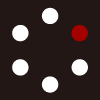
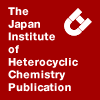
HETEROCYCLES
An International Journal for Reviews and Communications in Heterocyclic ChemistryWeb Edition ISSN: 1881-0942
Published online by The Japan Institute of Heterocyclic Chemistry
e-Journal
Full Text HTML
Received, 22nd February, 2016, Accepted, 24th March, 2016, Published online, 18th April, 2016.
■ A Biomimetic Approach to the Synthesis of Terpene-Amino Acid Conjugates. The Ugi Reaction in the Hypothetical Biosynthesis of Marine Natural Products
Yoshiyasu Ichikawa,* Kenta Saito, Rika Mimura, Ayumi Kitamori, Akihito Matsukawa, Akifumi Ikeda, Toshiya Masuda, Hiyoshizo Kotsuki, and Keiji Nakano
Faculty of Science, Kochi University, 2-5-1, Akebono-cho, Kochi 780-8520, Japan
Abstract
A unique pathway for the biosynthesis of the marine sponge terpenes, exigurin and boneratamides A–C, is proposed. Based on this proposal, a biomimetic strategy on key Ugi coupling reactions between terpene isocyanides and amino acids was developed for construction of the core structures of exigurin and boneratamides A–C.introduction
In 2003, Ohta and Ikegami at Hiroshima University reported the isolation and structural elucidation of exigurin (1) along with (–)-10-epi-axisonitrile-3 (2) from the marine sponge Geodia exigua collected in the Oshima Island in the Kagoshima Prefecture of Japan (Figure 1).1 While (–)-10-epi-axisonitrile-3 (2) is a typical marine terpene isocyanide, previously isolated from the nudibranch Phylliella pustulosa,2 exigurin (1) has an unprecedented structure in which a terpene unit and sarcosine (N-methylglycine) are joined through an amide linkage. Although Garson and Simpson suggested that (–)-10-epi-axisonitrile-3 (2) plays a possible precursor role in the biosynthesis of exigurin, questions have arisen about the metabolic origin of this marine sponge terpene-amino acid conjugate.3
In 2004, Andersen and co-workers at the University of British Columbia reported the isolation of boneratamides A–C from the marine sponge Axinyssa aplysinoides collected in Indonesia.4 X-Ray crystallographic analysis of the methyl ester of boneratamide A (4) established the relative stereochemistry of boneratamide A (3) as represented in Figure 2 (only one enantiomer with C-23S absolute configuration is shown). Boneratamide A is a terpene-amino acid conjugate in which the terpene unit and pyroglutamic acid are connected through a 2-aminoisobutyramide moiety. The structures of boneratamides B and C (5) were elucidated by extensive NMR spectroscopic analysis on the corresponding methyl esters 6, which revealed that boneratamides B and C are stereoisomers differing in the relative configuration at either one or both of the C18 and C23 stereogenic centers. Like boneratamide A (3), boneratamides B and C (5) are conjugates of a terpene unit and pyroglutamic acid.
In 2004, Andersen and co-workers at the University of British Columbia reported the isolation of boneratamides A–C from the marine sponge Axinyssa aplysinoides collected in Indonesia.4 X-Ray crystallographic analysis of the methyl ester of boneratamide A (4) established the relative stereochemistry of boneratamide A (3) as represented in Figure 2 (only one enantiomer with C-23S absolute configuration is shown). Boneratamide A is a terpene-amino acid conjugate in which the terpene unit and pyroglutamic acid are connected through a 2-aminoisobutyramide moiety. The structures of boneratamides B and C (5) were elucidated by extensive NMR spectroscopic analysis on the corresponding methyl esters 6, which revealed that boneratamides B and C are stereoisomers differing in the relative configuration at either one or both of the C18 and C23 stereogenic centers. Like boneratamide A (3), boneratamides B and C (5) are conjugates of a terpene unit and pyroglutamic acid.
