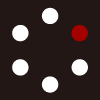
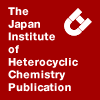
HETEROCYCLES
An International Journal for Reviews and Communications in Heterocyclic ChemistryWeb Edition ISSN: 1881-0942
Published online by The Japan Institute of Heterocyclic Chemistry
e-Journal
Full Text HTML
Received, 19th January, 2016, Accepted, 3rd February, 2016, Published online, 8th February, 2016.
DOI: 10.3987/COM-16-13410
■ Antioxidant Activity of Ascorbic Acid Analogs Containing a Nitrogen Atom in the Ring
Shogo Nomura, Keiko Inami, and Masataka Mochizuki*
Faculty of Pharmaceutical Sciences, Tokyo University of Science, 2641 Yamazaki, Noda-shi, Chiba 278-8510, Japan
Abstract
Ascorbic acid (AscH2) is a powerful antioxidant and protects the human body from oxidative stresses by scavenging reactive oxygen species. AscH2 analogs containing a carbonyl conjugated ene-diol structure and endocyclic nitrogen atom were evaluated for their oxidation potentials by cyclic and differential pulse voltammetry. The oxidation potentials of AscH2 analogs in which the endocyclic oxygen atom of AscH2 was replaced by a single nitrogen atom were lower than those of AscH2 under both non-aqueous and aqueous conditions. The results demonstrate that these analogs can have powerful antioxidant activities under physiological conditions.introduction
Reactive oxygen species (ROS) serve as signaling molecules to regulate biological and physiological processes;1 however, ROS can react with DNA, proteins, and other cellular components to become problematic.2 ROS are strongly implicated in the pathophysiology of diseases, such as cancer, neurodegenerative, and cardiovascular disease, as well as in the degenerative process associated with aging.3-6 The cells withstand and counteract ROS occurrence by the use of several different defense mechanisms ranging from radical scavengers, such as ascorbic acid (AscH2), α-tocopherol, and glutathione, to antioxidant enzymes, such as catalase, superoxide dismutase, and various peroxidases.7 Thus, antioxidant compounds and antioxidant enzymes have been extensively studied for their capacity to protect organisms and cells from damage brought on by oxidative stress.8, 9
Antioxidant compounds can act as reducing agents and, in solutions, they tend to be easily oxidized on inert electrodes. The relationship between the electrochemical behavior of compounds and their antioxidant activity is very interesting because low redox potentials correspond to high antioxidant activity.10 AscH2 is known for its powerful reducing properties, being easily oxidized to dehydroascorbic acid.11 Thus, many derivatives of AscH2 have been developed to improve stability and lipophilicity.12-14 In our previous paper, we reported the synthesis and radical scavenging activity against the galvinoxyl radical of AscH2 analogs 1–6 (Figure 1) containing a carbonyl conjugated ene-diol structure.15 In this study, the redox potential of AscH2 analogs in methanol and phosphate buffer (pH 7.4) was evaluated to understand the chemical properties of the AscH2 analogs with a nitrogen atom in the ring, for antioxidant activity.
RESULTS AND DISCUSSION
Synthesis of AscH2 analogs
AscH2 analogs were prepared by the method shown in our previous paper.15
Redox potential of AscH2 analogs
To determine the antioxidant activity of AscH2 analogs with a nitrogen atom in the ring, the redox potentials of the compounds were determined by cyclic voltammetry in deaerated methanol and phosphate buffer (pH 7.4). Because polar antioxidants are known to be more effective in less polar media, the chemistry of AscH2 and AscH2 analogs in both non-aqueous and aqueous media was vital for understanding their antioxidant activities.16 The cyclic voltammograms of AscH2 and its analogs show only an anodic oxidation peak (Figure 2).17 The oxidation of AscH2 analogs at the electrode was irreversible; thus, we evaluated the oxidation potentials by differential pulse voltammetry (Figure 3). The differential pulse width method can decrease the effect of charging current interference;18 therefore, it is far more sensitive than cyclic voltammetry for examining the electrochemical behavior of reactant molecules that bind to the electrode surface or irreversibly react with the electrode.19, 20 The measured oxidation potentials of all compounds are summarized in Table 1.
