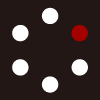
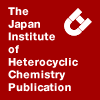
HETEROCYCLES
An International Journal for Reviews and Communications in Heterocyclic ChemistryWeb Edition ISSN: 1881-0942
Published online by The Japan Institute of Heterocyclic Chemistry
e-Journal
Full Text HTML
Received, 9th June, 2016, Accepted, 4th August, 2016, Published online, 22nd August, 2016.
DOI: 10.3987/COM-16-13516
■ Microwave-Assisted Efficient Synthesis of 4-Substituted Amino-2-methylquinolines Catalyzed by p-Toluenesulfonic Acid
Xiao-qin Wang,* Yuan-hong Cai, Xiao-yang Xie, Cui-ying Huang, Jia-yu Li, Wen-na Chen, Ming-hua He, and Wen-jia Pan*
School of Pharmacy, Guangdong Medical University, NO1, Xincheng Road, Songshan Lake, Dongguan 523808, China
Abstract
A series of novel 4-subtituted amino-2-methylquinolines (3a-3o) were readily synthesized via the reaction of 4-chloro-2-methylquinoline with amines catalyzed by p-toluenesulfonic acid (TsOH) at 120 ℃ for 1 h under microwave-assisted organic synthesis (MAOS) condition. The yields of product 3a-3o were in range of 55-89%. This approach has advantages such as higher yield, shorter reaction time, lower costs, more convenience, and higher efficiency compared to the conventional method. The structures of the products were characterized by using 1H NMR, 13C NMR and HRMS. The reactivity of different amines was discussed.4-Aminoquinoline derivatives represent an important class of compounds in organic chemistry and medicinal chemistry. In recent years, they have attracted a great deal of attention because they can be used as agents or intermediates for pharmaceuticals, agrochemicals, dyes, and luminescent materials in the chemical industry.1,2
Given the significance of these compounds, great efforts have been made to synthesize 4-aminoquinoline derivatives. In 1983, it was firstly reported that the reaction of aryl bromides with tributyltin amides catalyzed by palladium complexes gave the arylamine.3 Later, in 1995, Buchwald and Hartwig independently developed the methodology of tin-free amination of aryl halides.4,5 Based on their work, in the last two decades, transition-metal-catalyzed aminations of aryl halides have become the most popular tool for the production of arylamine,6-8 these catalysts include the complexes of palladium, nickel, and copper chelated with specifically designed ligands. In addition, Castello et al. reported that phenol was used to form a phenolic intermediate, and amine was added and heated at 125 °C for 5 h to afford arylamine.9,10 Moreover, 4-aminoquinolines were also synthesized by reacting 4-chloroquinoline with the corresponding amide solvents at reflux for overnight.11
Although all these achievements are desired to improve the efficiency of the preparation of 4-aminoquinolines, these traditional methods often suffer from one or more drawbacks, e.g. metal ligands are expensive and air sensitive, the reaction conditions are harsh, the scope of the reaction substrate is limited, environmental pollution is serious, the multistep synthesis is not straightforward and the overall yield is lower. Thus, the development of an inexpensive, mild, broadly applicable, convenient, and robust method to generate 4-aminoquinoline derivatives is still desirable and challenging.12
In recent years, microwave-assisted organic synthesis (MAOS) is a relatively new technique because of shorter reaction time, producing higher yields and increasing product purities, and it has been extensively used in organic chemistry synthesis.13 In our research on developing 2-arylethenylquinoline derivatives as multifunctional agents for the treatment of Alzheimer’s disease (AD), we previously reported the reaction of 4-chloro-2-methylquinoline with alicyclic amine (N-methylpiperazine, piperidine) in the presence of TsOH at 150 C in DMF for 12 h to afford the 4-amino-2-methylquinoline,14 but this method needs long reaction time and high temperature. Therefore, in this report, we planned to develop a convenient, rapid, and environment-friendly amination methodology using TsOH to prepare a series of 4-amino-2-methylquinolines by microwave-assisted organic synthesis (MAOS), which could overcome some shortcomings of the general synthetic method.
