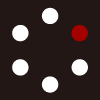
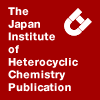
HETEROCYCLES
An International Journal for Reviews and Communications in Heterocyclic ChemistryWeb Edition ISSN: 1881-0942
Published online by The Japan Institute of Heterocyclic Chemistry
e-Journal
Full Text HTML
Received, 18th January, 2016, Accepted, 29th January, 2016, Published online, 1st February, 2016.
DOI: 10.3987/COM-16-13408
■ Synthesis of a New Chiral C2-Symmetric NHC-AuCl Complex
Naoya Okitsu, Takuya Yoshida, Kensuke Usui, and Masahisa Nakada*
Department of Chemistry and Biochemistry, School of Advanced Science and Engineering, Waseda University, 3-4-1 Okubo, Shinjuku-ku, Tokyo 169-8555, Japan
Abstract
A New chiral C2 symmetric NHC ligand with two binaphthyl units has been synthesized. The new NHC ligand features two seven-membered rings that link the imidazolylidene with the binaphthyl units. X-Ray crystallographic analysis of the new NHC-AuCl complex indicates that the Au-Cl bond is located between the phenyl groups of the binaphthyl units and suggests that this arrangement could induce enantioselectivity in reactions. Indeed, catalytic asymmetric ene-yne cyclization of 2b in the presence of a catalytic amount (5 mol%) of cationic complex of 13 quantitatively afforded the cyclized product with 78% ee.N-Heterocyclic carbenes (NHCs) were first isolated as stable crystals in 1991 by Arduengo and co-workers,1 but metal complexes of NHCs are known since the independent works of Öfele2 and Wanzlick3 in 1968. NHCs have attracted attention because of their unique structures and properties, which result from the strong coordination with late-transition metals owing to their strong -donor and weak -accepter characteristics.4 NHCs have been studied as a new class of ligands in organometallic chemistry and have been developed as privileged ligands in terms of their coordinating properties and stability. They have been used as organocatalysts,5 and their use is expected to increase in future.
Chiral ligands play a pivotal role in asymmetric catalysis, and a number of chiral phosphine ligands have been developed to achieve high enantioselectivity.6 While chiral phosphine7 and phosphoramidite ligands,8 and chiral counter ions,9 have been developed to achieve high enantioselectivity in Au(I)-catalyzed reactions, very few successful examples of chiral NHC-Au(I) have been documented.10 This is attributed to the linear geometry of Au(I) complexes, which naturally places the captured substrate trans to the chiral ligand. We have been studying the design and synthesis of chiral NHC ligands,11 and report herein a new chiral C2-symmetric NHC ligand-Au(I) complex that catalyzes the enantioselective cyclization of a 1,6-ene-yne with high % ee.
Recently, we reported new NHC ligands 1 with chiral binaphthyl units linked at the C4 and C5 positions by a carbocyclic eight-membered ring (Figure 1).11c A cationic Au(I) complex of 1 (R = iPrPh2C-) showed catalytic activity toward the enantioselective cyclization of 1,6-ene-yne 2a to afford the product 3a (98%, 44% ee, Scheme 1). The marginal enantioselectivity was attributed to unhindered (or free) rotation of the substituents (R) that were located at a sterically less hindered position to avoid steric repulsion from the phenyl groups at C3 and C3′. The enantioselectivity depends on the steric bulkiness of these substituents, and free rotation about the carbon-nitrogen bond allows them to move to positions less conducive for enantioselective discrimination.
To overcome the limitations due to the stability and rigid structure of NHC ligands possessing fixed nitrogen substituents, we designed a new chiral C2-symmetric NHC ligand using rigid nitrogen substituents 4 (Figure 2). This ligand features two chiral binaphthyl units that are bound to either side of the imidazolylidene moiety as part of the seven-membered rings. Although the phenyl groups on the binaphthyl groups rotate about the carbon-carbon bond, their fixed orientation would be expected to induce good enantioselectivity in the ene-yne cyclization.
Scheme 2 shows our retrosynthetic analysis of imidazolium salt 5, which would be the precursor of the new chiral ligand 4. Based on the previously reported procedure, diimine 6 was selected as the precursor of 5. Diimine 6 could be prepared by the intramolecular reaction of the corresponding diamino-diketone formed by the removal of the protective group on nitrogen in diketone 7. Diketone 7 could be prepared by the pinacol coupling of aldehyde 8, which itself could be prepared from 9, a derivative of (S)-BINOL reported by Page and co-workers.12
The synthesis of the new chiral C2-symmetric imidazolium salt 5 is summarized in Scheme 3. The synthesis commenced with the benzylic bromination of 9 with a slight excess of NBS (4 equiv), in the presence of AIBN in refluxing benzene, to afford 10 quantitatively. The sterically hindered methyl group (adjacent to the phenyl group) was mono-brominated, and the less-hindered methyl group was di-brominated. The two methyl groups were thus successfully converted to different oxidation levels. Treatment of compound 10 with formic acid under reflux afforded aldehyde 11 in 85% yield. Aldehyde 11 was converted to the corresponding ethylene acetal (94% yield), which was reacted with sodium azide to generate azide 12 (quantitative yield). Azide 12 was subjected to Staudinger reaction to afford the corresponding amine. Subsequent reaction of this amine with allyl chloroformate and hydrolysis of the ethylene acetal under acidic conditions afforded aldehyde 8, the key intermediate in Scheme 2. The reaction of 8 with a low-valent titanium reagent which was in situ generated by Cp2ZrCl2 and Zn lead to pinacol coupling,13 producing a mixture of diastereomeric 1,2-diols. Although Swern oxidation of the 1,2-diols was not reproducible, oxidation using DMSO and TFAA successfully afforded 1,2-diketone 7. Following the removal of the Alloc groups from 7,14 the obtained diamino-diketone underwent spontaneous cyclization to diimine 6 (84%, three steps). Treatment of 6 with MOMCl in THF at 50 °C produced the desired chiral C2-symmetric imidazolium salt 5 in 87% yield. Compound 5 was stable, and was purified by silica gel column chromatography.
