ester endo TS (OC-C3' = 2.973 Å for 2-ester exo TS, OC-C2' = 2.997 Å for 2-ester endo TS).
Next, Lewis acid-catalyzed Diels-Alder reaction of 1m and cyclopendtadiene (2) was examined using the bidentate AlCl3-coordinated 1m shown in Figure 3 as a model system.17,18 In the initial AlCl3-1m and 2 complex, 2-ester group is located perpendicularly to C=C plane (1,1-diester C=O groups are both s-trans to C=C). Stepwise mechanism with zwitterionic intermediates was obtained.19 Lewis acid-catalyzed reaction lowers the activation energies. The activation energy ΔG‡ of endo TS1 is 1.16 kcal/mol lower than that of exo TS1. The endo preference is in accord with the experimental results. The endo preference may arise from the steric congestion of 2-ester group and methylene hydrogen of cyclopentadiene in exo TS. The endo preference in the kinetic product has also been reported in the TiCl4-catalyzed cycloaddition reaction of dimethyl benzylidenemalonate and cyclopentadiene at -78 °C.10c
The reaction of 1b and Danishefsky's diene has also been carried out. The reaction of 1b and 1-methoxy-3-trimethylsiloxy-1,3-butadiene 4 in dichloromethane proceeded at room temperature to give the crude adduct 5, which was hydrolyzed directly with 1 N HCl in THF to give the cyclohexenone 6 in 67% yield regioselectively.
Thus, it was shown that ethenetricarboxylates 1 have high reactivity towards Diels-Alder reaction with reactive dienes such as 2 and 4.
Next, intramolecular [4+2] cycloaddition reaction of ethenetricarboxylates has been examined. Reaction of N-benzyl- or N-allyl-2-furylmethylamine 8a,b and 1,1-diethyl 2-hydrogen ethenetricarboxylate 7 in the presence of EDCI/HOBt/Et3N at room temperature led directly to an intramolecular Diels-Alder adducts 9a,b in 65-82% yield (eq 3, Table 2). The possible intermediate 10 could not be observed under the reaction conditions of amide formation (Scheme 2). The reaction of 7 and 8a in the absence of condensation reagents only gave the mixture of the 7 and 8a, probably forming a salt. Treatment of the mixture with condensation reagents led to the Diels-Alder adduct 9a in 75% yield.
The structure of 10-oxa-3-aza-tricyclo[5.2.1.01,5]dec-8-ene 9a was determined by X-ray analysis (Figure 4).20 The exo stereochemistry of Diels-Alder adducts 9a-e with respect to the amide group was also determined by NOEs. NOEs between C5-H and C2-HH and/or between C5-H and C9-H were observed (atom numbering is shown in eq 3.).
In order to explain the stereoselectivity of the intramolecular Diels-Alder reaction was examined by B3LYP/6-31G* calculations including the PCM solvent effect (solvent=THF).
The endo and exo intramolecular Diels-Alder reactions from a model compound 10m as a possible intermediate were calculated (Figure 5). The activation energy ΔG‡ of endo TS (31.17 kcal/mol) is much higher than that of exo TS (21.41 kcal/mol). The acid-catalyzed intramolecular Diels-Alder reactions of 10m were also calculated (Figure 6). The protonated six-membered ring intermediates with hydrogen bonding were assumed.21 The acid in situ, possibly generating from EDCI (1-[3-(dimethylamino)propyl]-3-ethylcarbodiimide hydrochloride) or starting material 7 may catalyze the cycloaddition reactions. Stepwise mechanism with zwitterionic intermediates was obtained for the H+-catalyzed reaction. The acid-catalyzed reaction lowers the activation energies compared to the uncatalyzed reaction. The activation energy ΔG‡ of the second bond formation TS (H+IM-exo-TS2) (10.10 kcal/mol) is higher than that of the first TS (H+IM-exo-TS1) (1.51 kcal/mol) for exo addition. The H+-catalyzed process accelerates the formation of the exo adduct 9.
The first bond formation TS (H+IM-endo-TS1) for endo addition was obtained, however the second bond formation TS could not be obtained. Optimization of the initial structure of endo10m-H+ led to the intermediate (H+IM- endo-Int). The endo TS and product are highly unstable probably because of the steric constraint. Therefore, the exo adducts 9 produced stereoselectively.
Reaction of 1-phenylethyl-2-furylmethylamine or benzyl-1-(2-furyl)ethylamine 8c,d gave Diels-Alder adducts 9c,d with diastereomer ratios of 2:1-1:1 in 43-48% yield. Reaction of N-benzyl-(5-bromofuran-2-yl)methylamine 8e gave Diels-Alder adduct 9e at room temperature in THF for 1 h in 40%, for 20 h in 48% and at 80 °C in CH2ClCH2Cl22 for 20 h in 75%. A small amount of byproducts formed at room temperature possibly contain amine adducts at C=C bond. The reaction of 7 and 8b with DCC gave a complex mixture containing a small amount of DCU-incorporated byproducts.23
Next, formation and reaction of 3-substituted amide 12 was examined as a comparison. The 3-substituted amide 12 may not undergo the intramolecular Diels-Alder reaction by the high strain. Although the main objective of this study is to examine Diels-Alder reactions, examination of the selectivity of Diels-Alder vs Friedel-Crafts/Michael addition for furans are also of interest.8,24 Reaction of benzyl-3-furylmethylamine 11 and ethenetricarboxylate 7 in the presence of EDCI/HOBt/Et3N gave a non-cyclized amide 12. Lewis acid-catalyzed reaction of the amide 12 gave the Friedel-Crafts cyclized product 13 selectively in 74-76% yield (Scheme 3), similar to Lewis acid-catalyzed cyclization of phenyl-substituted ethenetricarboxylate derivatives.25 The highly strained Diels-Alder adduct 14 with 10-oxa-7-aza-tricyclo[3.3.1.13,9]dec-4-ene system was not formed.
Unlike 2-furylmethylamides of ethenetricarboxylate, 2-furylmethyl ester of ethenetricarboxylate 17 is a stable compound at room temperature and the similar thermal or Lewis acid-catalyzed reaction did not give [4+2] adduct (Scheme 4). The reaction of 3-furylmethyl ester of ethenetricarboxylate 19 under the thermal or Lewis acid-catalyzed reaction only gave starting materials along with decomposed products.
The difference on reactivity between oxygen and nitrogen analogues (intermediate 10 vs 17, 12 vs 19) can be explained, similar to the cyclization of other ethenetricarboxylate derivatives.26 Triesters may be more stable in s-cis conformation of O=C−O−CH2furanyl bond, probably because of the steric repulsion. In diester amides, the energy differences of s-cis and s-trans conformations of O=C−N−CH2furanyl may be small. The facile intramolecular reactions of amides probably originates from higher ratio of the reactive s-trans conformer.
On the other hand, in the reaction of diethyl ketomalonate 15 with (2E,4E)-hexa-2,4-dien-1-yl (triphenylphosphoranylidne)acetate (20) in benzene at room temperature or at 80 °C, tandem Wittig reaction/intramolecular Diels-Alder reaction proceeded to give 22 in 42 and 59% yields, respectively (Scheme 5). Intermediate 21 could not be isolated. The corresponding intermolecular Diels-Alder reaction of ester 1b and (2E,4E)-hexa-2,4-dien-1-ol 23 in CH2Cl2 at room temperature for 20 h did not proceed. The reaction at 80 °C (in 1,2-dichloroethane) or 110 °C (in toluene) caused decomposition of the substrates and did not give the Diels-Alder product.
In summary, inter- and intramolecular [4+2] cycloaddition reactions of ethenetricarboxylates have been studied. Intermolecular Diels-Alder reaction of ethenetricarboxylate esters and cyclopentadiene proceeded at room temperature or -20 °C to give cycloadducts with 1:1.5-1.9 endo:exo ratio. Lewis acids such as EtAlCl2, Zn(OTf)2 and Cu(OTf)2 catalyzed reaction at room temperature or -40 °C gave cycloadducts with 3.1-5.4:1 endo:exo ratio. Reaction of benzyl- or allyl-2-furylmethylamine and 1,1-diethyl 2-hydrogen ethenetricarboxylate in the presence of EDCI/HOBt/Et3N at room temperature led directly to intramolecular Diels-Alder adducts stereoselectively. On the other hand, reaction of benzyl 3-furylmethylamine and 1,1-diethyl 2-hydrogen ethenetricarboxylate under the condensation reaction conditions gave a non-cyclized amide. Lewis acid-catalyzed reaction of the amide gave the Friedel-Crafts cyclized product selectively. The highly functionalized cyclic compounds obtained in this study should be useful synthetic intermediates.
EXPERIMENTAL
General Methods. 1H Chemical shifts are reported in ppm relative to Me4Si. 13C Chemical shifts are reported in ppm relative to CDCl3 (77.1 ppm). 13C mutiplicities were determined by DEPT and HSQC. Peak assignments are made by 2D COSY, HSQC, NOESY, and HMBC spectra. Mass analyzer type used for EI is double-focusing in the HRMS measurements. Column chromatography was performed on silica gel (75-150 μm).
Ethenetricarboxylates 1a,b were prepared according to the literature.27
Typical experimental procedure (eq 1, entry 3). A solution of 1a (272 mg, 1.0 mmol) in CH2Cl2 (2 mL) was cooled to 0 °C. Zn(OTf)2 (36 mg, 0.1 mmol) was added to the solution, followed by cyclopentadiene (66 mg, 83 μL, 1.0 mmol; distilled prior to use). The mixture was allowed to warm to room temperature and stirred for 17 h. The reaction mixture was quenched by water and then saturated aqueous NaHCO3. The mixture was extracted with CH2Cl2 and the organic phase was dried (Na2SO4), and evaporated in vacuo. The residue was purified by column chromatography over silica gel with hexane-Et2O (2 : 1) as eluent to give 3a (312 mg, 92%, 3a-endo:3a-exo = 3.1 : 1) as an inseparable endo:exo mixture.