In an extension of the U-4CR, bifunctional reagents in which the participating functional groups are present in a single component, have been used to construct unique structures such as β-lactams and iminodicarboxylic acids. In the route for β-lactam synthesis, β-amino acids serve as bifunctional starting materials instead of the amine and carboxylic acid components (Scheme 2).7 A plausible reaction mechanism involves initial condensation of the β-amino acid with the carbonyl component to form a Schiff’s base, which then reacts with the isocyanide to form a seven-membered ring intermediate I. Intramolecular O-to-N-acyl migration of I results in the formation of the β-lactam II. This type of the process for β-lactam synthesis is referred to as the Ugi-four-center three-component reaction (U-4C-3CR).8
A modification of the U-4CR using amino acids as bifunctional starting materials is classified as the Ugi five-center four-component reaction (U-5C-4CR) (Scheme 3).9 The proposed reaction mechanism of the U-5C-4CR begins with condensation of the amino acid with the aldehyde to form the corresponding protonated Schiff’s base I. Addition of the isocyanide then produces the cyclic O-acyl-imide II. High strain energy associated with the formation of α-lactam ring prevents II from undergoing intramolecular O-to-N-acyl migration. As a result, nucleophilic attack of the fourth component, such as methanol used as the solvent, occurs at the carboxylic carbon (the fifth reacting center). Subsequent six-membered ring opening leads to the formation of the iminodicarboxylic acid derivative III. It should be noted that this process creates a stereogenic center in the product III.
Taking U-4CR, U-4C-3CR and U-5C-4CR processes into consideration, analysis of the structures of exigurin (1), boneratamide A (3), boneratamides B and C (5) led us to recognize the structural resemblance among these terpene-amino acid conjugates. Specifically, these linked terpene-amino acid conjugates possess a common structural motif of iminodicarboxylic acid moiety, which connects the terpene and amino acid residues. This type of structural motif has been known as a product of the Ugi reaction in the field of organic syntheses. Although the Ugi reaction has been used extensively in combinatorial, diverse oriented synthesis,10 it is quite surprising for us to imagine that Nature employs a similar approach to build up a diversity of secondary metabolites in biosynthesis. We now report a further development and observations of our studies aiming at a biomimetic approach to the synthesis of terpene-amino acid conjugates exigurin and boneratamides A–C.
RESULTS AND DISCUSSION
Two hypothetical biosynthetic pathways for construction of the right hand, amide portion of exigurin are presented in Scheme 4. The key step in each route, involving a ugiase catalyzed U-5C-4CR, suggests that exigurin may be traced back to three building blocks consisting of (–)-10-epi-axisonitrile-3 (2) and two other simple precursors such as formaldehyde and either sarcosine or glycine. The Ugi reaction is known to be promoted by an enzyme,11 and this precedent led us to propose a putative ugiase enzyme that catalyzes the Ugi process in a manner reminiscent of the Ugi reaction in organic synthesis. In the clockwise hypothetical pathway, the U-5C-4CR of (–)-10-epi-axisonitrile-3 (2) with the iminium ion formed from formaldehyde and sarcosine generates the cyclic O-acyl imidate 7, which reacts with methanol to furnish exigurin (1). In the other route, the U-5C-4CR of formaldehyde and glycine with the isocyanide 2 produces 8, which is transformed to exigurin (1) by the action of methyltransferase.
Reaction of t-butyl isocyanide (9) with sarcosine (10) and aqueous formaldehyde was selected to evaluate the feasibility of the Ugi processes that take place in the hypothetical biosynthetic pathway (Scheme 5). The U-5C-4CR of these substances in methanol took place smoothly at room temperature to form 11 albeit in low yield (24%). After unsuccessful attempts to improve the yields of this process, the alternative hypothetical biosynthesis scenario shown in Scheme 4 was explored. Since the use of glycine as a substrate of the Ugi reaction leads to over-Ugi products,12 N-protected glycine was utilized as the amino acid substrate. To our delight, the U-5C-4CR using t-butyl isocyanide (9), N-benzylglycine (12) and paraformaldehyde generated amide 13 in 73% yield. Removal of the benzyl group in 13 followed by the reductive amination of 14 under the Eschweiler-Clarke reaction conditions furnished 11 in 60% yield in two steps.