It was reported that 4-amino-2-methylquinolines were prepared by the reaction of 4-chloro-2-methylquinoline with amines in DMF at 150 C for 15 h with good yields.14 However, the yields of these compounds were found to be unsatisfactory in our repeated experiment. Moreover, this reaction occured at high temperature and for long time. Therefore, the reaction conditions need to be optimized. At first, the synthetic methods of 4-(N-methylpiperazin-1-yl)-2-methylquinoline from 4-chloro-2-methylquinoline (1, 1 equivalent) and N-methylpiperazin (2a, 2 equivalent) in DMF at 150 C for 15 h catalyzed by different amounts of TsOH were investigated, and the results were shown in Table 1. The reaction of compound 1 and 2a without TsOH resulted in only low yield (about 6%, Table 1, entry 1). However, the use of TsOH as a catalyst led to higher yields (Table 1, entries 2-10), and the yield was gradually impoved with the increase of TsOH from 0.1 equivalent to 1.7 equivalent, but a further increase the amount of TsOH failed to improve the yield. These experimental results showed that the optimal amount of TsOH is 1.7 equivalent of compound 1.
Considering that MAOS has advantages over traditional heating methods in some cases, we further explored the reaction conditions of 4-chloro-2-methylquinoline with different primary and secondary amine using TsOH by MAOS. In order to optimize the reaction conditions, the reaction of 4-chloro-2-methylquinoline (1, 2 mmol) and N-methylpiperazine (2a, 4.4 mmol) using TsOH (3.4 mmol) by MAOS was acted as the model reaction. Different temperatures (110 C, 120 C, 130 C, 140 C, 150 C), durations (0.5 h, 1 h, 1.5 h, 2 h), and solvents (ethanol, DMF, excessive amine) were studied, and the results were summarized in Table 2.
According to our previous experiment, the reaction of compound 1 and 2a catalyzed by TsOH was firstly performed in DMF at 150 C for 1.5 h under MAOS conditions. The yield of product 3a was found to increase from 52% to 55% compared to our previous method (Table 2, entry 1 vs 2). Although the increased yield was little, the time was greatly shortened. This exciting result encouraged us to search for the optimization of the reaction conditions. Firstly, the reaction temperature was optimized (Table 2, entries 2-6). The yield was gradually improved with the decrease of temperature from 150 C to 120 C (Table 2, entries 2-5). However, a slight decrease of the yield (63%) was observed when the reaction was carried out at 110 C (Table 2, entry 6). Next, the reaction time was explored. The yield of product 3a was found to increase from 60% to 73% when the reaction time was shortened from 2 h to 1 h (Table 2, entries 5, 7, 8), but the yield was decreased when the reaction time was further shortened to 0.5 h (Table 2,
entry 8 vs 9). Fortunately, the product 3a was obtained in higher yield (81%) when the solvent was excessive amine than DMF or EtOH (Table 2, entry 11 vs 8, 10), which avoided the post-processing of DMF, decreased environmental pollution and lowered the costs. Through these experiments, a temperature at 120 C, a time of 1 h, and a solvent of excessive amine were the optimal conditions of synthesis of 4-(N-methylpiperazin-1-yl)-2-methylquinoline (Table 2, entry 11). Under these conditions, compound 3a was obtained in 81% yield.
Herein, the preparation of compound 3a by MAOS was also compared to the traditional heating method. It was found that the yield of compound 3a by MAOS were higher than the latter (Table 2, entries 2, 8, 9 vs 1, 12, 13), and the reaction time by MAOS was largely shortened. Our results showed that MAOS has evident advantages over traditional methods.
After optimizing the conditions, the substrate scope of amine was investigated using 4-chloro-2-methylquinoline and various types of amine in the presence of TsOH at 120 C under microwave-irradiation. As shown in Table 3, our proposed method could be adapted to the synthesis of 4-amino-2-methylquinoline with the following types of substituent amino: alicyclic amino (compounds 3a-3b), aromatic amino (compounds 3c-3e), and flexible aliphatic amino (compounds 3f-3o). Because the states of p-methoxyaniline and 4-(dimethylamino)pyridine are the solids, the reactions of entries 3 and 5 were processed in ethanol. It was found that these amines exhibited different reactivity under identical conditions. Amines comply with the reactivity order of aromatic amine > alicyclic amine > flexible aliphatic amine, and 4-methoxyaniline showed the best reactivity with a yield of 89% among the various types of amine (Table 3, entry 3). Furthermore, the scope of flexible aliphatic amine in this reaction was further broadened (Table 3, entries 6-15). It was found that the yields of monoamines are higher than diamines (Table 3, entries 10-15 vs 6-9) except entry 10 (55%), but different diamine showed little impact on the yield. All these results exhibited the proposed method is extendable to a wide variety of amines. The structures of the products 3a-3o were validated by their 1H NMR, 13C NMR, and HRMS spectra.
