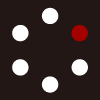
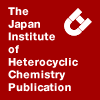
HETEROCYCLES
An International Journal for Reviews and Communications in Heterocyclic ChemistryWeb Edition ISSN: 1881-0942
Published online by The Japan Institute of Heterocyclic Chemistry
e-Journal
Full Text HTML
Received, 16th March, 2016, Accepted, 12th April, 2016, Published online, 27th April, 2016.
■ A One-Pot Synthesis of Phaitanthrin E Through Intermolecular Condensation/Intramolecular Aryl C-H Amination Cascade
Tomoki Itoh, Takumi Abe, Tominari Choshi, Takashi Nishiyama, and Minoru Ishikura*
School of Pharmaceutical Sciences, Health Sciences University of Hokkaido, Ishikari-Tobetsu, Hokkaido 061-0293, Japan
Abstract
A one-pot synthesis of phaitanthrin E starting from methyl indole-3-carboxylate and isatoic anhydride through intermolecular condensation/intramolecular aryl C-H amination cascade was developed.Alkaloids with an indolo[2,1-b]quinazoline core, represented by tryptanthrin (1), have attracted considerable biological and synthetic interest because of their intriguing structural features and wide range of promising biological activities.1 Many structural analogues of these alkaloids have been synthesized and some compounds have shown notable activity.2 In 2008, five new alkaloids, phaitanthrins A (2), B (3), C (4), D (5), and E (6), were isolated from Phaius mishmensis (Orchidaceae), and compound 2 showed moderate cytotoxicity against three human cancer cell lines.3 Total syntheses of 2, 3, and 4 were reported separately by two groups in 2013.4,5 In 2015, the first total synthesis of 5 and 6 was accomplished through a biogenetic pathway starting from anthranilic acid, o-aminophenylacetic acid, and glycolic acid, involving a one-pot transformation of a diamide intermediate through intramolecular dehydrative cyclization.6 We previously reported a one-pot synthesis of tryptanthrin (1) and candidine (7) by oxidative dimerization of indole-3-carboxaldehyde involving further conversion of 1 to 2 and 3, and we found that oxidative coupling of indole-3-carboxaldehyde with isatoic anhydride also produced 1 and 4.7 Moreover, 1 was synthesized through oxidative dimerization of skatole in a one-pot reaction.8 In continuing our studies, we envisioned that methyl indole-3-carboxylate (8) bearing the CO2Me group could serve as a useful building block for 6. In this paper, we describe one-pot access to 6 starting from methyl indole-3-carboxylate (8) and isatoic anhydride (9) through an intermolecular condensation/intramolecular aryl C-H amination cascade.
Initially, according to a previously reported protocol,7 the oxidative coupling reaction of 8 with 9 (1.5 equiv) using urea-hydrogen peroxide (UHP) (5 equiv) in toluene at 75 °C in air for 12 h was performed. This resulted in recovery of 8 (80%) along with trace amounts of amide 10 (8%). Ester 8 withstood the oxidative conditions for long reaction time, in contrast to the formation of 1 by the reaction of indole-3-carboxaldehyde with 9. Amide 10 was obtained in 72% yield by heating 8 with 9 in the presence of Et3N (2 equiv) in DMF at 130 °C for 16 h (Scheme 1).
We expected that intramolecular C-H amination of 10 would produce the indoloquinazoline core. Initially, 10 was oxidized with UHP (5 equiv) in toluene at 75 °C for 2 h, producing 1 in 65% yield. Considerable efforts have been made to develop metal-mediated C-H amination;9 therefore, we investigated whether a copper-mediated intramolecular aryl C-H amination in 10 would lead to 6. Heating 10 with CuI (1.5 equiv) in DMF at 130 °C for 16 h provided no products. However, to our surprise, we found that the addition of Et3N (2 equiv) promoted the reaction to produce 6 in 40% yield.