We next turned our attention to synthesize a terpene-amino acid conjugate, which possesses the structural motif corresponding to the right part of exigurin (Scheme 6). The terpene isocyanide 15, prepared from (–)-menthol by a method we previously developed,12a was treated with N-benzyl glycine 12 and paraformaldehyde in methanol under similar conditions to those depicted in Scheme 5. This U-5C-4CR proceeded uneventfully to afford 16 in 87% yield. Hydrogenolysis of the benzylamine in 16 produced 17, which was subjected to the Eschweiler-Clarke reaction conditions. To our surprise, we observed a considerable amount of a byproduct, the structure of which was tentatively assigned as cyclic amide 19. Separation of 19 was difficult due to its similar TLC behavior with 18. In contrast to the low-yield observed for reaction of t-butyl isocyanide (9), the U-5C-4CR of the terpene isocyanide 15 with sarcosine (10) and aqueous formaldehyde produced 18 in 40% yield. 1H and 13C NMR data for exigurin (1), model compound 11 and despiro-exigurin 18 are summarized in Table 1, which shows that the NMR data of both 11 and 18 are in good accord with those reported for exigurin (1). Thus, although the yield is modest, synthesis of despiro-exigurin 18 having the right structure found in exigurin was achieved through operationally simple one-pot U-5C-4CR process.
Two retrosynthetic scenarios, based on the hypothetical biosynthesis pathway, can be envisaged for the synthesis of boneratamides A–C (Scheme 7). A plausible intermediate in the biosynthetic route to boneratamides A–C is axisonitrile-3 (20), a terpene isocyanide previously isolated from the marine sponge Axinella cannabina.13 Based on this proposal, boneratamides A–C can be envisioned to arise from three building blocks including axisonitrile-3 (20), either acetone or acetaldehyde, and glutamic acid (21). Moreover, because U-4C-3CR of β-amino acids leads to β-lactams (Scheme 2), we surmised that corresponding reactions of γ-amino acids such as glutamic acid would produce γ-lactam ring systems present in boneratamides A–C. Another possible retrosynthetic scenario for the preparation of boneratamides A–C follows a stepwise sequence starting with U-5C-4CR of glutamic acid (21) as a bifunctional reagent and water as the fourth component to form 22, which subsequently cyclizes to form γ-lactam core structure in boneratamides A–C.
Initial studies probing these scenarios focused on the synthesis of the right part of boneratamide A employing t-butyl isocyanide (9) as a model substrate (Scheme 8). We observed that U-4C-3CR of 9, acetone (10 equiv) and α-methyl L-glutamate (23) (1.0 equiv) in methanol proceeds smoothly at 50 °C to produce the γ-lactam 25 (69%) that possesses the structural motif found in boneratamide A methyl ester (4) (Scheme 8, entry A). A plausible mechanism for formation of 25 involves generation of the oxazepinone
intermediate 24, which then undergoes intramolecular O-to-N-acyl migration. In the case of the U-4C-3CR using glutamic acid 21 as the substrate, a mixture of methyl ester 25 and carboxylic acid 26 was isolated in 19% and 10% yield, respectively (entry B). Note that the structure of free carboxylic acid in 26 corresponds to the right hand structure of boneratamide A.
We next explored the synthesis of the despiro analogue of boneratamide A employing a terpene isocyanide 27,14 which was prepared from (+)-menthol (Scheme 9). The U-4C-3CR of terpene isocyanide 27 using reaction conditions similar to those described in Scheme 8 furnished the desired despiro-boneratamide A 28 in 67% yield. It should be noted that in this highly efficient one-pot process, conjugation of terpene with amino acid took place along with γ-lactam ring formation. 1H and 13C NMR analysis of 28 shows an approximately 1:1 mixture of amide rotamers. Fortunately, we obtained a nice crystalline 28, and X-ray crystallographic analysis of 28 leads to an unambiguous assignment of its structure.
Synthesis of the right-hand portion of boneratamides B and C was explored using the reaction conditions established during the model synthesis of boneratamide A (Scheme 10). Disappointingly, the U-4C-3CR employing t-butyl isocyanide (9), acetaldehyde and α-methyl L-glutamate 23 produced a 1:1 mixture of products 29 in low yields. Moreover, the diastereomers display similar TLC behavior, which hampered separation and identification.