Finally, it’s important to note that all of synthesized compounds were designed specifically for the further synthesis of 4-substituted amino-2-arylethenylquinoline derivatives with potent activity against AD. In short, the method developed in our lab is to be a good alternative option for the preparation of 4- substituted amino-2-methylquinoline derivatives.
In summary, a series of 4-substituted amino-2-methylquinoline derivatives 3a-3o were successfully synthesized using TsOH at 120 C for 1 h under MAOS conditions with high yield (55%-89%). This method offers several advantages such as higher yield, shorter reaction time, lower costs, less pollution, more convenience, and higher efficiency compared to the conventional method.
EXPERIMENTAL
All reagents and solvents were purchased from commercial suppliers without further purification. All reactions were carried out using Biotage Microwave Synthesis System (Initiator); 1H and 13C NMR spectra were recorded using TMS as the internal standard in CDCl3 or DMSO-d6 with a Bruker BioSpin GmbH spectrometer at 400 MHz and 100 MHz, respectively; High resolution mass spectra (HRMS) were recorded on Shimadzu LCMS-IT-TOF spectrometer; Flash column chromatography was performed with silica gel (200-300 mesh) purchased from Qingdao Haiyang Chemical Co.Ltd.; Melting points (mp) were determined using an SRS-OptiMelt automated melting point instrument without correction.
Typical procedure for the synthesis of 3a-3o: A mixture of 4-chloro-2-methylquinoline (1.77 g, 10 mmol), various amine (50 mmol), and p-toluenesulfonic acid (17 mmol) was placed in a 5-mL pressurized microwave vial with snap on cap. The reaction suspension was subjected to microwave synthesis system to be stirred for 1 h at 120 C. After completion of the reaction, the reaction mixture was cooled to room temperature, and poured into ice water (50 mL), then aqueous NaOH was added to make the solution basic. The mixture was extracted with three 50 mL portions of CH2Cl2. The combine organic phase was washed with 50 mL water, dried over anhydrous sodium sulfate, and concentrated under reduced pressure. The crude product was purified by using flash column chromatography with CH2Cl2/MeOH (50 : 1) or CH2Cl2/petroleum ether (2 : 1) to afford compound 3a-3o.
4-(N-Methylpiperazin-1-yl)-2-methylquinoline (3a): pale yellow liquid. 1H NMR (400 MHz, CDCl3): δ 7.98 (t, J = 8.0 Hz, 2H), 7.62 (t, J = 8.0 Hz, 1H), 7.42 (t, J = 8.0 Hz, 1H), 6.74 (s, 1H), 3.27 (s, 4H), 2.73 (s, 4H), 2.69 (s, 3H), 2.43 (s, 3H). 13C NMR (101 MHz, CDCl3): δ 159.18, 156.70, 148.93, 128.89 (2C), 124.36, 123.33, 121.61, 109.25, 54.85 (2C), 51.78 (2C), 45.93, 25.40. HRMS (ESI): Cacld for [M+H]+ (C15H20N3) requires m/z 242.1652, found 242.1642.
4-(Piperidin-1-yl)-2-methylquinoline (3b): white solid, mp 41.2-42.6 C. 1H NMR (400 MHz, CDCl3): δ 7.95 (d, J = 8.0 Hz, 2H), 7.59 (t, J = 8.0 Hz, 1H), 7.40 (t, J = 8.0 Hz, 1H), 6.71 (s, 1H), 3.15 (s, 4H), 2.66 (s, 3H), 1.84 (s, 4H), 1.68 (s, 2H). 13C NMR (101 MHz, CDCl3): δ 159.32, 158.07, 149.15, 128.93, 128.89, 124.26, 123.70, 122.09, 109.24, 53.58 (2C), 26.11 (2C), 25.59, 24.48. HRMS (ESI): Cacld for [M+H]+ (C15H19N2) requires m/z 227.1543, found 227.1540.