2,2-Diethyl 3-tert-butyl bicyclo[2.2.1]hept-5-ene-2,2,3-tricarboxylate (3a; endo:exo = 1 : 11): 3a (endo:exo = 1 : 11) was obtained by recrystallization of endo:exo = 1 : 1.5 mixture (entry 1). (3a; endo:exo = 1 : 11): Rf = 0.6 (hexane-Et2O = 2 : 1); colorless crystals; mp 58-62 °C (EtOAc); 1H NMR (400 MHz, CDCl3) δ (ppm) 1.22 (t, J = 7.1 Hz, 3H), 1.23 (t, J = 7.1 Hz, 3H), 1.44 (s, 9H), 1.56 (dddd, J = 9.2, 1.8, 1.8, 1.8 Hz, 1H), 2.31 (ddd, J = 9.2, 1.6, 1.6 Hz, 1H), 2.98 (bs, 1H), 3.22 (d, J = 2.0 Hz, 1H), 3.45 (bs, 1H), 3.96-4.04 (m, 1H), 4.07-4.21 (m, 2H), 4.24-4.33 (m, 1H), 5.99 (dd, J = 5.5, 2.8 Hz, 1H), 6.33 (dd, J = 5.5, 3.1 Hz, 1H). Selected NOEs are between δ 3.22 (C3-H) and δ 6.33 (C5-H), 2.98 (C4-H). Atom numbering is shown in eq 1.; 13C NMR (100.6 MHz, CDCl3) δ (ppm) 13.97 (q), 14.14 (q), 28.01 (q), 47.27 (t), 47.74 (d), 50.10 (d), 51.80 (d), 61.43 (t), 61.52 (t), 63.57 (s), 80.92 (s), 135.13 (d), 139.97 (d), 170.08 (s), 170.42 (s), 172.21 (s). Selected HMBC correlations are between δ 2.31 (C7-HH) and δ 47.74 (C4), 50.10 (C1), 135.13 (C6), 139.97 (C5), and between δ 3.22 (C3-H) and δ 47.74 (C4), 63.57 (C2), 135.13 (C6).; IR (neat) 2984, 1735, 1458, 1392, 1365, 1251, 1228, 1191, 1153, 1118, 1063, 1018 cm-1; MS (EI) m/z 338 (M+, 2.7), 282 (14), 264 (54), 237 (47), 217 (81), 171 (100%); HRMS (EI) M+ 338.1729 (calcd for C18H26O6 338.1729).
(3a; endo:exo = 3.2 : 1); Rf = 0.6 (hexane-Et2O = 2 : 1); colorless oil; 1H NMR (400 MHz, CDCl3) For 3a-endo, δ (ppm) 1.18 (t, J = 7.1 Hz, 3H), 1.26 (t, J = 7.1 Hz, 3H), 1.41 (s, 9H), 1.41 (bs, 2H), 3.15 (bs, 1H), 3.37 (bs, 1H), 3.88 (d, J = 3.5 Hz, 1H), 3.96-4.04 (m, 1H), 4.09-4.31 (m, 3H), 6.16 (dd, J = 5.5, 2.9 Hz, 1H), 6.54 (dd, J = 5.5, 3.1 Hz, 1H). Selected NOEs are between δ 3.88 (C3-H) and δ 3.15 (C4-H), 1.41 (C7-HH, overlapped).; 13C NMR (100.6 MHz, CDCl3) For 3a-endo, δ (ppm) 13.93 (q), 14.03 (q), 28.01 (q), 46.64 (t), 47.29 (d), 51.48 (d), 52.78 (d), 60.94 (t), 61.87 (t), 65.21 (s), 80.57 (s), 135.25 (d), 136.47 (d), 169.55 (s), 171.10 (s), 171.33 (s). Selected HMBC correlations are between δ 1.41 (C7-HH) and δ 52.78 (C3), 65.21 (C2), 135.25 (C5), 134.67 (C6) and between δ 3.88 (C3-H) and δ 47.29 (C4), 65.21 (C2), 135.25 (C5).; IR (neat) 2980, 1732, 1456, 1392, 1366, 1335, 1253, 1152, 1093, 1047 cm-1; MS (EI) m/z 338 (M+, 1.4), 282 (23), 264 (85), 237 (59), 217 (66), 171 (100%); HRMS (EI) M+ 338.1733 (calcd for C18H26O6 338.1729).
2,2,3-Triethyl bicyclo[2.2.1]hept-5-ene-2,2,3-tricarboxylate (3b; endo:exo = 1 : 1.8); Rf = 0.7 (hexane-Et2O = 1 : 2); colorless oil; 1H NMR (400 MHz, CDCl3) For a major isomer 3b-exo, δ (ppm) 1.21 (t, J = 7.1 Hz, 3H), 1.24 (t, J = 7.1 Hz, 3H), 1.27 (t, J = 7.1 Hz, 3H), 1.59 (dddd, J = 9.2, 1.8, 1.8, 1.8 Hz, 1H), 2.33 (ddd, J = 9.2, 1.6, 1.6 Hz, 1H), 3.03 (bs, 1H), 3.31 (d, J = 2.2 Hz, 1H), 3.47 (bs, 1H), 3.98-4.31 (m, 6H), 6.02 (dd, J = 5.7, 2.8 Hz, 1H), 6.33 (dd, J = 5.7, 3.1 Hz, 1H). Selected NOEs are between δ 3.31 (C3-H) and δ 6.33 (C5-H), 3.03 (C4-H).; 13C NMR (100.6 MHz, CDCl3) For a major isomer 3b-exo, δ (ppm) 13.96 (q), 14.12 (q), 14.21(q), 47.38 (d), 47.56 (t), 50.02 (d), 50.94 (d), 60.92 (t), 61.59 (t), 61.65 (t), 64.03 (s), 135.25 (d), 139.90 (d), 169.91 (s), 170.18 (s), 173.08 (s). Selected HMBC correlations are between δ 2.33 (C7-HH) and δ 135.25 (C6), 139.90 (C5), and between δ 3.31 (C3-H) and δ 47.56 (C7), 139.90 (C5).; IR (neat) 2983, 1735, 1465, 1372, 1254, 1200, 1119, 1096, 1034 cm-1; MS (EI) m/z 310 (M+, 6.8), 264 (11), 199 (27), 91 (51), 66 (100%); HRMS (EI) M+ 310.1415 (calcd for C16H22O6 310.1416); Anal. Calcd for C16H22O6: C, 61.92; H, 7.15. Found: C, 61.75; H, 7.39.
(3b; endo:exo = 3.3 : 1); Rf = 0.7 (hexane-Et2O = 1 : 2); colorless oil; 1H NMR (400 MHz, CDCl3) For a major isomer 3b-endo, δ (ppm) 1.19 (t, J = 7.1 Hz, 3H), 1.24 (t, J = 7.1 Hz, 3H), 1.27 (t, J = 7.1 Hz, 3H), 1.43 (ddd, J = 9.0, 1.7, 1.7 Hz, 1H), 1.47 (ddd, J = 9.0, 1.6, 1.6 Hz, 1H), 3.17 (bs, 1H), 3.40 (bs, 1H), 3.90 (d, J = 3.5 Hz, 1H), 4.00-4.30 (m, 6H), 6.24 (dd, J = 5.5, 3.0 Hz, 1H), 6.47 (dd, J = 5.5, 3.1 Hz, 1H). Selected NOEs are between δ 3.90 (C3-H) and δ 3.17 (C4-H), 1.47 (C7-HH).; 13C NMR (100.6 MHz, CDCl3) For a major isomer 3b-endo, δ (ppm) 13.96 (q), 14.05 (q), 14.21 (q), 46.81 (t), 46.88 (d), 51.46 (d), 52.25 (d), 60.57 (t), 61.15 (t), 62.00 (t), 65.54 (s), 135.97 (d), 136.19 (d), 169.30 (s), 171.26 (s), 172.00 (s). Selected HMBC correlations are between δ 1.43, 1.47 (C7-H2) and δ 51.46 (C1), 52.25 (C3), 135.97 (C5), 136.19 (C6) and between δ 3.90 (C3-H) and δ 46.88 (C4), 65.54 (C2), 135.97 (C5).; IR (neat) 2982, 1733, 1446, 1368, 1335, 1255, 1219, 1094, 1034 cm-1; MS (EI) m/z 310 (M+, 6.8), 264 (47), 237 (50), 199 (58), 91 (62), 66 (100%); HRMS (EI) M+ 310.1417 (calcd for C16H22O6 310.1416).
Triethyl 5-oxocyclohex-3-ene-1,2,2-tricarboxylate (6) (eq 2): To a solution of 1b (244 mg, 1 mmol) in CH2Cl2 (2 mL) was added 1-methoxy-3-(trimethylsilyloxy)-1,3-butadiene (4) (0.19 mL, 172.3 mg, 1 mmol). The mixture was stirred at room temperature for 22 h. The reaction mixture was concentrated under reduced pressure. To the residue was added THF (1 mL) containing a drop of 1N HCl and the mixture was stirred at room temperature for 10 min. The reaction mixture was concentrated under reduced pressure. The residue was purified by column chromatography over silica gel with hexane-Et2O (1 : 2) as eluent to give 6 (209 mg, 67%).
