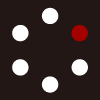
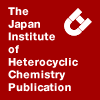
HETEROCYCLES
An International Journal for Reviews and Communications in Heterocyclic ChemistryWeb Edition ISSN: 1881-0942
Published online by The Japan Institute of Heterocyclic Chemistry
e-Journal
Full Text HTML
Received, 4th March, 2016, Accepted, 25th March, 2016, Published online, 5th April, 2016.
■ Concise Synthesis and Biological Evaluation of Chalcone Derivatives Bearing N-Heterocyclic Moieties
Zewei Mao, Xi Zheng, Yuping Lin, Yan Qi, Chunyan Hu, Chunping Wan,* and Gaoxiong Rao*
Central laboratory, The NO.1 Affiliated Hospital of Yunnan University of Traditional Chinese Medicine, Kunming, 650021, China
Abstract
In this study, we designed and synthesized a series of chalcone derivatives bearing N- heterocyclic moieties, and screened in vitro anti- inflammatory in lipopolysaccharide (LPS)-stimulated RAW-264.7 macrophages and anticancer activity against a panel of human tumor cell lines. The results indicated that compound 9 not only had inhibitory effect on the generation of NO (IC50=8.11μM) and significantly inhibited the production of TNF-α, but also showed better anticancer activity against Hela and SGC7901 (IC50=1.05μM and 6.47μM, respectively), which was identified as the most potent anti-inflammatory and anticancer agent.The secretion of inflammatory mediators by macrophages is involved in both the innate and adaptive immune responses of many human diseases, such as infection and rheumatoid arthritis (RA).1 LPS, a component of the cell wall of gram-negative bacteria, is known to activate a number of cellular signals in macrophages. The macrophage produce many chemokines (NO, PGE2) and pro-inflammatory cytokines (TNF-α, IL-6, IL-1β) when the macrophages are stimulated by LPS. So, the pro-inflammatory mediator is considered as potential target in inflammatory-associated diseases. On the other hand, cancer is the second most common cause of death in the world, which still remains a potentially life threatening disease resulting from uncontrolled cell growth.
Chalcones play an important role in living organisms2 with a wide range of biological activities, which are widely used as anti-inflammatory, antibacterial, antitumor and so on.3-6 Moreover, chalcones are widely present in nature, and a number of anti-inflammatory and anticancer chalcones have been reported in recent years, including sappanchalcone,7 butein,8 licochalcone A9 and echinatin.10 Due to their flexible character bearing α, β-unsaturated ketone unit, chalcones are playing the vital role in drug research through structural modification.11-14
Nitrogen heterocycles are important class of compounds having versatile biological activities, which are used in drug design and synthesis generally as active units. The combination of the chalcone and imidazole in one frame, has been reported in previous literature and is likely to lead to hybrid compounds with potential activity.15 Moreover, in previous work, we have reported the synthesis of chalcone-piperazine hybrid compounds and their potential anticancer activities.16 On the basis of these results, we wanted to design and synthesize molecules towards the recombination of chalcone and active nitrogen heterocycle moieties in the same structure through medicinal chemistry hybridization. Here, we reported the concise synthesis of chalcone derivatives containing nitrogen heterocycle compound, and screened the in vitro anti-inflammatory potential of compounds in LPS-stimulated inflammatory response in RAW-264.7 macrophages and anticancer activity against a panel of human tumor cell lines by MTT assay.
The general concise synthetic route used to obtain title compounds is outlined in Scheme 1. Treatment of commercially available 4-dimethylaminobenzaldehyde with 4-fluoroacetophenone gave the 4- dimethylamino-4’-fluorochalcone (1)17 in the presence of KOH resulted by the Aldol condensation, and it was in trans-configuration. The desired compounds were formed from chalcone intermediate by subsequent substitution reaction with different active nitrogen heterocycle compounds in the presence of Cs2CO3 at 110 °C in DMF in good yields (2-16) following the literature procedure, and no aza-Michael addition product was observed.18,19
Initially, K2CO3 was used in synthesis of title compounds. The results showed that compounds 8-16 were obtained in satisfied yields, but compounds 2-7 were in low yields. Replaced by Cs2CO3, chalcone derivatives 2-16 were in excellent yields,since the basicity of imidazole and benzimidazole was less than that of other nitrogen active compounds.