4-(4-Methoxyanilino)-2-methylquinoline (3c): white solid, mp 135.6-136.8 C. 1H NMR (400 MHz, CDCl3): δ 7.97 (d, J = 8.3 Hz, 1H), 7.89 (d, J = 8.3 Hz, 1H), 7.65 (t, J = 7.6 Hz, 1H), 7.43 (t, J = 7.6 Hz, 1H), 7.31-7.20 (m, 2H), 6.99 (d, J = 8.8 Hz, 2H), 6.69 (s, 1H), 6.61 (s, 1H), 3.87 (s, 3H), 2.55 (s, 3H). 13C NMR (101 MHz, CDCl3): δ 159.58, 157.31, 148.91, 148.66, 132.37, 129.38, 129.18, 126.12 (2C), 124.36, 119.35, 117.71, 114.94 (2C), 101.32, 55.57, 25.64. HRMS (ESI): Cacld for [M+H]+ (C17H17N2O) requires m/z 265.1335, found 265.1324.
4-Anilino-2-methylquinoline (3d): white solid, mp 127.9-129.1 C. 1H NMR (400 MHz, CDCl3): δ 7.97 (d, J = 8.4 Hz, 1H), 7.89 (d, J = 8.2 Hz, 1H), 7.65 (t, J = 7.6 Hz, 1H), 7.46-7.40 (m, 3H), 7.28 (t, J = 7.6 Hz, 2H), 7.19 (t, J = 7.3 Hz, 1H), 6.90 (s, 1H), 6.72 (s, 1H), 2.57 (s, 3H). 13C NMR (101 MHz, CDCl3): δ 159.60, 148.87, 147.50, 140.08, 129.70 (2C), 129.46, 129.26, 124.57, 124.44, 122.64 (2C), 119.57, 118.34, 102.48, 25.67. HRMS (ESI): Cacld for [M+H]+ (C16H15N2) requires m/z 235.1230, found 235.1217.
4-(Dimethylamino)-1-(2-methylquinolin-4-yl)pyridin-1-ium (3e): white solid, mp 195.3-196.7 C. 1H NMR (400 MHz, CDCl3): δ 8.36 (d, J = 6.6 Hz, 2H), 8.19 (d, J = 8.5 Hz, 1H), 7.83 (t, J = 7.7 Hz, 1H), 7.64-7.57 (m, 2H), 7.55 (d, J = 6.4 Hz, 2H), 7.45 (d, J = 8.2 Hz, 1H), 3.47 (s, 6H), 2.86 (s, 3H). 13C NMR (101 MHz, DMSO-d6): δ 160.16, 156.93, 148.95, 145.94, 142.87 (2C), 131.38, 129.46, 128.32, 121.88, 121.60, 119.98, 108.30 (2C), 42.67 (2C), 25.37. HRMS (ESI): Cacld for [M+H]+ (C17H19N3) requires m/z 265.1573, found 265.1515.
N1,N1-Diethyl-N3-(2-methylquinolin-4-yl)propane-1,3-diamine (3f): pale yellow liquid. 1H NMR (400 MHz, CDCl3): δ 7.90 (d, J = 8.4 Hz, 1H), 7.78 (s, 1H), 7.71 (d, J = 8.4 Hz, 1H), 7.57 (t, J = 8.3 Hz, 1H), 7.32 (t, J = 8.3 Hz, 1H), 6.23 (s, 1H), 3.39 (q, J = 4.1 Hz, 2H), 2.68 (t, J = 8.3 Hz, 2H), 2.64 (q, J =8.0 Hz, 4H), 2.61 (s, 3H), 1.95-1.88 (m, 2H), 1.10 (t, J = 7.1 Hz, 6H). 13C NMR (101 MHz, CDCl3): δ 159.52, 150.72, 148.13, 128.85, 128.66, 123.35, 120.36, 117.80, 98.15, 53.46, 47.02 (2C), 44.55, 25.67, 24.47, 11.57 (2C). HRMS (ESI): Cacld for [M+H]+ (C17H26N3) requires m/z 272.2121, found 272.2127.