Recrystallization of NHC-AuCl complex 13 from benzene-hexane gave a single crystal suitable for X-ray crystallographic analysis.15 Figure 3 shows the X-ray crystal structure of 13. The seven-membered rings lack flexibility because they include four atoms of the binaphthyl unit, and two of the imidazolylidene group. Although the two phenyl groups can rotate around the carbon-carbon bonds attaching them to the binaphthyl units, their axial direction is constrained due to the rigid conformation of the seven-membered rings. Moreover, the side view (b) of the crystal structure of 13 shows that the two phenyl groups are located near the Au-Cl bond. Reactions catalyzed by the Au(I) complex 13 would therefore be expected to experience an asymmetric steric interaction between the phenyl groups and the substrate, and display enantioselectivity.
The catalytic asymmetric ene-yne cyclization of 2a in the presence of 5 mol% of cationic complex of 13 afforded 3a with 33% ee in 40% yield (Scheme 4). When compared with the reaction of 2a with cationic 1 (R = iPrPh2C-)-Au(I) complex, the ee and yield decreased. However, the reaction of less-bulky substrate 2b with a catalytic amount of cationic 13 quantitatively afforded 3b with 78% ee, which surpassed those of the reaction of 2b using cationic 1 (R = iPrPh2C-)-Au(I) complex (95% yield and 34% ee). Therefore, further structural modification of 13, selection of the substrate and optimization of the reaction conditions could improve the ee and yield of the product.
In summary, a new chiral C2-symmetric NHC ligand with two binaphthyl units has been synthesized. The new NHC ligand features two seven-membered rings that link the imidazolylidene with the binaphthyl units. X-Ray crystallographic analysis of the new NHC-AuCl complex indicates that the Au-Cl bond is located between the phenyl groups of the binaphthyl units and suggests that this arrangement could induce enantioselectivity in the catalytic reactions. Indeed, although the catalytic asymmetric ene-yne cyclization of 2a in the presence of 5 mol% of cationic complex of 13 afforded 3a with 33% ee in 40% yield, the reaction of less-bulky substrate 2b quantitatively afforded 3b with 78% ee. Further modifications of the ligand and asymmetric catalysis utilizing the cationic complex of 13 and its derivatives will be reported in due course.
EXPERIMENTAL
1H and 13C NMR spectra were recorded on a JEOL AL-400 spectrometer. Chemical shifts are reported in ppm with the residual solvent resonance as internal standard (CDCl3 1H, δ = 7.26 ppm, 13C, δ = 77.16 ppm; (CD3)2SO 1H, δ = 2.50 ppm, 13C, δ = 39.52 ppm). The following abbreviations were used to explain the multiplicities: s, singlet; d, doublet; t, triplet; q, quartet; m, multiplet; band, several overlapping signals; br, broad. IR spectra were recorded on a JASCO FT/IR-8300. Optical rotations were measured using a 2 mL cell with a 1 dm path length on a JASCO DIP-1000. Mass spectra and elemental analyses were provided at the Materials Characterization Central Laboratory, Waseda University. All reactions were carried out under an argon atmosphere with dry, freshly distilled solvents under anhydrous conditions, unless otherwise noted. Melting point (mp) is uncorrected and recorded on a Yamato capillary melting point apparatus. Chiral HPLC analysis was performed on a JASCO PU-980 and UV-970 detector. X-Ray crystallographic analysis was performed with a Rigaku R-AXIS RAPID-II. All reactions were monitored by thin-layer chromatography carried out on 0.25 mm E. Merck silica gel plates (60F-254) using UV light as visualizing agent and phosphomolybdic acid and heat as developing agents. Kanto Chemical Silica Gel 60N (spherical, neutral, 63-210 μm or 40-50 μm partial size) was used for flash chromatography. Preparative thin-layer chromatography (PTLC) separations were carried out on self-made 0.3 mm E. Merck silica gel plates (60F-254). In general, reactions were carried out in dry solvents under an argon atmosphere. Unless otherwise noted, materials obtained from commercial suppliers were used without further purification. Dichloromethane (CH2Cl2) and N,N-dimethylformamide (DMF) was distilled from calcium hydride. Tetrahydrofuran (THF) was distilled from sodium/benzophenone ketyl. Toluene was distilled from sodium.