The oxidation potentials in methanol decrease in the following order: AscH2 ≥ 1 > 2 > 6 = 5 > 3 > 4 (Figure 3A, Table 1). The first oxidation potentials (Epa1) represent the one electron transfers with the formation of intermediate radicals. AscH2 and 1 showed only one oxidation potential wave; however, the other AscH2 analogs 2–6 showed two oxidation waves. The observation of two oxidation waves for 2–6 indicate that their intermediate radicals (Scheme 1) are more stabilized than that of AscH2 and 1. The separation between the two oxidation potentials (ΔE) of nitrogen-containing AscH2 analogs 3–6 are much larger than that for 2 (Table 1). These results indicate the extent of stabilization of the nitrogen-containing AscH2 analog radicals results from overlap between the lone pair of the endocyclic nitrogen atom and the π-electron system of the carbonyl and conjugated ene-diol groups (Scheme 1).21,22 Among the nitrogen-containing AscH2 analogs, N-ethylated 4 had the lowest oxidation potential, resulting from the electron-donating inductive effect. In contrast, 5 and 6 substituted with electron-withdrawing groups show higher oxidation potentials than 3. These results indicate that a nitrogen atom with a more electron-donating resonance effect than an oxygen atom better stabilizes the electron-deficient intermediate radical [R•] of AscH2 analogs, weakening the O–H bond and accelerating the oxidation reaction (Scheme 1).23
We previously reported that the galvinoxyl radical scavenging activity of AscH2 analogs increase in the following order: AscH2 ≤ 1 ≤ 2 << 6 < 3 < 5 (Table 1).15 The lower oxidation potential of AscH2 analogs correlates with the galvinoxyl radical scavenging activity (Figure 4). This correlation is in accordance with the relationship of low redox potential corresponding to high antioxidant activity.10
The oxidation potential in phosphate buffer (pH 7.4) decreased in the following order: 2 > 1 ≥ AscH2 > 6 > 5 ≥ 3 > 4 (Figure 3B, Table 1). The oxidation potentials of AscH2 and its analogs under neutral conditions were lower than those in methanol. The ionized form of AscH2 and its analogs have more powerful antioxidant activity than those in the protonated form.23 Under neutral conditions, oxidation proceeds via intermediate AscH2 analog radical anions [R•−] (Scheme 2).24 The first oxidation potentials (Epa1) show the one electron transfers with the formation of intermediate radical anions.
The oxidation potential of 1 was slightly higher than that of AscH2. Therefore, the side chain of AscH2 is not necessary for the oxidation reaction. 2 has a higher oxidation potential than 1. An oxygen atom, with a more electron-withdrawing inductive effect than a carbon atom, better stabilizes the radical anion of 1 than that of 2; however, 3–6, with more electron-donating resonance effects from the nitrogen atom, have lower oxidation potentials than 1. Through the electron-donating resonance effect, the lone pair of the nitrogen atom in the ring destabilizes the anions of 3–6 more than the anion of 1. Thus, the nitrogen AscH2 analogs 3–6 have a lower oxidation potential than 1 in neutral aqueous solutions. The oxidation potential of 6 was the highest among these compounds. The electron-withdrawing resonance effect of the acetyl group stabilized the anion of 6. These results indicate that the lone pair of an endocyclic nitrogen atom is important for promotion of the oxidation reaction of AscH2 analogs under neutral conditions. Compared with 3, 4 has a lower oxidation potential and 5 has a higher oxidation potential. The electron-donating ethyl group in 4 increased the electron density of the endocyclic nitrogen atom and destabilized the anion of 4, relative to 3. Conversely, the electron-withdrawing cyanomethyl group in 5 stabilized its anion and increased its oxidation potential.
CONCLUSION
We have previously reported the rate constant of the reaction between AscH2 analogs and galvinoxyl radicals,15 and the radical scavenging activity and oxidation potential in methanol was found to have a correlation. This result indicated that the oxidation reaction of AscH2 analogs proceeds by hydrogen atom transfer via their AscH2 analog radicals. The stabilization of the AscH2 analog radical was the key factor for decreasing their oxidation potential in this mechanism. In addition, the oxidation potential of the nitrogen AscH2 analogs, under neutral conditions, are lower than any other AscH2 analogs. The nitrogen AscH2 analogs have a low oxidation potential in both non-aqueous and aqueous solutions. The synthesized nitrogen AscH2 analogs should have powerful antioxidant activities under physiological conditions.
EXPERIMENTAL
Reagents
MeOH was obtained from Dojindo (Kumamoto, Japan). Tetrabutylammonium perchlorate was obtained Tokyo Chemical Industry (Tokyo, Japan). Another reagents and solvents were purchased from Wako pure Chemical Industries (Tokyo, Japan).