N1,N1-Dimethyl-N3-(2-methylquinolin-4-yl)propane-1,3-diamine (3g): pale yellow liquid. 1H NMR (400 MHz, CDCl3): δ 7.89 (d, J = 7.8 Hz, 1H), 7.64 (d, J = 7.7 Hz, 1H), 7.53 (s, 1H), 7.43 (s, 1H), 7.30 (t, J = 4.2 Hz, 1H), 6.20 (s, 1H), 3.31 (s, 2H), 2.59 (s, 3H), 2.47 (s, 2H), 2.30 (s, 6H), 1.84 (s, 2H). 13C NMR (101 MHz, CDCl3): δ 159.43, 150.64, 148.13, 128.79, 128.60, 123.59, 119.94, 117.81, 98.25, 59.06, 45.42 (2C), 43.58, 25.56, 24.81. HRMS (ESI): Cacld for [M+H]+ (C15H22N3) requires m/z 244.1808, found 244.1799.
N1,N1-Dimethyl-N2-(2-methylquinolin-4-yl)ethane-1,2-diamine (3h): pale yellow liquid. 1H NMR (400 MHz, CDCl3): δ 7.90 (d, J = 8.3 Hz, 1H), 7.76 (d, J = 8.3 Hz, 1H), 7.58 (t, J = 8.3 Hz, 1H), 7.35 (t, J = 8.3 Hz, 1H), 6.28 (s, 1H), 5.84 (s, 1H), 3.28 (dd, J = 11.3, 4.9 Hz, 2H), 2.66 (t, J = 6.9 Hz, 2H), 2.61 (s, 3H), 2.29 (s, 6H). 13C NMR (101 MHz, CDCl3): δ 159.52, 149.93, 148.14, 129.02, 128.83, 123.78, 119.67, 117.56, 99.10, 57.16, 45.06 (2C), 39.95, 25.64. HRMS (ESI): Cacld for [M+H]+ (C14H20N3) requires m/z 230.1652, found 230.1641.
N1-(2-Methylquinolin-4-yl)propane-1,3-diamine (3i): white solid, mp 98.7-99.2 C. 1H NMR (400 MHz, CDCl3): δ 7.89 (d, J = 8.4 Hz, 1H), 7.73 (d, J = 8.3 Hz, 1H), 7.57 (t, J = 7.6 Hz, 1H), 7.33 (t, J = 7.5 Hz, 1H), 6.78 (s, 1H), 6.28 (s, 1H), 3.44-3.38 (m, 2H), 3.02 (t, J = 5.8 Hz, 2H), 2.60 (s, 3H), 1.94-1.86 (m, 3H). 13C NMR (101 MHz, DMSO-d6): δ 159.15, 150.48, 148.51, 129.05, 128.76, 123.40, 121.87, 117.97, 98.38, 41.06, 39.93, 31.42, 25.74. HRMS (ESI): Cacld for [M+H]+ (C13H18N3) requires m/z 216.1495, found 216.1485.
N,N-Diethyl-2-methylquinolin-4-amine (3j): pale yellow liquid. 1H NMR (400 MHz, CDCl3): δ 8.04 (d, J = 8.4 Hz, 1H), 7.99 (d, J = 8.3 Hz, 1H), 7.62 (t, J = 7.2 Hz, 1H), 7.41 (t, J = 7.6 Hz, 1H), 6.70 (s, 1H), 3.40 (q, J = 7.1 Hz, 4H), 2.70 (s, 3H), 1.20 (t, J = 7.1 Hz, 6H). 13C NMR (101 MHz, CDCl3): δ 158.04, 156.33, 148.27, 129.41, 127.96, 124.26, 124.11, 122.40, 110.04, 46.40 (2C), 24.86, 12.21 (2C). HRMS (ESI): Cacld for [M+H]+ (C14H19N2) requires m/z 215.1543, found 215.1533.
