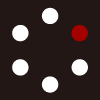
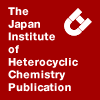
HETEROCYCLES
An International Journal for Reviews and Communications in Heterocyclic ChemistryWeb Edition ISSN: 1881-0942
Published online by The Japan Institute of Heterocyclic Chemistry
e-Journal
Full Text HTML
Received, 22nd January, 2016, Accepted, 9th February, 2016, Published online, 16th February, 2016.
DOI: 10.3987/COM-16-13414
■ Phase-Transfer Catalyzed Sulfenylation of 3-Substituted-2-oxindoles
Kazuhiro Nagata, Daisuke Sano, Osamu Aoyama, Takuya Kanemitsu, Michiko Miyazaki, Yuki Odanaka, Ayano Machida, and Takashi Itoh*
School of Pharmaceutical Sciences, Showa University, 1-5-8 Hatanodai, Shinagawa-ku, Tokyo 142-8555, Japan
Abstract
Optically active 3-sulfenyl-2-oxindoles were synthesized by a catalytic asymmetric substitution with a chiral phase-transfer catalyst. The reaction was suggested to proceed in a radical mechanism.3-Substituted oxindoles have received much attention due to their diverse biological and pharmaceutical activities,1 thus their asymmetric synthesis has been intensively investigated in recent years. Among the syntheses of 3-substituted oxindoles, those of 3-sulfenyl-2-oxindoles were less explored. Recently, anticancer, antifungal, and antitubercular activities have been found in many oxindoles with a thio group at the chiral carbon center.2 Therefore, the methods for the synthesis of 3-sulfenyloxindoles have become to be widely investigated.3 Among these methods, direct sulfenylation is the most straightforward. Only recently, asymmetric sulfenylations of 3-substituted oxindoles catalyzed by a chiral organocatalyst were independently reported by Enders, Cheng, and Jiang.4 In addition, Feng et al. reported similar sulfenylation catalyzed by a chiral N,N’-dioxide-Sc(OTf)3 complex.5 We have been investigating catalytic asymmetric construction of quaternary carbon center catalyzed by a chiral phase-transfer catalyst (PTC), and found that 3-hydroxyoxindoles are obtained in high yields and high enantioselectivities using cinchonidine derived PTC as an organocatalyst.6 The method is operationally simple and environmentally benign, thus we became interested in the application of the method to the synthesis of 3-sulfenyloxindoles. In this paper we report a direct sulfenylation of 3-substituted oxindoles with diphenyl disulfide catalyzed by PTC.
In our previous work,6 hydroxylation of oxindole under phase-transfer catalyzed conditions was assumed to proceed through a radical intermediate, which reacted with oxygen to give hydroxylated product.
Therefore, the sulfenylation was investigated using diphenyl disulfide which could afford the sulfenylated product by a radical process. Initially, we carried out the sulfenylation of 3-allyl-2-oxindole using an achiral tetrabutylammonium iodide (TBAI) as a PTC, and found that sulfenylated product was obtained in
a quantitative yield (eq.1). We thus investigated asymmetric sulfenylation with chiral PTCs which were commercially available (Table 1), and the result was that cinchona alkaloid derived PTC gave moderate to good enantioselectivities, while spirobinaphthyl quaternary ammonium salt 2 and tartrate derived bis-ammonium salt 3 gave low enantioselectivities. When trifluoromethylbenzyl substituted cinchoninium salt 1b was used, the best yield and enantioselectivity were obtained. The absolute configuration of the major isomer of 5a was determined after removal of the PMB group, by comparison of the retention time in chiral HPLC analysis with that of literature.5 Next we investigated scope of the substrate using 1b as the PTC (Table 2). The result was that generally high chemical yields and moderate enantioselectivities were obtained. Phenyl substituted oxindole, however, gave low enantioselectivity (entry 13). To consider the reaction mechanism, we also conducted the reaction using DMPO as a radical trapping reagent.
When 5 equivalent of DMPO was used instead of diphenyl disulfide, radical coupling product 6 was obtained in 16% yield accompanied with hydroxy oxindole 7, which would be produced via the reaction with solvated oxygen (Scheme 1). The result indicated the presence of a radical intermediate in the course of the reaction, and a plausible reaction mechanism was shown in Scheme 2.
Ammonium enolate 8, which is generated by the counter cation exchange with potassium enolate, transfers a single electron to diphenyl disulfide to produce a radical intermediate 9 and a benzenethiyl radical.7 Radical coupling of 9 with benzenethiyl radical occurs to afford sulfenyloxindole 5. Concomitantly generated ammonium thiolate changes the counter anion with potassium salt of oxindole to give ammonium enolate 8. Therefore, asymmetric induction is thought to occur in the radical coupling step. In the general phase transfer catalytic reactions, asymmetric induction occurs when a salt complex of enolate with PTC reacts with an electrophile. In the present reaction, the radical coupling is considered to occur before the electrically neutral radical separates from the chiral ammonium ion, because moderate to good enantioselectivities were obtained.