In order to improve yields and to obtain each stereoisomer 29 in pure form, we turned our attention to an alternative stepwise approach, which corresponds to the second hypothetical biosynthetic route depicted in Scheme 7. In this way, the U-5C-4CR of t-butyl isocyanide (9), acetaldehyde and γ-methyl L-glutamate (30) provided a 3:1 mixture of products 31a and 31b in 37% yield (Scheme 11). To our delight, this mixture could be separated and each respective isomer was cyclized by heating in toluene to furnish 29a and 29b in 77% and 71% respective yields. Fortunately, the major stereoisomer 29a is crystalline and the newly formed stereogenic center in 29a was firmly secured by X-ray crystallographic analysis to assign the R absolute configuration.
We next investigated to synthesize despiro analogues of boneratamides B and C using U-4C-3CR (Scheme 12). Unfortunately, the U-4C-3CR of terpene isocyanide 27, acetaldehyde and α-methyl L -glutamate 23 produced an intractable tarry reaction products and none of the desired 33 was detected by 1H NMR and TLC analysis of the crude products. One of the isolable and identified by-products was 32, which presumably arose via the Passerini reaction of isocyanide 27 with acetaldehyde followed by the Cannizzaro reaction.
The stepwise U-5C-4CR approach was then employed to synthesize despiro analogues of boneratamides B and C 33 (Scheme 13). In this route, reaction of the terpene isocyanide 27 using conditions similar to those described in Scheme 11 afforded an inseparable mixture of the products 34 in 28% yield. Heating a solution of this mixture 34 in refluxing toluene facilitated cyclization to form a 3:2 mixture of γ-lactams 33a and 33b in 80% yield. Repeated chromatographic separations gave isomer 33a as a crystalline substance. X-Ray crystallographic analysis confirmed the structure of 33a and the newly formed stereocenter corresponding to the C18 positions of boneratamides B and C was assigned to be R configuration.
The comparison of the NMR data of 33a and 33b with those of natural boneratamide B and C methyl esters is summarized in Table 2. The 1H and 13C NMR data for the methyl esters of boneratamides B and C were found to compare favourably with those of the despiro analogues 33a and 33b. However, characteristic resonances that would enable differentiation of boneratamides B and C as well as 33a and 33b were not present in the spectra. Although the assignment of the configurations to the C18 stereocenters in natural boneratamides B and C remains unclear, X-ray analysis of 33a coupled with comparison of NMR data in Table 2 confirms the proposed structures of natural boneratamides B and C.
Conclusions
Motivated by the belief that ‘molecules in nature obey the rules of organic chemistry and follow nonenzymatic mechanism’,15 the Ugi chemistry presumably carried out by marine organisms was explored on the bench in the laboratory. Our research endeavour along this line led to the synthesis of terpene-amino acid conjugates that have identical structural motifs found in exigurin and boneratamides A–C in a manner pertinent to our proposed biogenetic pathway. In particular, the synthesis of despiro analogue of boneratamide A 28 showed remarkable efficiency to assemble multiple components in a one-pot process. The results of the current study shed light on a long-standing mystery relating to the biosynthetic origin of exigurin and boneratamides. The Ugi type reaction is not confined to the realm of organic synthesis, and it is now apparent that conjugation of terpenes with amino acids by the Ugi reaction is one of the Nature’s strategies to expand the diversity of natural products.