Comparative data for these compounds with respective to structures and yield are provided in Table 1. All of the synthesized compounds were characterized by 1H NMR and 13C NMR, and some representative compounds were characterized by HRMS analysis.
Anti-inflammatory activity: RAW 264.7 cell, a murine macrophage cell line, is widely used to establish inflammatory model in vitro. In this study, we investigated the anti-inflammatory activity of synthetic derivatives in LPS-induced RAW 264.7 on the generation of NO and pro-inflammatory cytokines.
To evaluate the effect of chalcone analogs (2-16) on NO generation, the RAW 264.7 cells were stimulated with LPS for 24 h, the level of NO from cell culture supernatant was measured by Griess reagent. The results indicated that compounds 8 and 9 showed significant inhibitory effect on the generation of NO in LPS-induced RAW 264.7, the IC50 value was calculated to be about 10.13 μM and 8.11 μM respectively (Table 2), neither had showed obvious cell cytotoxicity to RAW 264.7 cells at the concentration of 100 μM.
Stimulated macrophages have great impact on inflammatory diseases via excess production of cytokines, including tumor necrosis factor-α (TNF-α), interleukin-1β (IL-1β), IL-6, and other inflammatory mediators such as nitric oxide (NO). In order to detect the effect of compound on pro-inflammatory cytokines (TNF-α, IL-6), we then selected the compound 9 for further study. The production of TNF-α in LPS-induced RAW 264.7 was significantly down-regulated after exposed to indicate concentration of compound 9. However, interestingly, it had no apparent effects on the generation of IL-6 (Figure. 1). At present, our results indicated that compound 9 significantly inhibited the production of TNF-α and NO in LPS-induced RAW264.7.
Anticancer activity: Cytotoxic activity of novel synthesized hybrid derivatives were evaluated against human lung cancer cell line A549, human cervical carcinoma Hela and human gastric carcinoma SGC7901 by MTT assay, cisplatin (DDP) was used as reference drug. The biological results of hybrid compounds are summarized in Tables 3.
As shown in Table 3, the N-heterocycle moieties of the hybrid compounds have an obvious influence on the antitumor activities. To our delight, compounds bearing imidazole, triazole, piperazine or 3-hydroxyazetidine displayed potent or similar cytotoxic activity against human tumor cell lines compared to DDP. Compound 2 displayed potent anticancer activity against A549 (10.21 μM) and Hela (7.42 μM), compound 8 appeared good cytotoxic activity against SGC7901 (9.53 μM), and compound 11 showed potent anticancer activity against Hela (6.58 μM). Especially, among all derivatives, compound 9 showed the best IC50 value against Hela (1.05 μM) and SGC7901 (6.47 μM), which was identified as the most potent anticancer agent. However, substitution at the N-heterocycle with other substituted groups resulted in lower activity. For example, compounds 3-4 and 10-11 were less active than compounds 2 and 9, respectively.
Finally, we discussed a clear tendency of structure-activity relationship: Comparison of the activities of the chalcone derivatives 8 and 9 revealed that compounds bearing piperazine or triazole moiety showed significant anti-inflammatory activity, and compounds 2, 8, 9, 11 and 14 bearing imidazole, triazole, piperazine or 3-hydroxyazetidine moiety displayed potent antitumor activity. Interestingly, both anti-inflammatory and anticancer activity would be decreased when substitution at the N-heterocycle moiety with other groups except pyrimidyl. Further derivatization and biological research is under progress in our laboratory and will be reported in due course.
EXPERIMENTAL
Starting materials were commercially available and analytically pure. Melting points were measured on YANACO microscopic melting point meter (Yanaco, Japan) and were uncorrected. 1H NMR and 13C NMR spectras were recorded on a Bruker AV 300 spectrometer (Bruker Company, Germany), using TMS as internal standard and CDCl3 as solvent, respectively. TLC analysis was carried out on silica gel plates GF254 (Qindao Haiyang Chemical, China). High-resolution mass spectra were performed on an ESI Q-TOF MS spectrometer (Micromass, England).