(S)-2-Bromomethyl-2’-dibromomethyl-3-phenyl-1,1’-binaphthyl (10). A stirred suspension of (S)-3-phenyl-2,2’-dimethyl-1,1’-binaphthyl 9 (3.40 g, 9.48 mmol), NBS (5.91 g, 33.2 mmol), and AIBN (156 mg, 0.95 mmol) in benzene (32 mL) was refluxed overnight. To the reaction mixture was added Et2O (20 mL) and water (20 mL), and the aqueous layer was extracted with Et2O (15 mL × 2). Combined organic layers were washed with brine (15 mL), dried over Na2SO4, and concentrated under reduced pressure. The residue was purified by silica gel column chromatography (hexane/EtOAc = 20/1) to afford 10 (5.64 g, quant) as a white solid: Rf = 0.49 (hexane/EtOH = 10/1); 1H NMR (400 MHz, CDCl3): δ 8.23 (d, J = 8.7 Hz, 1H), 8.13 (d, J = 8.7 Hz, 1H), 7.96–7.90 (m, 3H), 7.64–7.59 (m, 2H), 7.55–7.43 (m, 5H), 7.35–7.27 (m, 2H),7.16 (d, J = 8.7 Hz, 1H), 7.01 (d, J = 8.2 Hz, 1H), 6.29 (s, 1H), 4.33 (d, J = 10.3 Hz, 1H), 4.14 (d, J = 10.3 Hz, 1H); 13C NMR (100 MHz, CDCl3): δ 141.1, 140.3, 137.3, 134.3, 133.9, 133.2, 133.1, 131.8, 131.3, 131.0, 130.5, 130.3, 129.7, 128.4, 128.2, 128.1, 127.8, 127.7, 127.6, 127.1, 127.0, 126.3, 40.2, 30.4; IR (ATR): 3057.6, 1493.7, 1220.2, 902.2, 747.3, 701.4, 590.5 cm-1; HRMS (DART) [M–Br–] calcd for C28H19Br2: 512.9848, found 512.9838; mp 208 °C; [α]D24 –59 (c 0.40, CHCl3).
(S)-2-Bromomethyl-2’-oxomethyl-3-phenyl-1,1’-binaphthyl (11). A solution of 9 (894 mg, 1.50 mmol) in formic acid (15 mL) was refluxed for 6.5 h. To the reaction mixture were added CH2Cl2 (10 mL) and water (10 mL), and the aqueous layer was extracted with CH2Cl2 (15 mL × 2). Combined organic layers were dried over Na2SO4 and concentrated under reduced pressure. The residue was purified by silica gel column chromatography (CH2Cl2/EtOAc = 100/1 to 75/1) to afford 11 (579 mg, 85%) as an yellow solid: Rf = 0.29 (hexane/EtOAc = 10/1); 1H NMR (400 MHz, CDCl3): δ 9.65 (s, 1H), 8.24 (d, J = 8.7 Hz, 1H), 8.11 (d, J = 8.7 Hz, 1H), 8.01 (d, J = 8.2 Hz, 1H), 7.95 (s, 1H), 7.93 (d, J = 8.2 Hz, 1H), 7.66–7.60 (m, 3H), 7.55–7.43 (m, 4H), 7.40–7.34 (m, 1H), 7.31–7.27 (m, 2H), 7.02 (d, J = 8.2 Hz, 1H), 4.30 (d, J = 9.8 Hz, 1H), 3.99 (d, J = 9.8 Hz, 1H); 13C NMR (100 MHz, CDCl3): δ 192.2, 141.8, 140.7, 140.1, 136.5, 134.4, 133.2, 132.9, 132.8, 132.5, 130.8, 129.7, 129.5, 129.2, 128.7, 128.4, 128.2, 127.9, 127.5, 127.5, 127.4, 126.8, 126.5, 122.5, 30.7; IR (ATR): 3055.3, 2843.3, 1678.2, 1225.1, 788.8, 750.4, 698.0, 586.2 cm-1; HRMS (ESI) [M+Na+] calcd for C28H19BrONa: 473.0511, found 473.0513; mp 218 °C; [α]D24 –125 (c 0.40, CHCl3).
(S)-2-Bromomethyl-2’-(1,3-dioxolanyl)-3-phenyl-1,1’-binaphthyl (11a). A solution of 11 (1.20 g, 2.65 mmol), ethylene glycol (0.89 mL, 16.0 mmol), and TsOH·H2O (50.4 mg, 0.27 mmol) in toluene (27 mL) was refluxed using Dean-Stark apparatus for 2.5 h. Then, ethylene glycol (0.30 mL, 5.32 mmol) was added to the reaction mixture and reflux was continued for 2 h. To the reaction mixture was added saturated aqueous NaHCO3 (20 mL) and Et2O (15 mL), and the aqueous layer was extracted with Et2O (15 mL × 2). Combined organic layers were washed with brine (15 mL), dried over Na2SO4, and concentrated under reduced pressure. The residue was purified by silica gel column chromatography (hexane/EtOAc = 50/1 to 30/1) to afford 11a (1.23 g, 94%) as a white solid: Rf = 0.41 (hexane/EtOAc = 4/1); 1H NMR (400 MHz, CDCl3): δ 8.07 (d, J = 8.7 Hz, 1H), 7.94 (d, J = 8.2 Hz, 1H), 7.91–7.86 (m, 3H), 7.64–7.60 (m, 2H), 7.52–7.42 (m, 5H), 7.31–7.22 (m, 2H), 7.14 (d, J = 7.8 Hz, 1H), 7.08 (d, J = 9.2 Hz, 1H), 5.38 (s, 1H), 4.32 (d, J = 10.1 Hz, 1H), 4.25 (d, J = 10.1 Hz, 1H), 4.15–4.07 (m, 2H), 3.87–3.74 (m, 2H); 13C NMR (125 MHz, CDCl3): δ 140.7, 140.7, 136.1, 135.0, 134.3, 134.2, 133.0, 132.8, 132.7, 132.5, 130.2, 129.7, 129.2, 128.3, 128.1, 127.8, 127.6, 127.4, 127.3, 127.1, 126.8, 126.8, 126.3, 123.7, 101.9, 65.6, 31.1; IR (ATR): 3058.9, 2889.1, 1213.4, 1062.6, 942.0, 813.4, 754.6, 723.5 cm-1; HRMS (ESI) [M+Na+] calcd for C30H23BrO2Na: 517.0774, found 517.0776; mp 204 °C; [α]D24 –58 (c 0.40, CHCl3).