6: Rf = 0.6 (hexane-Et2O = 1 : 2); colorless oil; 1H NMR (400 MHz, CDCl3) δ (ppm) 1.23 (t, J = 7.1 Hz, 3H), 1.287 (t, J = 7.1 Hz, 3H), 1.293 (t, J = 7.1 Hz, 3H), 2.84 (ddd, J = 17.6, 6.1, 0.4 Hz, 1H), 2.99 (dd, J = 17.6, 5.5 Hz, 1H), 3.91 (ddd, J = 6.1, 5.5, 1.1 Hz, 1H), 4.14 (q, J = 7.1 Hz, 2H), 4.22-4.30 (m, 4H), 6.11 (dd, J = 10.3, 0.4 Hz, 1H), 7.21 (dd, J = 10.3, 1.1 Hz, 1H); 13C NMR (100.6 MHz, CDCl3) δ (ppm) 13.93 (q), 13.98 (q), 37.16 (t), 45.41 (d), 57.08 (s), 61.65 (t), 62.60 (t), 62.94 (t), 129.68 (d), 143.65 (d), 167.32 (s), 170.99 (s), 195.26 (s). Selected HMBC correlations are between δ 2.84, 2.99 (C6-H2) and δ 195.26 (C5), 57.08 (C2), 170.99 (CHCO2Et), between δ 6.11 (C4-H) and δ 37.16 (C6), 57.08 (C2) and between δ 7.21 (C3-H) and δ 195.26 (C5), 45.41 (C1), 167.32 (C(CO2Et)2). Atom numbering is shown in eq 2.; IR (neat) 2984, 1738, 1695, 1466, 1446, 1385, 1369, 1277, 1257, 1201, 1097, 1064, 1027 cm-1; MS (EI) m/z 312 (M+, 35), 267 (57), 167 (100%); HRMS (EI) M+ 312.1208 (calcd for C15H20O7 312.1209).
N-Allyl-furfurylamine (8a) was prepared by reaction of furfurylamine (2 equiv) with allyl bromide in Et2O according to the literature procedure.28
8a: (5.9 mmol scale, 266 mg, 33%): Rf = 0.4 (hexane-Et2O = 1 : 4); pale yellow oil; 1H NMR (400 MHz, CDCl3) δ (ppm) 1.74 (bs, 1H), 3.26 (ddd, J = 6.0, 1.6, 1.3 Hz, 2H), 3.79 (s, 2H), 5.12 (ddt, J = 10.3, 1.8, 1.3 Hz, 1H), 5.19 (ddt, J = 17.2, 1.8, 1.6 Hz, 1H), 5.90 (ddt, J = 17.2, 10.3, 6.0 Hz, 1H), 6.18 (ddt, J = 3.3, 0.9, 0.7 Hz, 1H), 6.31 (dd, J = 3.3, 1.8 Hz, 1H), 7.36 (dd, J = 1.8, 0.9 Hz, 1H); 13C NMR (100.6 MHz, CDCl3) δ (ppm) 45.35 (t), 51.41 (t), 107.09 (d), 110.15 (d), 116.46 (t), 136.36 (d), 141.89 (d), 153.71 (s); IR (neat) 3324, 3078, 2919, 2824, 1644, 1506, 1458, 1148, 1009, 921 cm-1; MS (EI) m/z 137 (M+, 62), 136 (100%); HRMS (EI) M+ 137.0830 (calcd for C8H11NO 137.0841).
N-Benzyl-furfurylamines 8b-e and 11 were prepared by reductive amination according to the literature procedure.29
N-Benzyl-furfurylamine (8b): (8.9 mmol scale, 1.62 g, 97%). 8b was also prepared by reaction of furfurylamine (2 equiv) with benzyl bromide in Et2O (5.0 mmol scale, 449 mg, 47%) according to the literature procedure.28
1H and 13C NMR of 8b were in accord with the reported data.29b
8b: Rf = 0.2 (hexane-Et2O = 1 : 1); pale yellow oil; 1H NMR (400 MHz, CDCl3) δ (ppm) 1.85 (bs, 1H), 3.78 (s, 4H), 6.18 (bd, J = 3.3 Hz, 1H), 6.31 (dd, J = 3.3, 1.8 Hz, 1H), 7.22-7.28 (m, 1H), 7.29-7.34 (m, 4H), 7.36 (dd, J = 1.8, 0.7 Hz, 1H); 13C NMR (100.6 MHz, CDCl3) δ (ppm) 45.37 (t), 52.81 (t), 107.12 (d), 110.15 (d), 127.08 (d), 128.32 (d), 128.46 (d), 139.86 (s), 141.88 (d), 153.80 (s).
N-Benzyl-1-(furan-2-yl)ethylamine (8c): (8.9 mmol scale, 976 mg, 54%): Rf = 0.5 (hexane-Et2O = 1 : 1); pale yellow oil; 1H NMR (400 MHz, CDCl3) δ (ppm) 1.43 (d, J = 6.8 Hz, 3H), 1.75 (bs, 1H), 3.66 (d, J = 13.1 Hz, 1H), 3.74 (d, J = 13.1 Hz, 1H), 3.88 (q, J = 6.8 Hz, 1H), 6.16 (ddd, J = 3.1, 0.8, 0.6 Hz, 1H), 6.32 (dd, J = 3.1, 1.8 Hz, 1H), 7.21-7.26 (m, 1H), 7.29-7.33 (m, 4H), 7.36 (dd, J = 1.8, 0.8 Hz, 1H); 13C NMR (100.6 MHz, CDCl3) δ (ppm) 20.51 (q), 50.60 (d), 51.21 (t), 105.54 (d), 109.95 (d), 126.99 (d), 128.28 (d), 128.46 (d), 140.34 (s), 141.47 (d), 157.85 (s); IR (neat) 3330, 3028, 2972, 2930, 1603, 1505, 1495, 1454, 1371, 1315, 1225, 1150, 1121, 1010 cm-1; MS (EI) m/z 201 (M+, 8.9), 186 (100), 91 (92%); HRMS (EI) M+ 201.1134 (calcd for C13H15NO 201.1154).
N-Furfuryl-1-phenylethylamine (8d): (8.9 mmol scale, 1.66 g, 93%): Rf = 0.5 (hexane-Et2O = 1 : 1); pale yellow oil; 1H NMR (400 MHz, CDCl3) δ (ppm) 1.36 (d, J = 6.6 Hz, 3H), 1.77 (bs, 1H), 3.58 (d, J = 14.5 Hz, 1H), 3.66 (d, J = 14.5 Hz, 1H), 3.78 (q, J = 6.6 Hz, 1H), 6.10 (dddd, J = 3.1, 0.8, 0.6, 0.6 Hz, 1H), 6.29 (dd, J = 3.1, 1.8 Hz, 1H), 7.23-7.28 (m, 1H), 7.31-7.36 (m, 5H); 13C NMR (100.6 MHz, CDCl3) δ (ppm) 24.30 (q), 44.05 (t), 57.15 (d), 106.90 (d), 110.13 (d), 126.85 (d), 127.12 (d), 128.57 (d), 141.82 (d), 145.08 (s), 154.03 (s); IR (neat) 3331, 3026, 2963, 2925, 1602, 1505, 1492, 1451, 1370, 1147, 1117, 1011 cm-1; MS (EI) m/z 201 (M+, 6.4), 186 (73), 81 (100%); HRMS (EI) M+ 201.1138 (calcd for C13H15NO 201.1154).
N-Benzyl-(5-bromofuran-2-yl)methylamine (8e): (9.0 mmol scale, 2.14 g, 89%): Rf = 0.3 (hexane-Et2O = 1 : 1); pale yellow oil; 1H NMR (400 MHz, CDCl3) δ (ppm) 1.75 (bs, 1H), 3.71 (s, 2H), 3.75 (s, 2H), 6.13 (d, J = 3.2 Hz, 1H), 6.20 (d, J = 3.2 Hz, 1H), 7.21-7.26 (m, 1H), 7.28-7.33 (m, 4H); 13C NMR (100.6 MHz, CDCl3) δ (ppm) 45.28 (t), 52.60 (t), 109.86 (d), 111.72 (d), 120.57 (s), 127.06 (d), 128.20 (d), 128.41 (d), 139.62 (s), 155.94 (s); IR (neat) 3329, 3027, 2917, 2830, 1602, 1505, 1453, 1200, 1125, 1010 cm-1; MS (EI) m/z 267 (M+, 12), 265 (M+, 12), 186 (11), 161 (21), 159 (22), 106 (23), 91 (100%); HRMS (EI) M+ 265.0099, 267.0045 (calcd for C12H12BrNO 265.0102, 267.0082).
N-Benzyl-furan-3-ylmethylamine (11): (9.2 mmol scale, 1.70 g, 99%): Rf = 0.6 (hexane-Et2O = 1 : 2); pale yellow oil; 1H NMR (400 MHz, CDCl3) δ (ppm) 1.64 (bs, 1H), 3.65 (dd, J = 0.6, 0.4 Hz, 2H), 5.80 (s, 2H), 6.39 (dd, J = 1.8, 0.8 Hz, 1H), 7.22-7.28 (m, 1H), 7.30-7.34 (m, 4H), 7.35 (ddd, J = 1.6, 0.8, 0.6 Hz, 1H), 7.38 (dd, J = 1.8, 1.6 Hz, 1H); 13C NMR (100.6 MHz, CDCl3) δ (ppm) 43.61 (t), 53.21 (t), 110.51 (d), 127.06 (d), 128.24 (d), 128.46 (s), 128.48 (d), 139.93 (d), 140.17 (s), 143.17 (d); IR (neat) 3315, 3027, 2921, 2823, 1602, 1496, 1453, 1362, 1158, 1108, 1065, 1021, 874 cm-1; MS (EI) m/z 187 (M+, 7.0), 186 (18), 106 (100), 91 (76), 81 (77%); HRMS (EI) M+ 187.0977 (calcd for C12H13NO 187.0997).