Synthesis of 4-Dimethylamino-4’-fluorochalcone (1): To a solution of EtOH (15 mL), 4-dimethyl- aminobenzaldehyde (1.49 g, 10 mmol) and 4-fluoroacetophenone (1.38 g, 10 mmol), was added 20% KOH (10mL) and left to react for 16 h at rt. The reaction was quenched by the addition of water (30 mL) and was extracted with CHCl3 (3×20 mL). The organic layer was dried using anhydrous sodium sulfate, concentrated in vacuo and purified by column chromatography (10% EtOAc/PE, 2.35g, 87% yield). Yellow solid; mp 139-141C; 1HNMR (300 MHz, CDCl3) δ: 8.01-8.05 (m, 2H), 7.81 (d, J=15.3 Hz, 1H), 7.55 (d, J=8.7 Hz, 2H), 7.32 (d, J=15.2 Hz, 1H), 7.11-7.17 (m, 2H), 6.69 (d, J=8.7Hz, 2H), 3.03 (s, 6H); 13C NMR (75 MHz,CDCl3) δ: 188.9, 166.9, 163.6, 152.1, 146.0, 135.4, 130.9, 130.7, 130.5, 122.5, 116.4, 115.6, 115.3, 111.8, 40.1.
General procedure for the preparation of chalcone derivatives 2-16. To a stirred solution of the 4-dimethylamino-4’-fluorochalcone 1 (1.0 mmol) and Cs2CO3 (2.0 mmol) in dried DMF (5 mL), nitrogen heterocycle (1.5 mmol) was added and reaction mixture was stirred for 12-15 h at 110 °C. After completion of the reaction as indicated by TLC, The reaction was quenched by the addition of CHCl3 (20 mL) and was washed with water (3×20 mL). The organic layer was dried using anhydrous sodium sulfate, concentrated in vacuo and purified by column chromatography to afford 2-16 in 55-91% yields.
4-Dimethylamino-4’-(1-imidazolyl)chalcone (2). Yellow solid; 1H NMR (300 MHz, CDCl3) δ: 8.14 (d, J=8.7 Hz, 2H), 7.95 (s, 1H), 7.85 (d, J=15.3 Hz, 1H), 7.57 (d, J=8.7 Hz, 2H), 7.51 (d, J=8.4 Hz, 2H), 7.36 (d, J=4.5 Hz, 2H), 7.24 (s, 1H), 6.71 (d, J=8.7 Hz, 2H), 3.05 (s, 6H); 13C NMR (75 MHz, CDCl3) δ: 188.8, 152.3, 146.5, 140.0, 137.9, 135.4, 131.0, 130.6, 130.2, 122.4, 120.7, 117.8, 116.1, 111.8, 40.1; HRMS: m/z calcd for C20H20N3O (M+H)+ 318.1601, found 318.1601.
4-Dimethylamino-4’-(2-methyl-1-imidazolyl)chalcone (3). Yellow solid; 1H NMR (300 MHz, CDCl3) δ: 8.11 (d, J=5.7 Hz, 2H), 7.90 (d, J=8.4 Hz, 1H), 7.55 (d, J=8.4 Hz, 2H), 7.34-7.47 (m, 2H), 7.04 (s, 2H), 6.62-6.71 (m, 3H), 3.01 (s, 6H), 2.40 (s, 3H); 13C NMR (75 MHz, CDCl3) δ: 186.8, 158.5, 153.8, 152.2, 144.8, 141.3, 138.3, 131.2, 130.9, 129.5, 128.4, 125.4, 125.3, 125.0, 122.5, 120.6, 113.7, 112.1, 111.2, 40.3, 14.3.