(S)-2-Azidomethyl-2’-(1,3-dioxolanyl)-3-phenyl-1,1’-binaphthyl (12). A suspension of 11a (643 mg, 1.30 mmol) and NaN3 (211 mg, 3.24 mmol) in DMF (13 mL) was heated at 60 °C overnight. To the reaction mixture was added Et2O (10 mL) and water (10 mL), and the aqueous layer was extracted with Et2O (5 mL × 2). Combined organic layers were washed with brine (5 mL), dried over Na2SO4, and concentrated under reduced pressure. The residue was purified by silica gel column chromatography (hexane/EtOAc = 40/1 to 30/1) to afford 11 (592 mg, 100%) as a white solid: Rf = 0.41 (hexane/EtOAc = 4/1); 1H NMR (400 MHz, CDCl3): δ 8.07 (d, J = 8.7 Hz, 1H), 7.97–7.86 (m, 4H), 7.56–7.47 (m, 6H), 7.46–7.40 (m, 1H), 7.33–7.27 (m, 2H), 7.14 (d, J = 8.9 Hz, 1H), 7.11 (d, J = 8.7 Hz, 1H), 5.36 (s, 1H), 4.20–4.07 (m, 4H), 3.85–3.76 (m, 2H); 13C NMR (125 MHz, CDCl3): δ 140.9, 140.7, 136.4, 135.2, 134.3, 134.1, 132.8, 132.8, 132.6, 131.5, 129.9, 129.6, 129.2, 128.4, 128.2, 127.9, 127.6, 127.1, 127.0, 126.8, 126.8, 126.7, 123.7, 101.9, 65.7, 50.8; IR (ATR): 3057.2, 2890.1, 2074.7, 1240.6, 1098.8, 817.1, 748.1, 701.6 cm-1; HRMS (ESI) [M+Na+] calcd for C30H23N3O2Na: 480.1682, found 480.1684; mp 76 °C [α]D24 –87 (c 0.33, CHCl3).
(S)-2-(N-Allyloxycarbonylaminomethyl)-2'-(1,3-dioxolanyl)-3-phenyl-1,1'-binaphthyl (12a). A suspension of 11 (927 mg, 2.03 mmol) and PPh3 (797 mg, 3.04 mmol) in THF (20 mL) and water (2 mL) was heated at 50 °C overnight. The reaction mixture was concentrated under reduced pressure and a phosphine oxide was removed by short silica gel column chromatography (CH2Cl2 to CH2Cl2/MeOH = 20/1) to afford a crude amine. To the crude amine dissolved in THF (20 mL) were added Et3N (0.56 mL, 4.05 mmol) and AllocCl (0.29 mL, 2.43 mmol) at room temperature. After stirring at room temperature for 15 min, to the reaction mixture was added water (15 mL) and the aqueous layer was extracted with Et2O (10 mL × 2). Combined organic layers were washed with brine (10 mL), dried over Na2SO4, and concentrated under reduced pressure. The residue was purified by silica gel column chromatography (hexane/EtOAc = 10/1 to 5/1) to afford 12a (956 mg, 91%) as a white solid: Rf = 0.36 (hexane/EtOAc = 2/1); 1H NMR (400 MHz, CDCl3): δ 8.03 (d, J = 8.7 Hz, 1H), 7.96–7.84 (m, 4H), 7.53–7.26 (m, 9H), 7.12 (d, J = 8.2 Hz, 2H), 5.83–5.45 (m, 2H), 5.41 (s, 1H), 5.20–4.88 (m, 2H), 4.34–3.58 (m, 8H); 13C NMR (100 MHz, CDCl3): δ 155.1, 141.6, 140.9, 135.7, 135.5, 134.1, 134.0, 133.9, 133.5, 132.7, 132.6, 132.2, 130.0, 129.5, 128.9, 128.3, 128.2, 127.9, 127.5, 127.0, 126.9, 126.9, 126.8, 126.4, 126.1, 123.5, 116.5, 101.8, 66.0, 65.2, 64.9, 41.0; IR (ATR): 3306.7, 3060.3, 2884.9, 1681.7, 1541.1, 1249.2, 1097.8, 703.7 cm-1; HRMS (ESI) [M+Na+] calcd for C34H29NO4Na: 538.1989, found 538.1989; mp 108 °C; [α]D24 –168 (c 0.33, CHCl3).