Synthesis
AscH2 analogs 1–6 were prepared as previously reported.15
Electrochemistry
The cyclic and differential pulse voltammetry was measured using a potentiostat with a glassy carbon working electrode, a platinum counter electrode, and a Ag/AgNO3 reference electrode in MeOH, or a Ag/AgCl reference electrode in 0.1 M phosphate buffer. The glassy carbon electrode (1 mm diameter) was carefully polished with alumina powder (0.3 µm) on a polishing cloth before each measurement. Voltammograms were obtained in deaerated MeOH using tetrabutylammonium perchlorate (100 mM) as an electrolyte and phosphate buffer (pH 7.4), at 25 °C. For compounds displaying reversible and irreversible redox chemistry, cyclic voltammograms were obtained using a scan rate of 100 mV/s and differential pulse voltammograms were obtained using a pulse amplitude of 50 mV, a pulse width of 60 mV, a pulse period of 0.02 s, and a scan rate of 20 mV/s.25
References
1. T. Finkel, J. Cell Biol., 2011, 194, 7. CrossRef
2. B. C. Dicknson and C. J. Chang, Nat. Chem. Biol., 2012, 7, 504. CrossRef
3. B. Halliwell and J. M. Gutteridge, Free Radicals in Biology and Medicine, 3rd ed., Oxford University Press, Oxford, UK, 1999.
4. M. H. Yan, X. Wang, and X. Zhu, Free Radic. Biol. Med., 2013, 62, 90. CrossRef
5. K. Sugamura and J. Keaney Jr., Free Radic. Biol. Med., 2011, 51, 978. CrossRef
6. T. Finkel and N. J. Holbrook, Nature, 2000, 408, 239. CrossRef
7. T. B. Kryston, A. B. Georgiev, P. Pissis, and A. G. Georgakilas, Mutat. Res., 2011, 711, 193. CrossRef
8. J. Sochor, J. Dobes, O. Krystofova, B. Ruttkay-Nedecky, P. Babula, M. Pohanka, T. Juriova, O. Zitka, V. Adam, B. Klejdus, and R. Kizek, Int. J. Electrochem. Sci., 2013, 8, 8464.
9. J. M. Mates, Toxicology, 2000, 153, 83. CrossRef
10. L. Barros, S. Falcão, P. Baptista, C. Freire, M. Vilas-Boas, and I. C. F. R. Ferreira, Food Chem., 2008, 111, 61. CrossRef
11. J. Du, J. J. Cullen, and G. R. Buettner, Biochem. Biophys. Acta, 2012, 1826, 443.
12. J. Takebayashi, A. Tai, E. Gohda, and I. Yamamoto, Biol. Pharm. Bull., 2006, 29, 766. CrossRef
13. R. Han, L. Liu, J. Li, G. Du, and J. Chen, Appl. Microbiol. Biotechnol., 2012, 95, 313. CrossRef
14. N. Kharrat, I. Aissa, M. Sghaier, M. Bouaziz, M. Sellami, D. Laouini, and Y. Gargouri, J. Agric. Food Chem., 2014, 62, 9118. CrossRef
15. S. Nomura, K. Inami, and M. Mochizuki, Heterocycles, 2016, 92, 86. CrossRef
16. F. Shahidi and Y. Zhong, J. Agric. Food Chem., 2011, 9, 3499. CrossRef
17. A. M. Pisoschi, A. Pop, A. I. Serban, and C. Fafaneata, Electrochim. Acta, 2014, 121, 443. CrossRef
18. E. P. Parry and R. A. Osteryoung, Anal. Chem., 1965, 37, 1634. CrossRef
19. B. K. Chethana and Y. A. Naik, Anal. Methods, 2012, 4, 3754. CrossRef
20. W. R. Heineman and P. T. Kissinger, Anal. Chem., 1978, 50, 166. CrossRef
21. K. U. Ingold and D. A. Pratt, Chem. Rev., 2014, 114, 9022. CrossRef
22. T. Nam, J. Ku, C. L. Rector, H. Choi, N. A. Porter, and B. Jeong, Org. Biomol. Chem., 2011, 9, 8475. CrossRef
23. L. Valgimigli and D. A. Pratt, Acc. Chem. Res., 2015, 48, 966. CrossRef
24. I. G. Casella and M. R. Guascito, Electroanal., 1997, 9, 1381. CrossRef
25. I.–F. Hu and T. Kuwana, Anal. Chem., 1986, 58, 3235. CrossRef