4-Dimethylamino-4’-(2-ethyl-1-imidazolyl)chalcone (4). Brown solid; 1H NMR (300 MHz, CDCl3) δ: 8.13 (d, J=5.1 Hz, 2H), 7.87 (d, J=15.6 Hz, 1H), 7.58 (d, J=9.0 Hz, 2H), 7.28-7.42 (m, 3H), 7.10 (d, J=15.6 Hz, 2H), 6.71 (d, J=9.0 Hz, 2H), 3.05 (s, 6H), 2.76 (q, J=7.5 Hz, 2H), 1.30 (t, J=7.5 Hz, 3H); 13C NMR (75 MHz, CDCl3) δ: 189.2, 152.3, 149.5, 146.7, 140.8, 138.7, 130.7, 129.6, 128.0, 125.5, 122.3, 120.4, 116.1, 111.8, 40.1, 20.7, 12.4.
4-Dimethylamino-4’-(1-benzimidazolyl)chalcone (5). Pale yellow solid; 1H NMR (300 MHz, CDCl3) δ: 8.19 (d, J=8.4 Hz, 2H), 8.15 (s, 1H), 7.86-7.90 (m, 1H), 7.81 (s, 1H), 7.51 - 7.60 (m, 5H), 7.31-7.36 (m, 3H), 6.66 (d, J=9.0 Hz, 2H), 2.99 (s, 6H); 13C NMR (75 MHz, CDCl3) δ: 188.7, 152.2, 146.5, 144.3, 142.0, 139.3, 138.2, 133.2, 130.6, 130.2, 124.0, 123.2, 123.1, 122.3, 120.7, 115.9, 111.8, 110.6, 40.0.
4-Dimethylamino-4’-(2-methyl-1-benzimidazolyl)chalcone (6). Yellow solid; 1H NMR (300 MHz, CDCl3) δ: 8.24 (d, J=8.4 Hz, 2H), 7.91 (d, J=15.6 Hz, 1H), 7.78 (d, J=7.8 Hz, 1H), 7.61 (d, J=9.0 Hz, 2H), 7.53 (d, J=8.7 Hz, 2H), 7.49 (d, J=15.6 Hz, 1H), 7.20-7.30 (m, 3H), 6.73 (d, J=8.7 Hz, 2H), 3.07 (s, 6H), 2.57 (s, 3H); 13C NMR (75 MHz, CDCl3) δ: 189.3, 152.3, 146.8, 142.7, 139.3, 130.7, 130.0, 126.9, 122.9, 122.7, 119.2, 116.1, 111.8, 109.9, 40.1, 14.6.
4-Dimethylamino-4’-(5, 6-dimethyl-1-benzimidazolyl)chalcone (7). Brown solid; 1H NMR (300 MHz, CDCl3) δ: 8.22 (d, J=8.4 Hz, 2H), 8.07 (s, 1H), 7.88 (d, J=15.6 Hz, 1H), 7.55-7.64 (m, 5H), 7.39 (s, 2H), 6.71 (d, J=8.7 Hz, 2H), 3.04 (s, 6H), 2.40 (s, 6H); 13C NMR (75 MHz, CDCl3) δ: 189.0, 152.2, 146.6, 142.9, 141.2, 139.7, 138.0, 133.3, 132.1, 131.7, 130.7, 130.2, 123.1, 122.4, 120.7, 116.0, 111.8, 110.7, 40.1, 20.7, 20.3.
4-Dimethylamino-4’-(1-triazolyl)chalcone (8). yellow solid; 1H NMR (300MHz, CDCl3) δ: 8.67 (s, 1H), 8.17 (s, 1H), 8.14 (s, 2H), 7.85 (d, J=15.6 Hz, 1H), 7.84 (d, J=8.7 Hz, 2H), 7.57 (d, J=8.7 Hz, 2H), 7.35 (d, J=15.6 Hz, 1H), 6.71 (d, J=8.7 Hz, 2H), 3.05 (s, 6H); 13C NMR (75 MHz, CDCl3) : 188.8, 152.9, 152.3, 146.6, 141.0, 139.4, 138.5, 130.6, 130.1, 122.4, 119.4, 116.1, 111.8, 40.1; HRMS: m/z calcd for C19H19N4O (M+H)+ 319.1553, found 319.1554.