(S)-2-(N-Allyloxycarbonylaminomethyl)-2'-oxomethyl-3-phenyl-1,1'-binaphthyl (8). A suspension of 12a (1.78 g, 3.46 mmol) and TsOH·H2O (328 mg, 1.73 mmol) in acetone/water (35mL/2.3mL) was stirred at room temperature for 4 h. To the reaction mixture was added saturated aqueous NaHCO3 (20 mL) and Et2O (20 mL), and the aqueous layer was extracted with Et2O (15 mL × 2). Combined organic layers were washed with brine (15 mL), dried over Na2SO4, and concentrated under reduced pressure. The residue was purified by recrystallization (CH2Cl2/EtOAc) to afford 8 (1.43g, 88%) as a white solid: Rf = 0.49 (hexane/EtOAc = 2/1); 1H NMR (400 MHz, CDCl3): δ 9.68 (s, 1H), 8.18 (d, J = 8.7 Hz, 1H), 8.08 (d, J = 8.7 Hz, 1H), 7.99 (d, J = 8.2 Hz, 1H), 7.94 (s, 1H), 7.93 (d, J = 10.5 Hz, 1H), 7.62 (dd, J = 7.3, 7.3 Hz, 1H), 7.54–7.27 (m, 9H), 7.04 (d, J = 8.2 Hz, 1H), 5.66 (br, 1H), 5.13–5.07 (m, 2H), 4.23–3.92 (m, 5H); 13C NMR (100 MHz, CDCl3): δ 192.0, 154.7, 142.7, 140.7, 140.6, 136.4, 133.1, 133.0, 132.9, 132.5, 132.4, 130.5, 129.3, 129.1, 128.7, 128.7, 128.1, 127.8, 127.2, 127.0, 126.9, 126.6, 122.6, 117.5, 65.4, 41.2; IR (ATR): 3304.3, 3060.7, 1682.4, 1540.8, 1254.3, 749.9, 700.8, 652.6 cm-1; HRMS (ESI) [M+Na+] calcd for C32H25NO3Na: 494.1727, found 494.1727; mp 180 °C; [α]D24 –40 (c 0.33, CHCl3).
(S,S)-1,2-Bis(2'-N-allyloxycarbonylaminomethyl-3'-phenyl-1,1'-binaphthyl-2-yl)ethan-1,2-dione (7). To a stirred suspension of Cp2TiCl2 (323 mg, 1.30 mmol), activated zinc dust (255 mg, 3.89 mmol) and ZnCl2 (1 M solution in Et2O, 0.66 mL, 0.66 mmol) in THF (4 mL) was added a solution of 8 (583 mg, 1.24 mmol) in THF (8 mL) at –78 °C. After stirring the reaction mixture at 0 °C for 30 min, to the mixture was added CH2Cl2 (20 mL) and saturated aqueous NaHCO3 (10 mL), and the aqueous layer was extracted with CH2Cl2 (20 mL × 2). Combined organic layers were dried over Na2SO4 and concentrated under reduced pressure. The residue was purified by silica gel column chromatography (hexane/EtOAc = 8/1 to 6/1 to 2/1) to afford a mixture of 1,2-diol diastereomers. To a solution of TFAA (1.02 mL, 7.42 mmol) and DMSO (0.70 mL, 9.89 mmol) in CH2Cl2 (4 mL) was added a solution of the mixture of 1,2-diol diastereomers in CH2Cl2 (8 mL) at –60 °C. After stirring the reaction mixture at –60 °C for 1.5 h, to the mixture was added Et3N (1.38 mL, 9.89 mmol) at –60 °C and 1 M-HCl (10 mL) after warming the mixture to room temperature. The aqueous layer was extracted with CH2Cl2 (5 mL × 2) and combined organic layers were dried over Na2SO4 and concentrated under reduced pressure. The residue was purified by silica gel column chromatography (hexane/EtOAc = 10/1 to 4/1) to afford 6 with inseparable impurities as an yellow solid. Pure 7 was obtained by preparative TLC to collect the spectroscopic data: Rf = 0.28 (hexane/EtOAc = 2/1); 1H NMR (400 MHz, CDCl3): 7.92–7.81 (m, 6H), 7.76–7.62 (m, 2H), 7.57–7.08 (m, 20H), 7.03–6.92 (m, 2H), 6.78 (d, J = 8.7 Hz, 2H), 5.69 (br, 2H), 5.50–4.77 (m, 6H), 4.26–3.68 (m, 8H); 13C NMR (125 MHz, CDCl3): δ 195.6, 155.0, 141.1, 140.9, 139.2, 135.4, 135.3, 133.4, 133.3, 132.9, 132.9, 131.9, 131.1, 130.2, 129.4, 129.0, 128.5, 128.3, 128.2, 128.1, 127.6, 127.5, 127.4, 126.6, 126.4, 126.0, 125.7, 117.1, 65.2, 41.7; IR (ATR): 3342.6, 3055.3, 1712.2, 1494.0, 1218.3, 1027.8, 746.2, 701.4 cm-1; HRMS (ESI) [M+Na+] calcd for C64H48N2O6Na: 963.3405, found 963.3402; mp 108 °C; [α]D24 –15 (c 0.25, CHCl3).