EXPERIMENTAL
U-5C-4-CR for the synthesis of despiro-exigurin 18. To a solution of sarcosine (10) (135 mg, 1.51 mmol) in MeOH (20.0 mL) at room temperature was added aqueous formaldehyde (37%, 0.10 mL, 1.33 mmol). After stirring at 50 °C for 20 min, terpene isocyanide 15 (100 mg, 0.61 mmol) was added. The solution was stirred at 50 °C for 20 min, and concentrated under reduced pressure. The resulting residue was treated with water, and the aqueous layers were extracted with AcOEt (× 3). The combined organic layers were dried (Na2SO4) and concentrated under reduced pressure. The crude residue (131 mg) was subjected to silica gel chromatography (AcOEt/hexane 1:1, containing 1% isopropylamine) to afford methyl ester 18 (72 mg, 40%) as a colorless oil: [α]D26 +22.3 (c 1.00, CHCl3); IR (NaCl) νmax 3473, 3352, 2950, 2922, 1747, 1679, 1517, 1457, 1199 cm-1; 1H NMR (CDCl3, 500 MHz) δ 0.86 (d, J = 7.0 Hz, 3H), 0.88 (d, J = 7.0 Hz, 3H), 0.89 (d, J = 7.0 Hz, 3H), 0.91–1.16 (m, 6H), 1.22–1.35 (m, 1H), 1.38–1.52 (m, 1H), 1.75 (dt, J = 13.0, 3.0 Hz, 1H), 1.82–1.88 (m, 2H), 2.42 (s, 3H), 3.16 (s, 2H), 3.32 (s, 2H), 3.71 (s, 3H), 4.32 (dd, J = 9.0, 3.0 Hz, 1H), 7.56 (brd, J = 9.0 Hz, 1H); 1H NMR (C6D6, 500 MHz) δ 0.72–0.92 (m, 2H), 0.98 (dt, J = 13.0, 3.0 Hz, 1H), 1.06 (d, J = 7.0 Hz, 3H), 1.18 (ddt, J = 17.0, 8.0, 3.0 Hz, 1H), 1.40–1.52 (m, 1H), 1.53–1.68 (m, 2H), 1.72 (brd, J = 13.0 Hz, 1H), 1.98–2.09 (m, 4H), 2.80 (d, J = 17.0 Hz, 1H), 2.82 (d, J = 17.0 Hz, 1H), 2.98 (d, J = 17.0 Hz, 1H), 3.01 (d, J = 14.0 Hz, 1H), 3.23 (s, 3H), 4.65 (dq, J = 13.0, 7.0 Hz, 1H), 7.59 (brd, J = 7.0 Hz, 1H); 13C NMR (CDCl3, 125 MHz) δ 20.6, 20.9, 22.2, 25.2, 26.7, 29.5, 34.7, 40.1, 43.1, 45.4, 46.2, 51.5, 58.4, 60.9, 169.1, 170.8; 13C NMR (C6D6, 125 MHz) δ 21.0, 21.3, 22.6, 25.7, 27.1, 30.0, 35.3, 40.9, 43.0, 45.6, 46.8, 51.2, 58.3, 61.5, 168.5, 170.9. HRMS(ESI): m/z calcd for C16H30N2O3Na [M+Na]+ 321.2154, found 321.2155.
U-4C-3CR for the synthesis of despiro-boneratamide A 28. To a solution of L-glutamic acid 1-methyl ester (23) (219 mg, 1.36 mmol) and acetone (0.70 mL, 9.1 mmol) in MeOH (15.0 mL) was added isocyanide 27 (150 mg, 0.91 mmol). After stirring at room temperature for 1.5 h, the solution was heated at 50 °C for 3 days, followed by refluxing for 2 days. The reaction mixture was concentrated under reduced pressure, and the residue was dissolved in AcOEt and water. The organic layer was separated, and the aqueous layer was extracted with AcOEt. The combined organic extracts were dried (Na2SO4) and concentrated to afford a residue, which was subjected to silica gel chromatography (AcOEt/hexane 1:1 to 4:1) to furnish γ-lactam 28 (222 mg, 67%): mp 139–140 °C (recrystallized from hexane); [α]D25 –24.9 (c 1.00, CHCl3); IR (KBr) νmax 3370, 2950, 1731, 1664, 1528, 1221 cm-1; 1H NMR (CDCl3, 500 MHz) δ 0.82–1.90 (m, 26H), 2.09 (m, 1H), 2.20 (dq, J = 13.2, 2.9 Hz, 1H ), {2.37 (m)}, 3.15 (d, J = 13.2 Hz, 3H), 3.74 (ddd, J = 20.0, 9.7, 1.7 Hz, 1H), 4.71 (m, 1H), 7.50 (d, J = 9.2 Hz, 1H), {7.60 (d, J = 8.0 Hz)}; 13C NMR (C6D6, 125 MHz) δ 21.1, (21.4), 21.6, (21.7), 22.9, (22.9), 23.4, (23.6), (24.4), 24.6, 25.3, (25.5), (25.8), 25.9, (27.1), 27.3, 29.8, (29.8), (30.0), 30.2, 35.6, (35.6), 40.5, (40.6), 46.4, (46.6), 46.8, (47.0), (52.36), 52.45, 57.4, (57.8), 60.3, (60.5), (172.2), 172.4, 173.7, (174.2), (175.6), 176.1. HRMS(ESI): m/z calcd for C20H34N2O4Na [M+Na]+ 389.2416, found 389.2401; HRMS(ESI): m/z calcd for C20H34N2O4K [M+K]+ 405.2156, found 405.2155.