Therefore, we envisioned that coupling 8 with 9 in the presence of a copper complex could allow the one-pot formation of 6 involving the formation of amide 10 and intramolecular amination steps (Table 1). Although heating 8 and 9 with CuI (1.5 equiv) in DMF at 130 °C provided 10 (12%) and 1 (35%) without 6, performing the reaction in the presence of Et3N (2 equiv) produced 6 in 62% yield along with trace amounts of 10 (10%) (entries 1 and 2). The NMR data for 6 agreed well with the literature data.6 Hence, in a search for optimized conditions, other copper complexes were screened initially. However, the reaction did not occur with CuOAc, Cu(OAc)2, CuCl, CuCl2, and CuBr2, and only trace amounts of 6 was obtained with CuBr (entry 3). Reducing the amount of CuI resulted in a considerable decrease in the yield of 6 (entries 4 and 5), and increasing the amount to 3 equiv did not improve the yield (entry 6). Pyridine, i-Pr2NEt, and DABCO were less effective than Et3N (entries 7–9).
In summary, we have developed a one-pot synthesis of phaitanthrin E (6) using methyl indole-3-carboxylate (8) and isatoic anhydride (9) in the presence of CuI. The one-pot reaction proceeded through in situ generation of amide 10, followed by Cu-mediated intramolecular aryl C-H amination.
EXPERIMENTAL
Melting points were recorded with a Yamato MP21 and were uncorrected. High-resolution MS spectra were recorded with a JEOL JMS-T100LP mass spectrometer. IR spectra were measured with a Shimadzu IRAffinity-1 spectrometer. The NMR experiments were performed with a JEOL JNM-ECA500 (500 MHz) spectrometer, and chemical shifts are expressed in ppm (δ).
Methyl 1-[(2-aminophenyl)carbonyl]-1H-indole-3-carboxylate (10): Et3N (4 mmol) was added to a solution of 8 (355 mg, 2 mmol) and 9 (490 mg, 3 mmol) in DMF (20 mL), and the mixture was stirred at 130 °C for 16 h. After cooling to room temperature, the mixture was added to 10% aq. HCl, extracted with AcOEt (100 mL), washed with brine, and dried over MgSO4. The solvent was removed, and the residue was purified by silica gel column chromatography with CH2Cl2/AcOEt (50:1) to give 10 (431 mg, 73%) as a colorless solid. Mp 158–159 °C (CH2Cl2/hexane). IR (CHCl3): 3343, 1694, 1660 cm-1. 1H-NMR (CDCl3) δ: 3.91 (s, 3H), 5.27 (br s, 2H), 6.74 (td, J = 1.2, 6.9 Hz, 1H), 6.80 (d, J = 8.1 Hz, 1H), 7.33–7.37 (m, 2H), 7.38–7.42 (m, 2H), 8.05 (s, 1H), 8.19–8.21 (m, 2H). 13C-NMR (CDCl3) δ: 51.6, 112.5, 114.2, 115.8, 116.9, 117.3, 121.7, 124.7, 125.3, 127.7, 131.7, 134.0, 134.4, 136.5, 150.1, 164.7, 169.3. HR-MS (ESI) m/z: Calcd for C17H14N2NaO3 [(M + Na) +]: 317.0902. Found 317.0877.
Dakin oxidation of 10 using UHP: UHP (941 mg, 10 mmol) was added to a solution of 10 (588 mg, 2 mmol) in toluene (30 mL) at room temperature, and the mixture was heated at 75 °C. After 16 h, the mixture was gradually cooled to room temperature, 10% NaOH solution (4 mL) was added to the mixture, and stirred for 0.5 h. The mixture was diluted with AcOEt (100 mL) and washed with brine, and dried over MgSO4. The solvent was removed, and the residue was separated by silica gel column chromatography with CH2Cl2/AcOEt (50:1) to give 17 (322 mg, 65%).