(S,S)- 6,6'-Diphenyl-5H,5'H-3,3'-bidinaphtho[2,1-c:1',2'-e]azepine (6). To a stirred solution of crude 7 (568 mg, 0.60 mmol) and Pd(PPh3)4 (34.9 mg, 0.030 mmol) in CH2Cl2 (12 mL) was added Me2NSiMe3 (0.29 mL, 1.81 mmol) and AcOSiMe3 (0.27 mL, 1.81 mmol) at room temperature. After stirring the reaction mixture for 6 h, the solution was concentrated under reduced pressure. The residue was purified by silica gel column chromatography (hexane/EtOAc = 30/1 to 15/1 to 8/1) to afford 6 (374.3 mg, 84% for 3 steps from aldehyde 8) as a white solid: Rf = 0.57 (hexane/EtOAc = 2/1); 1H NMR (400 MHz, CDCl3): δ 8.00 (s, 2H), 7.99 (d, J = 8.7 Hz, 2H), 7.76 (br, 4H), 7.68 (d, J = 7.8 Hz, 2H), 7.53 (d, J = 6.9 Hz, 4H), 7.49 (d, J = 8.2 Hz, 4H), 7.44 (d, J = 7.3 Hz, 2H), 7.40 (d, J = 6.9 Hz, 2H), 7.28–7.10 (m, 8H), 6.51 (d, J = 8.2 Hz, 2H), 5.33 (d, J = 10.1 Hz, 2H), 3.79 (d, J = 10.1 Hz, 2H); 13C NMR (125 MHz, CDCl3): δ 168.0, 140.9, 140.8, 139.0, 137.4, 133.4, 132.9, 132.5, 131.9, 131.8, 131.4, 130.2, 129.9, 128.9, 128.5, 128.4, 128.1, 127.9, 127.6, 127.4, 127.0, 126.3, 125.9, 125.8, 123.9, 52.9; IR (ATR): 3054.1, 2855.7, 1586.0, 1492.5, 1206.7, 1044.8, 807.8, 749.8, 703.4 cm-1; HRMS (ESI) [M+H+] calcd for C56H37N2: 737.2951, found 737.2947; mp 233 °C; [α]D24 +695 (c 0.33, CHCl3).
(S,S)-Imidazolium salt 5. To a stirred solution of 6 (810 mg, 1.10 mmol) in THF (22 mL) was added MOMCl (0.83 mL, 11.0 mmol) at room temperature. After stirring the reaction mixture at 50 °C overnight, the solution was concentrated under reduced pressure. The residue was purified by silica gel column chromatography (CH2Cl2 to CH2Cl2/MeOH = 20/1) to afford 5 (755 mg, 87%) as a white solid: Rf = 0.34 (CH2Cl2/MeOH = 10/1); 1H NMR (400 MHz, DMSO-d6): δ 8.98 (s, 1H), 8.18–8.07 (m, 8H), 7.69–7.53 (m, 14H), 7.44–7.35 (m, 6H), 7.18 (d, J = 6.0 Hz, 2H), 7.16 (d, J = 6.0 Hz, 2H), 5.55 (d, J = 14.7 Hz, 2H), 5.07 (d, J = 14.7 Hz, 2H); 13C NMR (100 MHz, CDCl3): δ 139.3, 138.8, 135.1, 134.0, 133.6, 133.6, 133.2, 132.8, 132.3, 130.9, 130.2, 129.9, 129.7, 129.7, 128.6, 128.5, 128.4, 127.9, 127.3, 127.3, 127.1, 126.9, 126.4, 124.2, 47.9; IR (ATR): 3307.0, 3052.0, 1589.6, 1493.1, 1446.4, 1179.5, 819.6, 749.7, 703.9 cm-1; HRMS (ESI) [M–Cl–] calcd for C57H37N2: 749.2951, found 749.2946; mp 307 °C (decomposition); [α]D24 +394 (c 0.33, CHCl3).
(S,S)-NHC-Au(I) complex 13. To a mixture of 5 (158 mg, 0.20 mmol) and Ag2O (46.5 mg, 0.20 mmol) was added CH2Cl2 (4 mL). After stirring the reaction mixture in the absence of light at room temperature overnight, the resulting mixture was filtered through a pad of Celite and concentrated under reduced pressure. To a solution of the crude NHC-silver (I) complex in CH2Cl2 (4 mL) was added AuCl·SMe2 (59.3 mg 0.20 mmol). After stirring the reaction mixture at room temperature for 4.5 h, the mixture was filtered through a pad of Celite and concentrated at reduced pressure. The residue was purified by silica gel column chromatography (CH2Cl2) to afford the corresponding NHC-Au(I) complex 13 (149 mg, 76% (2 steps)) as a yellowish to white solid: Rf = 0.59 (benzene/EtOAc = 20/1); 1H NMR (400 MHz, CDCl3): δ 7.93–7.87 (m, 6H), 8.07 (d, J = 8.2 Hz, 2H), 7.63–7.19 (m, 24H), 5.98 (d, J = 14.0 Hz, 2H), 4.59 (d, J = 14.0 Hz, 2H); 13C NMR (100 MHz, CDCl3): δ 166.4, 139.9, 138.9, 135.2, 134.6, 133.9, 133.1, 133.0, 132.3, 130.2, 129.8, 129.3, 128.7, 128.5, 128.4, 127.4, 127.2, 126.9, 126.8, 126.6, 126.5, 48.8; IR (ATR): 3054.0, 1493.6, 1398.7, 899.0, 819.3, 745.7, 694.1 cm-1; HRMS (ESI) [M–Cl–] calcd for C57H36N2Au: 945.2539, found 945.2522, [M–Cl–+NH3] calcd for C57H39N3Au: 962.2804, found 962.2803; mp 319 °C (decomposition); [α]D24 +207 (c 0.33, CHCl3).