Typical experimental procedure for eq 3 and preparation of 12 in Scheme 3 (eq 3, Table 2, entry 1). To a solution of 1,1-diethyl 2-hydrogen ethenetricarboxylate (7) (313 mg, 1.45 mmol) (prepared from 1,1-diethyl 2-tert-butyl ethenetricarboxylate (1a) upon treatment with CF3CO2H)25 in THF (0.5 mL) were added N-allyl-furfurylamine (8a) (176 mg, 1.28 mmol) in THF (1.5 mL), Et3N (0.25 mL, 179 mg, 1.77 mmol), HOBt (1-hydroxybenzotriazole) (430 mg, 3.18 mmol) and EDCI (1-[3-(dimethylamino)propyl]-3-ethylcarbodiimide hydrochloride) (292 mg, 1.52 mmol) at 0 °C. The reaction mixture was stirred for 1 h at 0 °C, and was allowed to warm to room temperature and stirred for 17 h. The reaction mixture was concentrated under reduced pressure and the residue was diluted with CH2Cl2. The organic phase was washed with saturated aqueous NaHCO3 solution, 2M aqueous citric acid, saturated aqueous NaHCO3 and water, dried (Na2SO4), and evaporated in vacuo. The residue was purified by column chromatography over silica gel eluting with hexane-Et2O to give 9a (350 mg, 82%).
Diethyl 3-allyl-4-oxo-10-oxa-3-azatricyclo[5.2.1.01,5]dec-8-ene-6,6-dicarboxylate (9a): Rf = 0.3 (hexane-Et2O = 1 : 2); colorless crystals; mp 100-103 °C (EtOAc); 1H NMR (400 MHz, CDCl3) δ (ppm) 1.27 (t, J = 7.1 Hz, 3H), 1.30 (t, J = 7.1 Hz, 3H), 3.44 (s, 1H), 3.67 (d, J = 11.7 Hz, 1H), 3.88 (dddd, J = 15.6, 5.6, 1.5, 1.5 Hz, 1H), 3.94 (d, J = 11.7 Hz, 1H), 3.97 (dd, J = 15.6, 5.6 Hz, 1H), 4.11-4.37 (m, 4H), 5.20 (dddd, J = 10.1, 1.5, 1.3, 1.3 Hz, 1H), 5.23 (dddd, J = 17.0, 1.5, 1.3, 1.3 Hz, 1H), 5.36 (d, J = 1.6 Hz, 1H), 5.72 (dddd, J = 17.0, 10.1, 5.6, 5.6 Hz, 1H), 6.33 (dd, J = 5.7, 1.6 Hz, 1H), 6.61 (d, J = 5.7 Hz, 1H). Selected NOEs are between δ 3.44 (C5-H) and δ 3.94 (C2-HH), 6.61 (C9-H), between δ 3.94 (C2-HH) and δ 6.61 (C9-H), and between δ 6.33 (C8-H) and δ 5.36 (C7-H), 6.61 (C9-H). Atom numbering is shown in eq 3.; 13C NMR (100.6 MHz, CDCl3) δ (ppm) 13.72 (q), 13.77 (q), 44.87 (t), 47.92 (t), 54.34 (d), 61.60 (t), 61.75 (t), 62.04 (s), 83.20 (d), 89.36 (s), 117.58 (t), 131.43 (d), 135.26 (d), 136.89 (d), 167.86 (s), 168.52 (s), 169.49 (s). Selected HMBC correlations are between δ 3.44 (C5-H), 3.94 (C2-HH), 5.36 (C7-H), 6.33 (C8-H) and δ 136.89 (C9), between δ 3.94 (C2-HH), 5.36 (C7-H), 6.33 (C8-H) and δ 89.36 (C1) and between δ 3.44 (C5-H), 6.61 (C9-H), 6.33 (C8-H) and δ 83.20 (C7).; IR (KBr) 2997, 1730, 1691, 1480, 1365, 1266, 1107, 1066, 1039, 1000 cm-1; MS (EI) m/z 335 (M+); HRMS (EI) 335.1358 (calcd for C17H21NO6 M+ 335.1369); Anal. Calcd for C17H21NO6: C, 60.89; H, 6.31; N, 4.18. Found: C, 60.93; H, 6.08; N, 4.17.
9b: (2.35 mmol scale, 590 mg, 65%): Rf = 0.8 (CH2Cl2-Et2O = 1 : 1); colorless crystals; mp 138-140 °C (EtOAc); 1H NMR (400 MHz, CDCl3) δ (ppm) 1.32 (t, J = 7.1 Hz, 3H), 1.33 (t, J = 7.1 Hz, 3H), 3.47 (s, 1H), 3.59 (d, J = 11.9 Hz, 1H), 3.65 (d, J = 11.9 Hz, 1H), 4.11-4.40 (m, 5H), 4.68 (d, J = 15.0 Hz, 1H), 5.37 (d, J = 1.6 Hz, 1H), 6.32 (dd, J = 5.7, 1.6 Hz, 1H), 6.54 (d, J = 5.7 Hz, 1H), 7.23-7.36 (m, 5H). Selected NOEs are between δ 3.47 (C5-H) and δ 3.65 (C2-HH), 6.54 (C9-H), between δ 3.65 (C2-HH) and δ 3.47 (C5-H), and between δ 6.32 (C8-H) and δ 5.37 (C7-H), 6.54 (C9-H).; 13C NMR (100.6 MHz, CDCl3) δ (ppm) 14.07 (q), 14.09 (q), 46.76 (t), 48.13 (t), 54.69 (d), 61.99 (t), 62.23 (t), 62.38 (t), 83.58 (d), 89.49 (s), 127.74 (d), 128.00 (d), 128.88 (d), 135.73 (d), 135.89 (d), 136.96 (d), 168.20 (s), 168.86 (s), 170.01 (s). Selected HMBC correlations are between δ 3.59 (C2-HH), 3.65 (C2-HH), 5.37 (C7-H) and δ 136.96 (C9), between δ 3.59 (C2-HH), 6.54 (C9-H), 5.37 (C7-H), 6.32 (C8-H) and δ 89.49 (C1) and between δ 3.47 (C5-H), 6.54 (C9-H), 6.32 (C8-H) and δ 83.58 (C7).; IR (KBr) 2984, 1756, 1734, 1690, 1478, 1368, 1266, 1192, 1117, 1046 cm-1; MS (EI) m/z 385 (M+, 1.9), 340 (9.4), 295 (11), 248 (19), 221 (25), 200 (40), 186 (100%); HRMS (EI) 385.1521 (calcd for C21H23NO6 M+ 385.1525); Anal. Calcd for C21H23NO6: C, 65.44; H, 6.02; N, 3.63. Found: C, 65.50; H, 6.06; N, 3.76.
9c: (2 mmol scale, 381 mg, 48%, diastereomer ratio 2 : 1): Rf = 0.5 (hexane-Et2O = 1 : 3); pale yellow oil; 1H NMR (400 MHz, CDCl3) δ (ppm) 1.25-1.34 (m, 9H), 3.43 (s, 1H×0.33), 3.53 (s, 1H×0.67), 3.77 (q, J = 6.8 Hz, 1H×0.67), 3.97 (d, J = 15.2 Hz, 1H×0.33), 4.00 (q, J = 6.5 Hz, 1H×0.33), 4.10-4.41 (m, 4H+1H×0.67), 4.92 (d, J = 15.6, 1H×0.67), 5.06 (d, J = 15.2 Hz, 1H×0.33), 5.37 (d, J = 1.8 Hz, 1H×0.67), 5.39 (d, J = 1.8 Hz, 1H×0.33), 6.33 (dd, J = 5.7, 1.8 Hz, 1H×0.33), 6.34 (dd, J = 5.9, 1.8 Hz, 1H×0.67), 6.49 (d, J = 5.7 Hz, 1H×0.33), 6.59 (d, J = 5.9 Hz, 1H×0.67), 7.22-7.35 (m, 5H). Selected NOEs are between δ 3.43 (C5i-H) and δ 6.49 (C9i-H), between δ 3.53 (C5-H) and δ 6.59 (C9-H), 1.25-1.34 (C2-CH3, overlapped) and between δ 6.33, 6.34 (C8i,8-H) and δ 5.37, 5.39 (C7,7i-H). Atom numberings Cx and Cxi denote those of major and minor diastereomers, respectively.; 13C NMR (100.6 MHz, CDCl3) δ (ppm) 12.97 (q), 14.06 (q), 14.09 (q), 15.77 (q), 43.70 (t), 44.45 (t), 52.52 (d), 53.08 (d), 54.17 (d), 54.48 (d), 61.95 (t), 61.98 (t), 62.18 (t), 62.61 (s), 62.78 (s), 83.35 (d), 83.53 (d), 92.41 (s), 93.29 (s), 127.49 (d), 127.60 (d), 127.89 (d), 128.76 (d), 128.83 (d), 135.55 (d), 135.96 (d), 136.10 (s), 136.21 (d), 136.46 (s), 136.63 (d), 168.25 (s), 168.39 (s), 168.91 (s), 168.94 (s), 169.93 (s), 170.46 (s). Selected HMBC correlations are between δ 5.37, 5.39 (C7,7i-H) and δ 135.55, 136.63 (C9,9i), between δ 5.37, 5.39 (C7,7i-H), 6.33, 6.34 (C8i,8-H), 6.49, 6.59 (C9i,9-H), 1.25-1.34 (C2,2i-CH3, overlapped) and δ 92.41, 93.29 (C1i,1) and between δ 6.33, 6.34 (C8i,8-H), 6.49, 6.59 (C9i,9-H) and δ 83.35, 83.53 (C7i,7).; IR (neat) 2979, 1732, 1695, 1496, 1418, 1367, 1262, 1197, 1112, 1068, 1039 cm-1; MS (EI) m/z 399 (M+, 1.3), 304 (24), 200 (100%); HRMS (EI) 399.1689 (calcd for C22H25NO6 M+ 399.1682).