References
1. To the best of our knowledge, exigurin is the first literature—emerged example of secondary metabolites produced through the Ugi reaction.a) M. M. Uy, S. Ohta, M. Yanai, E. Ohta, T. Hirata, and S. Ikegami, Tetrahedron, 2003, 59, 731; b) E. Avilés and A. D. Rodríguez, Org. Lett., 2010, 12, 5290; c) S. Suto, N. Tanaka, J. Fromont, and J. Kobayashi, Tetrahedron Lett., 2011, 52, 3470; CrossRef d) N. Tanaka, S. Suto, H. Ishiyama, T. Kubota, A. Yamano, M. Shiro, J. Fromont, and J. Kobayashi, Org. Lett., 2012, 14, 3498; CrossRef e) N. Tanaka, S. Suto, M. Asai, T. Kusama, A. Takahashi-Nakaguchi, T. Gonoi, J. Fromont, and J. Kobayashi, Heterocycles, 2015, 90, 173. CrossRef
2. T. Okino, E. Yoshimura, H. Hirota, and N. Fusetani, Tetrahedron, 1996, 52, 9447. CrossRef
3. M. J. Garson and J. S. Simpson, Nat. Prod. Rep., 2004, 21, 164. CrossRef
4. D. E. Williams, B. O. Patrick, A. Tahir, R. Van Soest, M. Roberge, and R. J. Andersen, J. Nat. Prod., 2004, 67, 1752. CrossRef
5. K. Saito, A. Nishimori, H. Kotsuki, K. Nakano, and Y. Ichikawa, Synlett, 2013, 24, 757. CrossRef
6. a) I. Ugi, Angew. Chem., Int. Ed. Engl., 1962, 1, 8; CrossRef b) I. Ugi, Angew. Chem., Int. Ed. Engl., 1982, 21, 810. CrossRef
7. I. Ugi and C. Steinbrückner, Chem. Ber., 1961, 94, 2802. CrossRef
8. C. Hulme and J. Dietrich, Mol. Divers., 2009, 13, 195. CrossRef
9. a) A. Demharter, W. Hörl, E. Herdtweck, and I. Ugi, Angew. Chem., Int. Ed. Engl., 1996, 35, 173; CrossRef b) I. Ugi, A. Demharter, W. Hörl, and T. Schmid, Tetrahedron, 1996, 52, 11657. CrossRef
10. a) A. Dömling and I. Ugi, Angew. Chem. Int. Ed., 2000, 39, 3168; CrossRef b) A. Dömling, Chem. Rev., 2006, 106, 17. CrossRef
11. S. Kłossowski, B. Wiraszka, S. Berłożecki, and R. Ostaszewski, Org. Lett., 2013, 15, 566. CrossRef
12. a) K. Saito, A. Nishimori, R. Mimura, K. Nakano, H. Kotsuki, T. Masuda, and Y. Ichikawa, Eur. J. Org. Chem., 2013, 2013, 7041; b) R. Mimura, A. Kitamori, A. Ikeda, T. Masuda, K. Nakano, H. Kotsuki, and Y. Ichikawa, Synthesis, 2015, 47, 3043. CrossRef
13. B. Di Blasio, E. Fattorusso, S. Magno, L. Mayol, C. Pedone, C. Santacroce, and D. Sica, Tetrahedron, 1976, 32, 473. CrossRef
14. This terpene isocyanide 27 is an enantiomer of 15. For the synthesis of 27, see the SI.
15. This dogma is taken from the reference; B. Lowry, C. T. Walsh, and C. Khosla, Synlett, 2015, 26, 1008