(Z)-3-Benzylidene-4-(2-methoxypropan-2-yl)-1,1-bis(phenylsulfonyl)cyclopentane (3a). A suspension of AgNTf2 (0.679 mg, 1.75×10-3 mmol) and NHC-AuCl complex 13 (1.7 mg, 1.75×10-3 mmol) in CH2Cl2 (0.20 mL) was stirred for 30 min at room temperature in the absence of light. To the stirred suspension was added MeOH (0.28 mL) and a solution of 2a16 (16.8 mg, 0.035 mmol) in CH2Cl2 (0.22 mL). After stirring at room temperature for 88 h in the absence of light, the reaction mixture was diluted with CH2Cl2, filtered through a pad of Celite and concentrated under reduced pressure. The residue was purified by silica gel column chromatography to afford 3a (7.1 mg, 40%): Spectroscopic data were in agreement with those previously reported16). Ee was determined by chiral HPLC analysis (Daicel ID-3 column, hexane/2-propanol = 9/1, 1 mL/ min, 254 nm, tR = 21.1 min (minor) and 26.8 min (major), 33% ee): Rf = 0.28 (hexane/EtOAc = 3/1); 1H NMR (400 MHz, CDCl3): δ 8.15–8.08 (m, 4H), 7.75–7.66 (m, 2H), 7.64–7.58 (m, 2H), 7.58–7.52 (m, 2H), 7.34–7.31 (m, 4H), 7.25–7.19 (m, 1H), 6.41 (s, 1H), 3.77 (dd, J = 6.0, 8.7 Hz, 1H), 3.68 (d, J = 15.6 Hz, 1H), 2.94 (s, 3H), 2.87 (dd, J = 6.0, 15.6 Hz, 1H), 2.79 (dd, J = 8.7, 15.6 Hz, 1H), 2.55 (d, J = 15.6 Hz, 1H), 0.89 (s, 3H), 0.80 (s, 3H); [α]D24 +60 (c 0.42, CHCl3, 33% ee).
4-(2-Methoxypropan-2-yl)-3-methylidene-1,1-bis(phenylsulfonyl)cyclopentane (3b). A suspension of AgNTf2 (0.679 mg, 1.75×10-3 mmol) and NHC-AuCl complex 13 (1.7 mg, 1.75×10-3 mmol) in CH2Cl2 (0.22 mL) was stirred for 30 min at room temperature in the absence of light. To the stirred suspension was added MeOH (0.28 mL) and a solution of 2b16 (14.1 mg, 0.035 mmol) in CH2Cl2 (0.20 mL). After stirring at room temperature for 20 min in the absence of light, the reaction mixture was diluted with CH2Cl2, filtered through a pad of Celite and concentrated under reduced pressure. The residue was purified by silica gel column chromatography to afford 3b (15.2 mg, quant): Spectroscopic data were in agreement with those previously reported16). Ee was determined by chiral HPLC analysis (Daicel ID-3 column, hexane/2-propanol = 2/1, 1 mL/ min, 254 nm, tR = 15.8 min (major) and 18.2 min (minor), 78% ee): Rf = 0.22 (hexane/EtOAc = 3/1); 1H NMR (400 MHz, CDCl3): δ 8.07 (d, 7.8 Hz, 2H), 8.05 (d, 7.8 Hz, 2H), 7.71 (t, 7.8 Hz, 1H), 7.71 (t, 7.8 Hz, 1H), 7.60 (dd, J = 7.8, 7.8 Hz, 2H), 7.58 (dd, J = 7.8, 7.8 Hz, 2H), 5.03–4.97 (m, 2H), 3.46 (ddd, 16.9, 6.0, 3.2 Hz, 1H), 3.13 (s, 3H), 3.05–2.98 (m, 1H), 2.77 (d, 16.9 Hz, 1H), 2.73 (dd, J = 15.6, 8.2 Hz, 1H), 2.64 (dd, J = 15.6, 9.2 Hz, 1H), 1.19 (s, 3H), 1.10 (s, 3H); [α]D18 –20 (c 0.24, CHCl3, 78% ee).17
ACKNOWLEDGEMENTS
This work was financially supported in part by a Grant-in-Aid for Challenging Exploratory Research (No. 26670007) from JSPS, Japan and a Waseda University Grant for Special Research Projects.