9d: (2 mmol scale, 340 mg, 43%, diastereomer ratio 1 : 1): Rf = 0.2 (hexane-Et2O = 1 : 2); pale yellow viscous oil; 1H NMR (400 MHz, CDCl3) δ (ppm) 1.26 (t, 7.1 Hz, 3H×0.5), 1.28 (t, J = 7.1 Hz, 3H×0.5), 1.30 (t, J = 7.1 Hz, 3H×0.5), 1.33 (t, J = 7.1 Hz, 3H×0.5), 1.53 (d, J = 7.0 Hz, 3H×0.5), 1.57 (d, J = 3H×0.5), 3.37 (d, J = 11.7 Hz, 1H×0.5), 3.41 (s, 1H×0,5), 3.44 (d, J = 11.7 Hz, 1H×0.5), 3.49 (s, 1H×0.5), 3.61 (d, J = 11.7 Hz, 1H×0.5), 3.82 (d, J = 11.7 Hz, 1H×0.5), 4.09-4.42 (m, 4H), 5.34 (d, J = 1.8 Hz, 1H×0.5), 5.35 (d, J = 1.8 Hz, 1H×0.5), 5.50 (q, J = 7.0 Hz, 1H×0.5), 5.53 (q, J = 7.0 Hz, 1H×0.5), 6.29 (dd, J = 5.7, 1.6 Hz, 1H×0.5), 6.31 (dd, J = 5.7, 1.6 Hz, 1H×0.5), 6.49 (d, J = 5.7 Hz, 1H×0.5), 6.56 (d, J = 5.7 Hz, 1H×0.5), 7.22-7.38 (m, 5H). Selected NOEs are between δ 3.41 (C5-H) and δ 6.49 (C9-H), between δ 3.49 (C5i-H) and δ 6.56 (C9i-H), between δ 3.37 (C2-HH) and δ 6.49 (C9-H), between δ 3.44 (C2i-HH) and δ 6.56 (C9i-H), and between δ 6.29, 6.31 (C8,8i-H) and δ 5.34, 5.35 (C7,7i-H), 6.49, 6.56 (C9,9i-H). Atom numberings Cx and Cxi denote those of two diastereomers, respectively.; 13C NMR (100.6 MHz, CDCl3) δ (ppm) 14.01 (q), 14.05 (q), 15.82 (q), 16.74 (q), 44.02 (t), 44.05 (t), 48.70 (d), 49.30 (d), 55.03 (d), 55.11 (d), 61.91 (t), 62.09 (t), 62.35 (s), 62.49 (s), 83.43 (d), 83.50 (d), 89.28 (s), 89.47 (s), 126.77 (d), 127.25 (d), 127.35 (d), 127.76 (d), 128.63 (d), 128.76 (d), 135.69 (d), 135.79 (d), 136.93 (d), 136.98 (d), 139.36 (s), 139.67 (s), 168.17 (s), 168.31 (s), 168.77 (s), 169.49 (s), 169.71 (s). Selected HMBC correlations are between δ 5.34, 5.35 (C7,7'-H) and δ 136.93, 136.98 (C9,9'), between δ 5.34, 5.35 (C7,7'-H), 6.49, 6.56 (C9,9'-H), 6.29, 6.32 (C8,8'-H) and δ 89.28, 89.47 (C1,1') and between δ 3.41, 3.49 (C5,5'-H), 6.49, 6.56 (C9,9'-H), 6.29, 6.32 (C8,8'-H) and δ 83.43, 83.50 (C7,7').; IR (neat) 2980, 1736, 1691, 1496, 1469, 1450, 1423, 1364, 1302, 1261, 1189, 1110, 1064, 1041 cm-1; MS (EI) m/z 399 (M+, 2.2), 354 (7.6), 294 (56), 200 (100%); HRMS (EI) 399.1685 (calcd for C22H25NO6 M+ 399.1682).
9e: (1 mmol scale, 227 mg, 48%): Rf = 0.1 (hexane-Et2O = 1 : 4); pale yellow crystals; mp 127-127.5 °C (EtOAc-Et2O = 1 : 1); 1H NMR (400 MHz, CDCl3) δ (ppm) 1.32 (t, J = 7.1 Hz, 3H), 1.33 (t, J = 7.1 Hz, 3H), 3.54 (s, 1H), 3.66 (d, J = 11.9 Hz, 1H), 3.78 (d, J = 11.9 Hz, 1H), 4.24-4.41 (m, 4H), 4.43 (d, J = 15.0 Hz, 1H), 4.61 (d, J = 15.0 Hz, 1H), 6.41 (d, J = 5.5 Hz, 1H), 6.54 (d, J = 5.5 Hz, 1H), 7.25-7.30 (m, 3H), 7.33-7.37 (m, 2H). Selected NOEs are between δ 3.54 (C5-H) and δ 6.54 (C9-H), between δ 3.78 (C2-HH) and δ 6.54 (C9-H), and between δ 6.41 (C8-H) and δ 6.54 (C9-H).; 13C NMR (100.6 MHz, CDCl3) δ (ppm) 13.96 (q), 14.05 (q), 46.77 (t), 48.09 (t), 57.80 (d), 62.42 (t), 62.56 (t), 66.86 (s), 87.69 (s), 91.14 (s), 127.86 (d), 128.02 (d), 128.94 (d), 135.50 (s), 137.23 (d), 140.81 (d), 166.06 (s), 167.52 (s), 169.23 (s). Selected HMBC correlations are between δ 3.78 (C2-HH) and δ 137.23 (C9), between δ 3.66 (C2-HH), 6.54 (C9-H), 6.41 (C8-H) and δ 87.69 (C1) and between δ 3.54 (C5-H), 6.54 (C9-H), 6.41 (C8-H) and δ 91.14 (C7).; IR (KBr) 2980, 1745, 1718, 1687, 1475, 1440, 1359, 1311, 1288, 1264, 1240, 1213, 1199, 1083, 1037 cm-1; MS (EI) m/z 465 (M+, 0.6), 463 (M+, 0.6), 438 (4.5), 436 (4.6), 266 (92), 264 (100%); HRMS (EI) 463.0648, 465.0623 (calcd for C21H22BrNO6 M+ 463.0631, 465.0610); Anal. Calcd for C21H22BrNO6: C, 54.32; H, 4.78; N, 3.02. Found: C, 54.22; H, 4.68; N, 3.05.
12: (3 mmol scale, 598 mg, 51%): Rf = 0.5 (hexane-Et2O = 1 : 3); pale yellow oil; 1H NMR (400 MHz, CDCl3) (2 rotamers, ratio 1.3 : 1) δ (ppm) 1.27-1.35 (m, 6H), 4.23-4.38 (m, 4H), 4.28 (s, 2H×0.4, minor rotamer), 4.40 (s, 2H×0.6, major rotamer), 4.49 (s, 2H×0.6), 4.62 (s, 2H×0.4), 6.29 (d, J = 0.8 Hz, 1H×0.4), 6.35 (d, J = 0.8 Hz, 1H×0.6), 7.19-7.43 (m, 8H); 13C NMR (100.6 MHz, CDCl3) δ (ppm) 13.98 (q), 14.02 (q), 14.04 (q), 38.86 (t), 42.17 (t), 47.49 (t), 50.54 (t), 62.00 (t), 62.22 (t), 62.27 (t), 109.68 (d), 110.93 (d), 120.14 (s), 120.35 (s), 127.10 (d), 127.79 (d), 128.14 (d), 128.49 (d), 128.76 (d), 129.10 (d), 134.57 (d), 134.73 (d), 135.25 (s), 135.28 (s), 135.56 (s), 136.30 (s), 140.57 (d), 141.26 (d), 143.49 (d), 144.17 (d), 162.92 (s), 162.97 (s), 164.36 (s), 164.45 (s), 164.47 (s), 164.62 (s); IR (neat) 2983, 1730, 1651, 1497, 1465, 1446, 1374, 1256, 1202, 1069, 1021 cm-1; MS (EI) m/z 385 (M+, 2.5), 340 (9.4), 248 (11), 200 (42), 186 (100%); HRMS (EI) 385.1514 (calcd for C21H23NO6 M+ 385.1525).
Diethyl 2-(5-benzyl-4,5,6,7-tetrahydro-6-oxofuro[3,2-c]pyridin-7-yl)malonate (13). To a solution of 12 (187 mg, 0.49 mmol) in CH2Cl2 (1 mL) was added Sc(OTf)3 (50 mg, 0.1 mmol). The mixture was stirred at room temperature overnight. The reaction mixture was quenched by water and then saturated aqueous NaHCO3. The mixture was extracted with CH2Cl2 and the organic phase was dried (Na2SO4), and evaporated in vacuo. The residue was purified by column chromatography over silica gel with hexane-Et2O (1 : 1) as eluent to give 13 (142 mg, 76%).