Phaitanthrin E (6): After a mixture of CuI (1.05 g, 6 mmol) and Et3N (1.3 g, 8 mmol) in DMF (50 mL) was stirred at room temperature for 0.5 h, methyl indole-3-carboxylate (8) (701 mg, 4 mmol) and isatoic anhydride (9) (979 mg, 6 mmol) were added to the mixture and the mixture was stirred at 130 °C for 16 h. After cooling, 10% aq. HCl solution was added to the mixture, and the mixture was extracted with AcOEt (100 mL). The organic layer was washed with brine and dried over MgSO4. The solvent was removed, and the residue was separated by silica gel column chromatography with CH2Cl2 to give 6 (725 mg, 62%) as amorphous powder and 10 (10%). IR (CHCl3): 3329, 3020, 1697, 1665, 1626, 1579 cm-1. 1H-NMR (CDCl3) δ: 4.01 (s, 3H), 7.28 (d, J = 8.6 Hz, 1H), 7.30–7.34 (m, 2H), 7.43 (td, J = 1.2, 8.0 Hz, 1H), 7.71 (td, J = 1.7, 7.7 Hz, 1H), 7.94 (d, J = 8.0 Hz, 1H), 8.39 (d, J = 8.0 Hz, 1H), 8.70 (d, J = 8.0 Hz, 1H), 10.29 (br s 1H). 13C-NMR (CDCl3) δ: 51.4, 86.7, 114.4, 115.7, 116.3, 119.4, 122.4, 123.2, 125.7, 126.3, 128.7, 130.4, 135.3, 138.2, 144.1, 158.5, 167.3. HR-MS (ESI) m/z: Calcd for C17H13N2O3 [(M + H)+] 293.0926. Found 293.0926.
ACKNOWLEDGEMENTS
This work was supported in part by the Ministry of Education, Culture, Sports, Science and Technology of Japan through a Grant-in Aid for Scientific Research (No. 26460012), and the Akiyama Foundation.
References
1. A. M. Tucker and P. Grundt, ARKIVOC, 2012, i, 546; Y. Jahng, Arch. Pharm. Res., 2013, 36, 517; CrossRef U. A. Kshirsagar, Org. Biomol. Chem., 2015, 13, 9336. CrossRef
2. B. Krivogorsky, A. C. Nelson, K. A. Douglas, and P. Grundt, Bioorg. Med. Chem. Lett., 2013, 23, 1032; CrossRef J. M. Hwang, T. Oh, T. Kaneko, A. M. Upton, S. G. Franzblau, Z. Ma, S. N. Cho, and P. Kim, J. Nat. Prod., 2013, 76, 354; CrossRef K. Seya, A. Yamaya, S. Kamachi, M. Murakami, H. Kitahara, J. Kawakami, K. Okumura, M. Murakami, S. Motoura, and K.-I. Furukawa, J. Nat. Prod., 2014, 77, 1413. CrossRef
3. C.-W. Jao, W.-C. Lin, Y.-T. Wu, and P.-L. Wu, J. Nat. Prod., 2008, 71, 1275. CrossRef
4. S. D. Vaidya and N. P. Argade, Org. Lett., 2013, 15, 4006. CrossRef
5. G. Kang, Z. Luo, C. Liu, H. Gao, Q. Wu, H. Wu, and J. Jiang, Org. Lett., 2013, 15, 4738. CrossRef
6. S. D. Vaidya and N. P. Argade, Org. Lett., 2015, 17, 6218. CrossRef
7. T. Abe, T. Itoh, T. Choshi, S. Hibino, and M. Ishikura, Tetrahedron Lett., 2014, 55, 5268. CrossRef
8. T. Itoh, T. Abe, S. Nakamura, and M. Ishikura, Heterocycles, 2015, 91, 1423. CrossRef
9. For recent reviews for aryl C-H amination, see K. Okano, H. Tokuyama, and T. Fukuyama, Chem. Commun., 2014, 50, 13650; CrossRef M. Moselage, J. Li, and L. Ackermann, ACS Catal., 2016, 6, 498; CrossRef J. Jiao, K. Murakami, and K. Itami, ACS Catal., 2016, 6, 610 CrossRef