References
1. A. J. Arduengo III, R. L. Harlow, and M. Kline, J. Am. Chem. Soc., 1991, 113, 361. CrossRef
2. K. Öfele, J. Organometal. Chem., 1968, 12, 42.
3. H.-W. Wanzlick and H.-J. Schönherr, Angew. Chem., Int. Ed. Engl., 1968, 7, 141. CrossRef
4. Selected reviews: (a) W. A. Herrmann and C. Köcher, Angew. Chem., Int. Ed. Engl., 1997, 36, 2162; (b) W. A. Herrmann, Angew. Chem. Int. Ed., 2002, 41, 1290; CrossRef (c) J. C. Y. Lin, R. T. W. Huang, C. S. Lee, A. Bhattacharyya, W. S. Hwang, and I. J. B. Lin, Chem. Rev., 2009, 109, 3561. CrossRef
5. For reviews on NHC as organocatalyst see: (a) D. Enders, O. Niemeier, and A. Henseler, Chem. Rev., 2007, 107, 5606; (b) K. Hirano, I. Piel, and F. Glorius, Chem. Lett., 2011, 40, 786; CrossRef (c) V. Nair, R. S. Menon, A. T. Biju, C. R. Sinu, R. R. Paul, A. Josea, and V. Sreekumarc, Chem. Soc. Rev., 2011, 40, 5336; CrossRef (d) A. Grossmann and D. Enders, Angew. Chem. Int. Ed., 2012, 51, 314. CrossRef
6. For reviews on chiral NHC ligand see: (a) M. C. Perry and K. Burgess, Tetrahedron: Asymmetry, 2003, 14, 951; (b) V. César, S. Bellemin-Laponnaz, and L. H. Gade, Chem. Soc. Rev., 2004, 33, 619. CrossRef
7. (a) M. P. Muñoz, J. Adrio, J. C. Carretero, and A. M. Echavarren, Organometallics, 2005, 24, 1293; (b) C.-M. Chao, E. Genin, P. Y. Toullec, J.-P. Genêt, and V. Michelet, J. Organomet. Chem., 2009, 694, 538; CrossRef (c) C.-M. Chao, M. R. Vitale, P. Y. Toullec, J.-P. Genêt, and V. Michelet, Chem. Eur. J., 2009, 15, 1319. CrossRef
8. (a) I. Alonso, B. Trillo, F. López, S. Montserrat, G. Ujaque, L. Castedo, A. Lledós, and J. L. Mascareñas, J. Am. Chem. Soc., 2009, 131, 13020; (b) H. Teller, S. Flügge, R. Goddard, and A. Fürstner, Angew. Chem. Int. Ed., 2010, 49, 1949; CrossRef (c) A. Z. González, D. Benitez, E. Tkatchouk, W. A. Goddard III, and F. D. Toste, J. Am. Chem. Soc., 2011, 133, 5500; CrossRef (d) I. Alonso, H. Faustino, F. López, and J. L. Mascareñas, Angew. Chem. Int. Ed., 2011, 50, 11496; (e) S. Suárez-Pantiga, C. Hernández-Díaz, E. Rubio, and J. M. González, Angew. Chem. Int. Ed., 2012, 51, 11552; CrossRef (f) C. Michona, F. Medina, M.-A. Abadie, and F. Agbossou-Niedercorn, Organometallics, 2013, 32, 5589; CrossRef (g) C. Michona, M.-A. Abadie, F. Medina, and F. Agbossou-Niedercorn, Catalysis Today, 2014, 235, 2; CrossRef (h) Y. Wang, P. Zhang, Y. Liu, F. Xia, and J. Zhang, Chem. Sci., 2015, 6, 5564; CrossRef (i) S. Klimczyk, A. Misale, X. Huang, and N. Maulide, Angew. Chem. Int. Ed., 2015, 54, 10365. CrossRef
9. G. L. Hamilton, E. J. Kang, M. Mba, and F. D. Toste, Science, 2007, 317, 496. CrossRef
10. (a) Y. Matsumoto, K. B. Selim, H. Nakanishi, K. Yamada, Y. Yamamoto, and K. Tomioka, Tetrahedron Lett., 2010, 51, 404; CrossRef (b) L. Liu, F. Wang, W. Wang, M. Zhao, and M. Shi, Beilstein J. Org. Chem., 2011, 7, 555; CrossRef (c) W. Wang, J. Yang, F. Wang, and M. Shi, Organometallics, 2011, 30, 3859; CrossRef (d) J. Yang, R. Zhang, W. Wang, Z. Zhang, and M. Shi, Tetrahedron: Asymmetry, 2011, 22, 2029; CrossRef (e) K. Yamada, Y. Matsumoto, K. B. Selim, Y. Yamamoto, and K. Tomioka, Tetrahedron, 2012, 68, 4159; CrossRef (f) R. B. Strand, T. Helgerud, T. Solvang, A. Dolva, C. A. Sperger, and A. Fiksdahl, Tetrahedron: Asymmetry, 2012, 23, 1350; CrossRef (g) J. Francos, F. Grande-Carmona, H. Faustino, J. Iglesias-Sigüenza, E. Díez, I. Alonso, R. Fernández, J. M. Lassaletta, F. López, and J. L. Mascareñas, J. Am. Chem. Soc., 2012, 134, 14322.
11. (a) K. Usui and M. Nakada, Heterocycles, 2014, 88, 1539; CrossRef (b) Y. Uetake, T. Niwa, and M. Nakada, Tetrahedron: Asymmetry, 2015, 26, 158; CrossRef (c) K. Usui, T. Yoshida, and M. Nakada, Tetrahedron: Asymmetry, 2016, 27, accepted. CrossRef
12. P. C. B. Page, C. Bordogna, I. Strutt, Y. Chan, and B. R. Buckley, Synlett, 2013, 24, 2067. CrossRef
13. C.-C. Wang, S.-F. Chen, S.-Y. Chou, K.-S. Shih, and S.-S. T. Chen, U. S. Pat. Appl. Publ., 2006/0287500.
14. A. Merzouk and F. Guibé, Tetrahedron Lett., 1992, 33, 477. CrossRef
15. CCDC 1443479 (13) contain the supplementary crystallographic data for this paper. These data can be obtained free of charge from The Cambridge Crystallographic Data Centre via www.ccdc.cam.ac.uk/data_request/cif.
16. M. Méndez, M. P. Muñoz, C. Nevado, D. J. Cárdenas, and A. M. Echavarren, J. Am. Chem. Soc., 2001, 123, 10511.
17. The absolute value of the specific rotation of 3b ([α]D18 –20 (c 0.24, CHCl3, 78% ee)) was inconsistent with those reported by other research groups ([α]D25 +21.2 (c 0.4, CHCl3, 53% ee)7a; [α]D25 –20.5 (c 0.97, CHCl3, 52% ee)10e) when compared their ee values with that of 3b, but the ee of 3b was confirmed by independent HPLC analyses using different chiral columns, Daicel ID-3 and AD-H (see Supporting Information).