13: Rf = 0.3 (hexane-Et2O = 1 : 1); pale yellow oil; 1H NMR (400 MHz, CDCl3) δ (ppm) 1.21 (t, J = 7.1 Hz, 3H), 1.25 (t, J = 7.1 Hz, 3H), 4.15-4.30 (m, 6H), 4.33 (d, J = 3.9 Hz, 1H), 4.45 (ddd, J = 3.9, 3.9, 3.9 Hz, 1H), 4.75 (d, J = 14.8 Hz, 1H), 4.77 (d, J = 14.8 Hz, 1H), 6.20 (d, J = 1.8 Hz, 1H), 7.24-7.34 (m, 6H); 13C NMR (100.6 MHz, CDCl3) δ (ppm) 13.98 (q), 14.01 (q), 41.40 (d), 44.89 (t), 50.89 (t), 52.52 (d), 61.57 (t), 61.66 (t), 107.68 (d), 112.65 (s), 127.60 (d), 128.18 (d), 128.66 (d), 136.61 (s), 143.10 (d), 144.65 (s), 166.99 (s), 167.63 (s), 167.79 (s). Selected HMBC correlations are between δ 4.33 (CH(CO2Et)2), 6.20 (C3-H) and δ 144.65 (C7a), between δ 4.33 (CH(CO2Et)2) and δ 41.40 (C7), and between δ 6.20 (C3-H) and δ 112.65 (C3a). Atom numberings are shown in Scheme 8.; IR (neat) 2981, 1738, 1645, 1507, 1483, 1454, 1372, 1347, 1263, 1176, 1034 cm-1; MS (EI) m/z 385 (M+, 64), 340 (22), 293 (64), 266 (82), 91 (100%); HRMS (EI) M+ 385.1531 (calcd for C21H23NO6 385.1525).
Ethenetricarboxylates 17 and 19 were prepared by the reaction of diethyl ketomalonate with the corresponding (triphenylphosphoranylidne)acetate according to the literature procedure.27,30 The (triphenylphosphoranylidne)acetate esters for 17 and 19, and ylide 20 were prepared by the corresponding chloroacetates and triphenylphosphine in benezene and subsequent treatment with NaOH. The chloroacetates were preprared by the reaction of the corresponding alcohols (1 equiv) and chloroacetyl chloride (1 equiv) in the presence of pyridine (1 equiv) in Et2O at 0 °C. Data of chloroacetates for the (triphenylphosphoranylidne) acetate esters and ethenetricarboxylates 17, 19 are shown below.
(Furan-2-yl)methyl 2-chloroacetate: (30.4 mmol scale, 5.31 g, 100%); pale yellow oil; 1H NMR (400 MHz, CDCl3) δ (ppm) 4.08 (s, 2H), 5.17 (s, 2H), 6.38 (dd, J = 3.3, 2.0 Hz, 1H), 6.46 (d, J = 3.3 Hz, 1H), 7.43 (dd, J = 2.0, 0.9 Hz, 1H); 13C NMR (100.6 MHz, CDCl3) δ (ppm) 40.82 (t), 59.55 (t), 110.77 (d), 111.55 (d), 143.70 (d), 148.53 (s), 167.07 (s); IR (neat) 3126, 2958, 1760, 1501, 1411, 1369, 1309, 1284, 1167, 1079, 1017, 964 cm-1; MS (EI) m/z 176 (M+, 11), 174 (M+, 34), 98 (19), 81 (100%); HRMS (EI) M+ 174.0065, 176.0070 (calcd for C7H7ClO3 M+ 174.0083, 176.0054).
(Furan-3-yl)methyl 2-chloroacetate: (30.4 mmol scale, 5.27 g, 99%); pale yellow oil; 1H NMR (400 MHz, CDCl3) δ (ppm) 4.07 (s, 2H), 5.10 (s, 2H), 6.44 (dd, J = 1.8, 0.8 Hz, 1H), 7.41 (dd, J = 1.8, 1.8 Hz, 1H), 7.51 (ddd, J = 1.8, 0.8, 0.8 Hz, 1H); 13C NMR (100.6 MHz, CDCl3) δ (ppm) 40.92 (t), 59.39 (t), 110.63 (d), 119.62 (s), 142.15 (d), 143.67 (d), 167.28 (s); IR (neat) 3137, 2959, 1752, 1505, 1412, 1312, 1289, 1159, 1076, 1021, 956, 875 cm-1; MS (EI) m/z 176 (M+, 8.8), 174 (M+, 27), 98 (100), 81 (63%); HRMS (EI) M+ 174.0064, 176.0070 (calcd for C7H7ClO3 M+ 174.0084, 176.0054); Anal. Calcd for C7H7ClO3: C, 48.16; H, 4.04. Found: C, 48.11; H, 3.79.
(2E,4E)-Hexa-2,4-dienyl 2-chloroacetate: (15.2 mmol scale, 2.55 g, 96%); colorless oil; bp 78-80 °C/3.6 mmHg; 1H NMR (400 MHz, CDCl3) δ (ppm) 1.77 (bd, J = 6.6 Hz, 3H), 4.07 (s, 2H), 4.68 (d, J = 6.6 Hz, 2H), 5.62 (dt, J = 15.2, 6.6 Hz, 1H), 5.78 (dq, J = 15.0, 6.6 Hz, 1H), 6.06 (ddq, J = 15.0, 10.5, 1.6 Hz, 1H), 6.29 (dd, J = 15.2, 10.5 Hz, 1H); 13C NMR (100.6 MHz, CDCl3) δ (ppm) 18.16 (q), 40.93 (t), 66.69 (t), 122.48 (d), 130.23 (d), 132.10 (d), 136.08 (d), 167.11 (s); IR (neat) 3024, 2959, 1762, 1737, 1661, 1446, 1413, 1380, 1309, 1168, 990 cm-1; MS (EI) m/z 176 (M+, 13), 174 (M+, 39), 98 (37), 97 (37), 81 (79), 79 (100%); HRMS (EI) M+ 174.0431, 176.0431 (calcd for C8H11ClO2 M+ 174.0448, 176.0418).
1,1-Diethyl 2-(furan-2-yl)methyl ethene-1,1,2-tricarboxylate (17): (19.2 mmol scale, 3.85 g, 68%); Rf = 0.6 (hexane-Et2O = 1 : 1); pale yellow oil; 1H NMR (400 MHz, CDCl3) δ (ppm) 1.31 (t, J = 7.1 Hz, 3H), 1.32 (t, J = 7.1 Hz, 3H), 4.29 (q, J = 7.1 Hz, 2H), 4.31 (q, J = 7.1 Hz, 2H), 5.17 (s, 2H), 6.37 (dd, J = 3.3, 1.8 Hz, 1H), 6.45 (bd, J = 3.3 Hz, 1H), 6.88 (s, 1H), 7.44 (dd, J = 1.8, 0.9 Hz, 1H); 13C NMR (100.6 MHz, CDCl3) δ (ppm) 13.91 (q), 13.99 (q), 59.11 (t), 62.18 (t), 62.58 (t), 110.77 (d), 111.52 (d), 129.42 (d), 139.47 (s), 143.66 (d), 148.38 (s), 162.20 (s), 163.18 (s), 164.14 (s); IR (neat) 2984, 1781, 1732, 1650, 1502, 1467, 1446, 1377, 1344, 1261, 1177, 1067, 1018 cm-1; MS (EI) m/z 296 (M+, 1.3), 200 (61), 154 (81), 126 (56), 81 (100%); HRMS (EI) M+ 296.0891 (calcd for C14H16O7 M+ 296.0896).
1,1-Diethyl 2-(furan-3-yl)methyl ethene-1,1,2-tricarboxylate (19): (16.4 mmol scale, 1.25 g, 26%); Rf = 0.6 (hexane-Et2O = 1 : 1); colorless oil; 1H NMR (400 MHz, CDCl3) δ (ppm) 1.311 (t, J = 7.1 Hz, 3H), 1.314 (t, J = 7.1 Hz, 3H), 4.29 (q, J = 7.1 Hz, 2H), 4.31 (q, J = 7.1 Hz, 2H), 5.09 (s, 2H), 6.43 (dd, J = 1.8, 0.8 Hz, 1H), 6.87 (s, 1H), 7.41 (dd, J = 1.8, 1.6 Hz, 1H), 7.50 (ddd, J = 1.6, 0.8, 0.8 Hz, 1H); 13C NMR (100.6 MHz, CDCl3) δ (ppm) 13.89 (q), 13.97 (q), 58.95 (t), 62.12 (t), 62.57 (t), 110.62 (d), 119.50 (s), 129.65 (d), 139.29 (s), 142.04 (d), 143.63 (d), 162.23 (s), 163.43 (s), 164.18 (s); IR (neat) 2984, 1737, 1651, 1505, 1466, 1447, 1372, 1345, 1257, 1179, 1067, 1023 cm-1; MS (EI) m/z 296 (M+, 3.8), 199 (83), 143 (72), 126 (64), 81 (100%); HRMS (EI) M+ 296.0880 (calcd for C14H16O7 M+ 290.0896).