4-Dimethylamino-4’-(1-piperazinyl)chalcone (9). brown solid; 1H NMR (300 MHz, CDCl3) δ: 8.01 (d, J=9.0 Hz, 2H), 7.80 (d, J=15.3 Hz, 1H), 7.56 (d, J=9.0 Hz, 2H), 7.41 (d, J=15.6 Hz, 1H), 6.92 (d, J=9.0 Hz, 2H), 6.70 (d, J=9.0 Hz, 2H), 3.33 (t, J=4.8 Hz, 4H), 3.01-3.04 (m, 10H), 2.12 (s, 1H); 13C NMR (75 MHz, CDCl3) : 188.3, 154.2, 151.8, 144.1, 130.3, 130.1, 129.1, 123.0, 116.8, 113.6, 111.8, 48.5, 45.9, 40.2; HRMS: m/z calcd for C21H26N3O (M+H)+ 336.2070, found 336.2072.
4-Dimethylamino-4’-(N-methyl-1-piperazinyl)chalcone (10). Brown solid; 1H NMR (300 MHz, CDCl3 ) δ: 8.00 (d, J=9.0 Hz, 2H), 7.80 (d, J=15.6 Hz, 1H), 7.55 (d, J=8.7 Hz, 2H), 7.39 (d, J=15.6 Hz, 1H), 6.92 (d, J=8.7 Hz, 2H), 6.70 (d, J=9.0 Hz, 2H), 3.38 (t, J=5.1 Hz, 4H), 3.01 (s, 6H), 2.57 (t, J=5.1 Hz, 4H), 2.34 (s, 3H); 13C NMR (75 MHz, CDCl3) : 188.3, 153.8, 151.8, 144.1, 130.3, 130.1, 129.2, 123.1, 116.9, 113.7, 111.9, 54.8, 47.3, 46.1, 40.1.
4-Dimethylamino-4’-(N-(2-pyrimidyl)-1-Piperazinyl)chalcone (11). Yellow solid; 1H NMR (300 MHz, CDCl3 ) δ: 8.35 (d, J=4.8 Hz, 2H), 8.03 (d, J=8.7 Hz, 2H), 7.81 (d, J=15.6 Hz, 1H), 7.56 (d, J=8.7 Hz, 2H), 7.40 (d, J=15.3 Hz, 1H), 6.96 (d, J=9.0 Hz, 2H), 6.70 (d, J=9.0 Hz, 2H), 6.55 (t, J=4.8 Hz, 1H), 4.01 (t, J=5.4 Hz, 4H), 3.47 (t, J=5.4 Hz, 4H), 3.02 (s, 6H); 13C NMR (75 MHz, CDCl3) : 188.4, 161.6, 157.8, 153.7, 151.8, 144.2, 130.4, 130.1, 129.4, 123.1, 116.9, 113.8, 111.9, 110.3, 47.3, 43.3, 40.1.
4-Dimethylamino-4’-(1-pyrazolyl)chalcone (12). Yellow solid; 1H NMR (300 MHz, CDCl3 ) δ: 8.14 (d, J=8.7 Hz, 2H), 8.02 (d, J=2.1 Hz, 1H), 7.77-7.85 (m, 4H), 7.58 (d, J=8.7 Hz, 2H), 7.38 (d, J=15.3 Hz, 1H), 6.70 (d, J=8.7 Hz, 2H), 6.52 (t, J=1.8 Hz, 1H), 3.04 (s, 6H); 13C NMR (75 MHz, CDCl3) : 189.0, 152.1, 146.0, 142.7, 141.9, 136.7, 130.5, 129.9, 126.9, 122.5, 118.4, 116.3, 111.8, 108.4, 40.1; HRMS: m/z calcd for C20H20N3O (M+H)+ 318.1601, found 318.1603.