In conclusion, we have described asymmetric sulfenylation of oxindoles using a chiral PTC derived from Cinchona alkaloids which are readily available. The reaction was suggested to proceed by a radical mechanism which has not reported so far in the reaction with PTC except our example.6 The present method would be also applicable for the C-C bond formation, thus syntheses of 3,3-bis-alkyl substituted 2-oxindoles which cannot be obtained by the ionic reaction are now under investigation.
ACKNOWLEDGEMENTS
This work was supported in part by a Grant-in-Aid for Scientific Research from the Ministry of Education, Culture, Sports, Science and Technology of Japan.
References
1. For reviews, see: (a) F. Zhou, Y.-L. Liu, and J. Zhou, Adv. Synth. Catal., 2010, 352, 1381; (b) C. Marti and E. M. Carreira, Eur. J. Org. Chem., 2003, 2209; CrossRef (c) C. V. Galliford and A. Scheidt, Angew. Chem. Int. Ed., 2007, 46, 8748; CrossRef (d) N. R. BallJone, J. J. Badille, and A. K. Franz, Org. Biomol. Chem., 2012, 10, 5165; (e) B. M. Trost and M. K. Brennan, Synthesis, 2009, 3003. CrossRef
2. (a) R. G. Mehta, J. R. G. Liu, A. Constantinou, M. Hawthorne, Pezzuto, R. C. Moon, and R. M. Moriarty, Anticancer Res., 1994, 14, 1209; (b) M. S. C. Pedras and M. Hossain, Org. Biomol. Chem., 2006, 4, 2581; CrossRef (c) A. Dandia, M. Sati, K. Arya, R. Sharma, and A. Loupy, Chem. Pharm. Bull., 2003, 51, 1137. CrossRef
3. (a) Y. Li, Y. Shi, Z. Huang, X. Wu, P. Xu, J. Wang, and Y. Zhang, Org. Lett., 2011, 13, 1210; CrossRef (b) D. B. England, G. Merey, and A. Padwa, Heterocycles, 2007, 74, 491; CrossRef (c) H. Chen and D. Shi, Tetrahedron, 2011, 67, 5686; CrossRef (d) M. Miller, J. C. Vogel, W. Tsang, A. Merrit, and J. D. Procter, Org. Biomol. Chem., 2009, 7, 589; CrossRef (e) M. Miller, W. Tsang, A. Merrit, and J. D. Procter, Chem. Commun., 2007, 498; CrossRef (f) L. A. McAllisster, R. A. McCormick, S. Brand, and J. D. Procter, Angew. Chem. Int. Ed., 2005, 44, 452; CrossRef (g) A. Dandia, K. Arya, M. Sati, and S. Gautam, Tetrahedron, 2004, 60, 5253; CrossRef (h) M. F. Greaney and W. B. Motherwell, Tetrahedron Lett., 2000, 41, 4467; CrossRef (i) X. Dou, B. Zhou, W. Yao, F. Zhong, C. Jiang, and Y. Lu, Org. Lett., 2013, 15, 4920. CrossRef
4. (a) C. Wang, X. Yang, C. C. J. Loh, G. Raabe, and D. Enders, Chem. Eur. J., 2012, 18, 11531; CrossRef (b) X. Li, C. Liu, X.-S. Xue, and J.-P. Cheng, Org. Lett., 2012, 14, 4374; CrossRef (c) Z. Han, W. Chen, S. Dong, C. Yang, H. Liu, Y. Pan, L. Yan, and Z. Jiang, Org. Lett., 2012, 14, 4670; CrossRef (d) L. Huang, J. Li, Y. Zhao, X. Ye, Y. Liu, L. Yan, C.-H. Tan, H. Liu, and Z. Jiang, J. Org. Chem., 2015, 80, 8933. CrossRef
5. Y. Cai, J. Li, W. Chen, M. Xie, X. Liu, L. Lin, and X. Feng, Org. Lett., 2012, 14, 2726. CrossRef
6. D. Sano, K. Nagata, and T. Itoh, Org. Lett., 2008, 10, 1593. CrossRef
7. (a) T. Taniguchi, T. Fujii, A. Iota, and H. Ishibashi, Org. Lett., 2009, 11, 3298; CrossRef (b) M. Z. Hoffman and E. Hayon, J. Am. Chem. Soc., 1972, 94, 7950 CrossRef