Diethyl 1,3,3a,7a-tetrahydro-5-methyl-3-oxoisobenzofuran-4,4(5H)-dicarboxylate (22). To an ice-water-cooled solution of (2E,4E)-hexa-2,4-dien-1-yl (triphenylphosphoranylidene)acetate (20) (2.63 g, 6.57 mmol) in 13 mL of benzene was added diethyl ketomalonate (1.0 mL, 1.14 g, 6.57 mmol). The mixture was stirred for 1 h at 0 °C and then allowed to warm to room temperature and stirred overnight. The benzene was evaporated, and Et2O was added. The precipitated triphenylphosphine oxide was removed by filtration. The filtrate was concentrated, and the residue was purified by column chromatography over silica gel with hexane-Et2O (2 : 1) as eluent to give 22 (821 mg, 42%) as an isolable product.
22: Rf = 0.1 (hexane-Et2O = 2 : 1); colorless oil; 1H NMR (400 MHz, CDCl3) δ (ppm) 1.03 (d, J = 7.3 Hz, 3H), 1.25 (t, J = 7.1 Hz, 3H), 1.30 (t, J = 7.1 Hz, 3H), 2.69-2.80 (m, 2H), 3.40 (qdd, J = 7.3, 2.6, 2.2 Hz, 1H), 3.83-3.88 (m, 1H), 4.19-4.36 (m, 4H), 4.43-4.46 (m, 1H), 5.72 (bs, 2H); 13C NMR (100.6 MHz, CDCl3) δ (ppm) 13.72 (q), 13.88 (q), 17.72 (q), 36.37 (d), 38.00 (d), 42.65 (d), 57.38 (s), 61.61 (t), 61.88 (t), 69.20 (t), 121.65 (d), 134.73 (d), 167.48 (s), 168.55 (s), 171.52 (s); 1H NMR (400 MHz, C6D6) δ (ppm) 0.860 (d, J = 7.5 Hz, 3H), 0.956 (t, J = 7.1 Hz, 3H), 0.998 (t, J = 7.1 Hz, 3H), 2.46 (m, 1H), 2.58 (d, J = 13.9 Hz, 1H), 3.04 (dd, J = 11.1, 8.0 Hz, 1H), 3.51 (m, 1H), 3.68 (dd, J = 8.0, 6.7 Hz, 1H), 3.93-4.10 (m, 4H), 5.11 (ddd, J = 9.9, 1.7, 1.7 Hz, 1H), 5.32 (ddd, J = 9.9, 3.8, 2.7 Hz, 1H). Selected NOEs are between δ 0.860 (C5-CH3) and δ 2.58 (C3a-H), 5.32 (C6-H), between δ 2.46 (C7a-H) and δ 3.68 (C1-HH), and between δ 2.58 (C3a-H) and δ 3.04 (C1-HH). Atom numbering is shown in Scheme 10.; 13C NMR (100.6 MHz, C6D6) δ (ppm) 13.83 (q), 13.99 (q), 18.01 (q), 36.89 (d), 38.25 (d), 43.22 (d), 57.92 (s), 61.61 (t), 61.82 (t), 68.68 (t), 121.99 (d), 134.85 (d), 167.93 (s), 168.81 (s), 170.95 (s). Selected HMBC correlations are between δ 3.04, 3.68 (C1-H2) and δ 121.99 (C7), 38.25 (C7a), 43.22 (C3a), between δ 2.58 (C3a-H) and δ 38.25 (C7a), 57.92 (C4), and between δ 5.11 (C7-H) and δ 43.22 (C3a), 36.89 (C5), between δ 2.58 (C3a-H), 5.32 (C6-H) and δ 36.89 (C5).; IR (neat) 2980, 1799, 1733, 1465, 1368, 1265, 1209, 1185, 1099, 1002 cm-1; MS (EI) m/z 296 (M+, 41), 251 (29), 223 (100%); HRMS (EI) M+ 296.1261 (calcd for C15H20NO6 296.1260); Anal. Calcd for C15H20O6: C, 60.80; H, 6.80. Found: C, C, 61.05; H, 7.02.
ACKNOWLEDGEMENTS
This work was supported by the Ministry of Education, Culture, Sports, Science, and Technology (MEXT), Japan and JSPS KAKENHI Grant Number 26410048. This work was partly supported by Nanotechnology Platform Program of MEXT. We thank Nara Institute of Science and Technology (NAIST) and Prof. K. Kakiuchi (NAIST) for mass spectrometry. We also thank Ms E. Hirai and Mr. K. Ueda (Nara University of Education) for experimental help.
Supporting Information available: Cartesian coordinates of the optimized geometries, Crystallographic data, 1H and 13C NMR spectral data, and 2D NOESY spectra.
References
1. (a) W. Oppolzer, In Comprehensive Organic Synthesis, ed. by B. M. Trost and I. Fleming, Pergamon, 1991, Vol. 5, 315; CrossRef (b) W. R. Roush, In Comprehensive Organic Synthesis, ed. by B. M. Trost and I. Fleming, Pergamon, 1991, Vol. 5, 513. CrossRef
2. (a) S. Yamazaki, K. Fujinami, Y. Maitoko, K. Ueda, and K. Kakiuchi, J. Org. Chem., 2013, 78, 8405; CrossRef (b) S. Yamazaki, Y. Maenaka, K. Fujinami, and Y. Mikata, RSC Adv., 2012, 2, 8095; (c) S. Yamazaki, Y. Yamamoto, Y. Fukushima, M. Takebayashi, T. Ukai, and Y. Mikata, J. Org. Chem., 2010, 75, 5216; CrossRef (d) S. Yamazaki, M. Takebayashi, and K. Miyazaki, J. Org. Chem., 2010, 75, 1188; CrossRef (e) S. Yamazaki, Y. Iwata, and Y. Fukushima, Org. Biomol. Chem., 2009, 7, 655; CrossRef (f) S. Morikawa, S. Yamazaki, M. Tsukada, S. Izuhara, T. Morimoto, and K. Kakiuchi, J. Org. Chem., 2007, 72, 6459; CrossRef (g) S. Yamazaki and Y. Iwata, J. Org. Chem., 2006, 71, 739. CrossRef
3. B. B. Snider, D. M. Roush, and T. A. Killinger, J. Am. Chem. Soc., 1979, 101, 6023. CrossRef
4. (a) H. K. Hall, P. Nogues, J. W. Rhoades, R. C. Sentman, and M. Detar, J. Org. Chem., 1982, 47, 1451; CrossRef (b) W. Zestawski, J. Barafiska, M. Jamrozik, and J. Jamrozik, Monatsh. Chem., 1997, 128, 389; CrossRef (c) E. Winterfeldt and H.-J. Dillinger, Chem. Ber., 1966, 99, 1558. CrossRef
5. (a) D. D. Sternbach and D. M. Rossana, Tetrahedron Lett., 1982, 23, 303; CrossRef (b) M. E. Jung and L. J. Street, J. Am. Chem. Soc., 1984, 106, 8327; CrossRef (c) D. D. Sternbach, D. M. Rossana, and K. D. Onan, Tetrahedron Lett., 1985, 26, 591; CrossRef (d) M. E. Jung, Synlett, 1990, 186; CrossRef (e) M. E. Jung and J. Gervay, Tetrahedron Lett., 1990, 31, 4685; CrossRef (f) M. E. Jung and J. Gervay, J. Am. Chem. Soc., 1991, 113, 224; CrossRef (g) T. Hudlicky, G. Butora, S. P. Fearnley, A. G. Gum, P. J. Persichini, III, M. R. Stabile, and J. S. Merola, J. Chem. Soc., Perkin Trans. 1, 1995, 2393; CrossRef (h) C. Giessner-Prettre, S. Hückel, J. Maddaluno, and M. E. Jung, J. Org. Chem., 1997, 62, 1439; CrossRef (i) M. E. Jung and M. Kiankarimi, J. Org. Chem., 1998, 63, 2968; CrossRef (j) A. L. Schwan, J. L. Snelgrove, M. L. Kalin, R. D. J. Froese, and K. Morokuma, Org. Lett., 1999, 1, 487; CrossRef (k) M. E. Jung and S.-J. Min, J. Am. Chem. Soc., 2005, 127, 10834; CrossRef (l) A. Padwa and Q. Wang, J. Org. Chem., 2006, 71, 3210; CrossRef (m) K. Paulvannan and J. W. Jacobs, Tetrahedron, 1999, 55, 7433; CrossRef (n) F. I. Zubkov, I. K. Airiyan, J. D. Ershova, T. R. Galeev, V. P. Zaytsev, E. V. Nikitina, and A. V. Varlamov, RSC Adv., 2012, 2, 4103; (o) C.-C. Wang and W.-D. Z. Li, J. Org. Chem., 2012, 77, 4217; CrossRef (p) C.-H. Chen, G. S. Yellol, C.-H. Tsai, P. B. Dalvi, and C.-M. Sun, J. Org. Chem., 2013, 78, 9738. CrossRef
6. (a) G. W. Huber, S. Iborra, and A. Corma, Chem. Rev., 2006, 106, 4044; CrossRef (b) A. Corma, S. Iborra, and A. Velty, Chem. Rev., 2007, 107, 2411; CrossRef (c) X. Tong, Y. Ma, and Y. Li, Appl. Catal. A: General, 2010, 385, 1; CrossRef (d) J. N. Chheda, G. W. Huber, and J. A. Dumesic, Angew. Chem. Int. Ed., 2007, 46, 7164; CrossRef (e) A. J. Ragauskas, C. K. Williams, B. H. Davison, G. Britovsek, J. Cairney, C. A. Eckert, W. J. Frederick Jr., J. P. Hallett, D. J. Leak, C. L. Liotta, J. R. Mielenz, R. Murphy, R. Templer, and T. Tschaplinski, Science, 2006, 311, 484; CrossRef (f) P. W. Lichtenthaler, Acc. Chem. Res., 2002, 35, 728; CrossRef (g) R.-J. van Putten, J. C. van der Waal, E. de Jong, C. B. Rasrendra, H. J. Heeres, and J. G. de Vries, Chem. Rev., 2013, 113, 1499. CrossRef