4-Dimethylamino-4’-(3,5-dimethyl-1-pyrazolyl)chalcone (13). Yellow solid; 1H NMR (300 MHz, CDCl3 ) δ: 8.11 (d, J=8.7 Hz, 2H), 7.84 (d, J=15.6 Hz, 1H), 7.59 (d, J=6.6 Hz, 2H),, 7.57 (d, J=7.2 Hz, 2H), 7.36 (d, J=15.6 Hz, 1H), 6.71 (d, J=8.7 Hz, 2H), 6.04 (s, 1H), 3.04 (s, 6H), 2.38 (s, 3H), 2.32 (s, 3H); 13C NMR (75 MHz, CDCl3) : 189.6, 152.1, 149.8, 146.3, 142.9, 139.6, 137.2, 130.5, 129.3, 123.8, 122.5, 116.6, 111.8, 108.1, 40.1, 13.6, 12.8.
4-Dimethylamino-4’-(3-hydroxyazetidin-1-yl)chalcone (14). Pale yellow solid; 1H NMR (300 MHz, CDCl3 ) δ: 7.94 (d, J=8.7 Hz, 2H), 7.78 (d, J=15.3 Hz, 1H), 7.54 (d, J=8.7 Hz, 2H), 7.38 (d, J=15.3 Hz, 1H), 6.70 (d, J=8.7 Hz, 2H), 6.40 (d, J=8.7 Hz, 2H), 4.77-4.81 (m, 1H), 4.27 (t, J=8.1 Hz, 2H), 3.79-3.83 (dd, J=4.5 Hz, 4.5Hz, 2H), 3.03 (s, 6H); HRMS: m/z calcd for C20H23N2O2 (M+H)+ 323.1754, found 323.1750.
4-Dimethylamino-4’-(1-pyrrolyl)chalcone (15). Yellow solid; 1H NMR (300 MHz, CDCl3 ) δ: 8.00 (d, J=9.0 Hz, 2H), 7.79 (d, J=15.3 Hz, 1H), 7.54 (d, J=8.7 Hz, 2H), 7.43 (d, J=15.3 Hz, 1H), 6.68 (d, J=9.0 Hz, 2H), 6.53 (d, J=8.7 Hz, 2H), 3.34 (t, J=6.9 Hz, 4H), 2.98 (s, 6H), 1.96-2.01 (m, 4H); 13C NMR (75 MHz, CDCl3) : 187.9, 151.6, 150.7, 143.2, 130.7, 130.0, 126.2, 123.4, 117.2, 111.9, 110.9, 47.6, 40.2, 25.4.
4-Dimethylamino-4’-(1-piperidyl)chalcone (16). Brown solid; 1H NMR (300 MHz, CDCl3 ) δ: 7.99 (d, J=9.0 Hz, 2H), 7.80 (d, J=15.6 Hz, 1H), 7.55 (d, J=8.7 Hz, 2H), 7.41 (d, J=15.6 Hz, 1H), 6.90 (d, J=8.7 Hz, 2H), 6.69 (d, J=8.7 Hz, 2H), 3.35 (s, 4H), 3.01 (s, 6H), 1.65 (s, 6H); 13C NMR (75 MHz, CDCl3) : 188.2, 154.2, 143.8, 130.5, 130.0, 128.2, 123.3, 117.0, 113.5, 111.9, 48.7, 40.2, 25.4, 24.4.
Antimicrobial Activity. Briefly, murine RAW264.7 macrophages (American Type Culture Collection, Manassas) were plated in 96 well plate at a density of 1× 105 cells/well and stimulated with 1μg/mL LPS in the present or absence of various concentration of compound for 24 h, the culture supernatant were harvested to measure NO and cytokines production. The production of NO was determined by assaying culture supernatant for NO2-, a stable reaction product of NO, 100 μL of supernatant was mixed with an equal volume of Griess reagent [1% sulfanilamide and 0.1% N-(1-naphthyl)ethylenediamine dihydrochloride in 2.5% H3PO4] at room temperature for 10 min. Absorbance was measured at 540 nm in a microplate reader. Nitrite concentration was calculated from a NaNO2 standard curve. For the cytokines detection, culture supernatants were harvested at 36 h to measure IL-6 and TNF-α level by ELISA following the manufacture’s instruction.