7. (a) H. Heany and J. S. Ahn, In Comprehensive Heterocyclic Chemistry II, ed. by A. R. Katrizky, C. W. Rees, and E. F. V. Scriven, Pergamon, Oxford, 1996, Vol. 2, p 297; CrossRef (b) B. A. Keay and P. W. Dibble, In Comprehensive Heterocyclic Chemistry II, ed. by A. R. Katrizky, C. W. Rees and E. F. V. Scriven, Pergamon, Oxford, 1996, Vol. 2, p 395; CrossRef (c) M. V. Sargent and F. M. Dean, In Comprehensive Heterocyclic Chemistry II, ed. by A. R. Katrizky and C. W. Rees, Pergamon, Oxford, 1984, Vol. 4, p 599; CrossRef (d) B. H. Lipshutz, Chem. Rev., 1986, 86, 795; CrossRef (e) H. Frey, Synlett, 1993, 905. CrossRef
8. (a) W. Zhuang, T. Hansen, and K. A. Jorgensen, Chem. Commun., 2001, 347; CrossRef (b) K. B. Jensen, J. Thorhauge, R. G. Hazell, and K. A. Jørgensen, Angew. Chem. Int. Ed., 2001, 40, 160; CrossRef (c) S. Adachi, F. Tanaka, K. Watanabe, and T. Harada, Org. Lett., 2009, 11, 5206; CrossRef (d) M. J. Stoermer, H.-M. Richter, and D. E. Kaufmann, Tetrahedron Lett., 2013, 54, 6776. CrossRef
9. S. Yamazaki, S. Kashima, T. Kuriyama, Y. Iwata, T. Morimoto, and K. Kakiuchi, Tetrahedron: Asymmetry, 2009, 20, 1224. CrossRef
10. (a) N. Katagiri, H. Akatsuka, C. Kaneko, and A. Sera, Tetrahedron Lett., 1988, 29, 5397; CrossRef (b) N. Katagiri, T. Haneda, E. Hayasaka, N. Watanabe, and C. Kaneko, J. Org. Chem., 1988, 53, 226; CrossRef (c) M. M. Walker, C. G. Goodman, and J. S. Johnson, J. Org. Chem., 2014, 79, 9385; CrossRef (d) J. N. Marx and E, J. Bombach, Tetrahedron Lett., 1977, 2391; CrossRef (e) G. B. Bachman and H. A. Tanner, J. Org. Chem., 1939, 4, 493. CrossRef
11. R. K. Boeckman Jr. and S. S. Ko, J. Am. Chem. Soc., 1982, 104, 1033. CrossRef
12. (a) K. N. Houk and L. J. Luskus, J. Am. Chem. Soc., 1971, 93, 4606; CrossRef (b) J. Furukawa, Y. Kobuke, and T. Fueno, J. Am. Chem. Soc., 1970, 92, 6548; CrossRef (c) K. L. Williamson and Y.-F. L. Hsu, J. Am. Chem. Soc., 1970, 92, 7385. CrossRef
13. (a) A. D. Becke, J. Chem. Phys., 1993, 98, 5648; CrossRef (b) C. Lee, W. Yang, and R. G. Parr, Phys. Rev. B, 1998, 37, 785. CrossRef
14. Gaussian 09, Revision D.01, M. J. Frisch, G. W. Trucks, H. B. Schlegel, G. E. Scuseria, M. A. Robb, J. R. Cheeseman, G. Scalmani, V. Barone, B. Mennucci, G. A. Petersson, H. Nakatsuji, M. Caricato, X. Li, H. P. Hratchian, A. F. Izmaylov, J. Bloino, G. Zheng, J. L. Sonnenberg, M. Hada, M. Ehara, K. Toyota, R. Fukuda, J. Hasegawa, M. Ishida, T. Nakajima, Y. Honda, O. Kitao, H. Nakai, T. Vreven, J. A. Montgomery, Jr., J. E. Peralta, F. Ogliaro, M. Bearpark, J. J. Heyd, E. Brothers, K. N. Kudin, V. N. Staroverov, R. Kobayashi, J. Normand, K. Raghavachari, A. Rendell, J. C. Burant, S. S. Iyengar, J. Tomasi, M. Cossi, N. Rega, J. M. Millam, M. Klene, J. E. Knox, J. B. Cross, V. Bakken, C. Adamo, J. Jaramillo, R. Gomperts, R. E. Stratmann, O. Yazyev, A. J. Austin, R. Cammi, C. Pomelli, J. W. Ochterski, R. L. Martin, K. Morokuma, V. G. Zakrzewski, G. A. Voth, P. Salvador, J. J. Dannenberg, S. Dapprich, A. D. Daniels, Ö. Farkas, J. B. Foresman, J. V. Ortiz, J. Cioslowski, and D. J. Fox, Gaussian, Inc., Wallingford CT, 2009.
15. (a) E. Cancès, B. Mennucci, and J. Tomasi, J. Chem. Phys., 1997, 107, 3032; CrossRef (b) M. Cossi, V. Barone, B. Mennucci, and J. Tomasi, Chem. Phys. Lett., 1998, 286, 253; CrossRef (c) B. Mennucci and J. Tomasi, J. Chem. Phys., 1997, 106, 5151. CrossRef
16. (a) K. Fukui, J. Phys. Chem., 1970, 74, 4161; CrossRef (b) C. Gonzalez and H. B. Schlegel, J. Phys. Chem., 1989, 90, 2154. CrossRef
17. Using AlCl3 as a model of EtAlCl2, five-coordinated bidentate complexes were calculated. Although Al Lewis acids have a tendency to form stable four-coordinated complexes, the model could represent bidentate Lewis acid coordination to 1m. In the real system, one Cl- may bind with another EtAlCl2 and form EtAlCl3-. Lewis acids such as Zn(OTf)2 and Cu(OTf)2 may form stable two carbonyl coordinated chelate intermediates.
18. D. A. Evans, T. Rovis, M. C. Kozlowski, C. W. Downey, and J. S. Tedrow, J. Am. Chem. Soc., 2000, 122, 9134. CrossRef
19. (a) K. N. Houk, J. Gonzalez, and Y. Li, Acc. Chem. Res., 1995, 28, 81; CrossRef (b) L. R. Domingo, M. T. Picher, and M. J. Aurell, J. Phys. Chem. A, 1999, 103, 11425; CrossRef (c) L. R. Domingo, M. J. Aurell, P. Pérez, and R. Contreras, J. Org. Chem., 2003, 68, 3884; CrossRef (d) M. Linder and T. Brinck, J. Org. Chem., 2012, 77, 6563; CrossRef (e) L. R. Domingo and J. A. Sáez, Org. Biomol. Chem., 2009, 7, 3576. CrossRef
20. Crystallographic data for 9a have been deposited with the Cambridge Crystallographic Data Centre (no. CCDC 1419386). Interestingly, obtained single crystals from the racemic product 9a are chiral ones. Flack parameter is 0.1(7). Resolution of racemic 9a may be possible using the single crystals.
21. S. Duan, R. Jana, and J. A. Tunge, J. Org. Chem., 2009, 74, 4612. CrossRef
22. The byproducts by the reaction of CH2ClCH2Cl and HOBt in the presence of Et3N was formed and removed by column chromatography. (a) J.-G. Ji, D.-Y. Zhang, Y.-H. Ye, and Q.-Y. Xing, Tetrahedron Lett., 1998, 39, 6515; CrossRef (b) W. A. Feld and D. G. Evans, J. Chem. Eng. Data, 1983, 28, 138. CrossRef
23. A. Volonterio, C. R. de Arellano, and M. Zanda, J. Org. Chem., 2005, 70, 2161. CrossRef
24. (a) L. R. Domingo, M. T. Picher, and R. J. Zaragozá, J. Org. Chem., 1998, 63, 9183; CrossRef (b) L. R. Domingo, P. Pérez, and D. E. Ortega, J. Org. Chem., 2013, 78, 2462. CrossRef
25. (a) S. Yamazaki, S. Morikawa, Y. Iwata, M. Yamamoto, and K. Kuramoto, Org. Biomol. Chem., 2004, 2, 3134; CrossRef (b) S. Yamazaki, M. Yamamoto, and S. Morikawa, Heterocycles, 2006, 67, 269. CrossRef
26. (a) Y. Fukushima, S. Yamazaki, and A. Ogawa, Org. Biomol. Chem., 2014, 12, 3964; CrossRef (b) S. Yamazaki, J. Wada, and K. Kakiuchi, Can. J. Chem., 2015, 93, 1122. CrossRef
27. S. Yamazaki, K. Ohmitsu, K. Ohi, T. Otsubo, and K. Moriyama, Org. Lett., 2005, 7, 759. CrossRef
28. H.-P. Wu, R. Aumann, R. Fröhlich, and E. Wegelius, Organometallics, 2001, 20, 2183. CrossRef
29. (a) H. Anan, A. Tanaka, R. Tsuzuki, M. Yokota, T. Yatsu, and T. Fujikura, Chem. Pharm. Bull., 1996, 44, 1865; CrossRef (b) R. Murali, H. S. P. Rao, and H. W Scheeren, Tetrahedron, 2001, 57, 3165. CrossRef
30. S. Yamazaki and M. Takebayashi, J. Org. Chem., 2011, 76, 6432. CrossRef