Antitumor activity: About 1×104 cell/well were seeded into 96-well microtiter plates. After 24 h post-seeding, cells were treated with vehicle control or various concentrations of samples for 48 h. 20 μL of MTT solution (5 mg/mL) was added to each well and the tumor cells were incubated at 37 °C in a humidified atmosphere of 5% CO2 air for 4 h. Upon removal of MTT/medium, 150 μL of DMSO was added to each well and the plate was agitated at oscillator for 5 min to dissolve the MTT-formazan. The assay plate was read at a wavelength of 570 nm using a microplate reader.
ACKNOWLEDGEMENTS
This work was financially supported by the Natural Science Foundation of China (81460624) and the Application Basic Research Program of Yunnan Province (2014FZ087).
References
1. W. L. Smith and L. J. Marnett, Biochim. Biophys. Acta, 1991, 1083, 1. CrossRef
2. F. Berrue, M. W. B. McCulloch, and R. G. Kerr, Bioorg. Med. Chem., 2011, 19, 6702. CrossRef
3. L. Jayasinghe, B. A. I. S. Balasooriya, W. C. Padmini, N. Hara, and Y. Fujimoto, Phytochemistry, 2004, 65, 1287. CrossRef
4. K. Sugamoto, Y. I. Matsusita, K. Matsui, C. Kurogi, and T. Matsui, Tetrahedron, 2011, 67, 5346. CrossRef
5. N. Mishra, P. Arora, B. Kumar, L. C. Mishra, A. Bhattacharya, S. K. Awasthi, and V. K. Bhasin, Eur. J. Med. Chem., 2008, 43, 1530. CrossRef
6. Z. Nowakowska, B. Kedzia, and G. Schroeder, Eur. J. Med. Chem., 2008, 43, 707. CrossRef
7. G. S. Jeong, D. S. Lee, B. Li, H. J. Lee, E. C. Kim, and Y. C. Kim, Eur. J. Pharmacol., 2010, 644, 1. CrossRef
8. Z. Wang, Y. Y. Lee, J. S. Eun, and E. J. Bae, Eur. J. Pharmacol., 2014, 738, 40. CrossRef
9. M. Chen, S. B. Christensen, J. Blom, E. Lemmich, L. Nadelmann, K. Fich, T. G. Theander, and A. Kharazmi, Antimicrob. Agents Chemother., 1993, 37, 2550. CrossRef
10. S. I. Ayabe and T. Furuya, Tetrahedron Lett., 1981, 22, 2097. CrossRef
11. J. Chen, J. Yan, J. H. Hu, Y. Q. Pang, L. Huang, and X.S. Li, RSC Adv., 2015, 5, 68128.
12. Y. N. Shen, L. Lin, H.Y. Qiu, W. Y. Zou, Y. Qian, and H. L. Zhu, RSC Adv., 2015, 5, 23767.
13. K. V. Sashidhara, M. Kumar, R. K. Modukuri, R. Sonkar, G. Bhatia, A. K. Khanna, S. Rai, and R. Shukla, Bioorg. Med. Chem. Lett., 2011, 21, 4480. CrossRef
14. C. Tang, L. H. Zhu, Y. Chen, R. Qin, Z. N. Mei, J. Xu, and G. Z. Yang, RSC Adv., 2014, 4, 10862.
15. V. K. Marrapu, V. Chaturvedi, S. Singh, S. Singh, S. Sinha, and K. Bhandari, Eur. J. Med. Chem., 2011, 46, 4302. CrossRef
16. Z. W. Mao, X. Zheng, Y. Qi, M. D. Zhang, Y. Huang, C. P. Wan, and G. X. Rao, RSC Adv., 2016, 6, 7723.
17. A. Kamal, A. Mallareddy, P. Suresh, V. L. Nayak, R. V. C. R. N. C. Shetti, N. S. Rao, J. R. Tamboli, T. B. Shaik, M. V. P. S. Vishnuvardhan, and S. Ramakrishna, Eur. J. Med. Chem., 2012, 47, 530. CrossRef
18. A. J. Watson and A. J. Fairbanks, Eur. J. Org. Chem., 2013, 30, 6784. CrossRef
19. M. L. Kantam, J. Yadav, S. Laha, B. Sreedhar, and S. Jha, Adv. Synth. Catal., 2007, 349, 1938 CrossRef