2-Methyl-N-propylquinolin-4-amine (3k): white solid, mp 102.7-103.9 C. 1H NMR (400 MHz, CDCl3): δ 7.90 (d, J = 8.4 Hz, 1H), 7.69 (d, J = 8.2 Hz, 1H), 7.56 (t, J = 7.6 Hz, 1H), 7.31 (t, J = 7.5 Hz, 1H), 6.30 (s, 1H), 5.12 (s, 1H), 3.22 (q, J = 6.3 Hz, 2H), 2.61 (s, 3H), 1.79-1.70 (m, 2H), 1.03 (t, J = 7.3 Hz, 3H). 13C NMR (101 MHz, CDCl3): δ 159.50, 149.79, 148.24, 129.01, 128.95, 123.75, 119.23, 117.36, 98.94, 44.93, 25.74, 22.16, 11.64. HRMS (ESI): Cacld for [M+H]+ (C13H17N2) requires m/z 201.1386, found 201.1377.
3-((2-Methylquinolin-4-yl)amino)propan-1-ol (3l): white solid, mp 121.1-122.4 C. 1H NMR (400 MHz, CDCl3): δ 7.90 (d, J = 8.4 Hz, 1H), 7.66 (d, J = 8.3 Hz, 1H), 7.55 (t, J = 8.3 Hz, 1H), 7.31 (t, J = 8.3 Hz, 1H), 6.24 (s, 1H), 5.91 (s, 1H), 3.96 (t, J = 8.0 Hz, 2H), 3.48-3.42 (m, 2H), 2.72 (s, 1H), 2.59 (s, 3H), 2.07-2.01 (m, 2H). 13C NMR (101 MHz, CDCl3): δ 159.57, 150.13, 147.84, 129.07, 128.70, 123.85, 119.41, 117.28, 98.66, 61.82, 41.91, 30.71, 25.61. HRMS (ESI): Cacld for [M+H]+ (C13H17N2O) requires m/z 217.1335, found 217.1325.
N-Butyl-2-methylquinolin-4-amine (3m): pale yellow liquid. 1H NMR (400 MHz, CDCl3): δ 7.84 (d, J = 8.4 Hz, 1H), 7.68 (d, J = 8.3 Hz, 1H), 7.49 (t, J = 7.5 Hz, 1H), 7.26 (t, J = 7.5 Hz, 1H), 6.21 (s, 1H), 5.27 (s, 1H), 3.22 (q, J = 6.7 Hz, 2H), 2.54 (s, 3H), 1.70-1.61 (m, 2H), 1.47-1.35 (m, 2H), 0.91 (t, J = 7.3 Hz, 3H). 13C NMR (101 MHz, CDCl3): δ 159.02, 150.25, 147.26, 129.35, 128.15, 124.01, 119.48, 117.20, 98.83, 42.95, 30.95, 25.19, 20.36, 13.90. HRMS (ESI): Cacld for [M+H]+ (C14H19N2) requires m/z 215.1543, found 215.1543.
N-Isobutylquinolin-4-amine (3n): yellow liquid. 1H NMR (400 MHz, CDCl3): δ 7.90-7.83 (m, 2H), 7.53 (t, J = 8.2 Hz, 1H), 7.28 (t, J = 8.2 Hz, 1H), 6.28 (s, 1H), 5.74 (t, J = 5.3 Hz, 1H), 3.05 (t, J = 6.9 Hz, 2H), 2.59 (s, 3H), 2.04-1.96 (m, 1H), 0.98 (d, J = 6.7 Hz, 6H). 13C NMR (101 MHz, CDCl3): δ 159.27, 150.19, 148.11, 128.90, 128.39, 123.60, 119.84, 117.49, 98.71, 45.40, 27.42, 25.47, 20.42 (2C). HRMS (ESI): Cacld for [M+H]+ (C14H19N2) requires m/z 215.1543, found 215.1544.
N-(Prop-2-yn-1-yl)quinolin-4-amine (3o): white solid, mp 131.7-132.5 C. 1H NMR (400 MHz, CDCl3): δ 7.96 (d, J = 8.4 Hz, 1H), 7.74 (d, J = 8.3 Hz, 1H), 7.64 (t, J = 7.5 Hz, 1H), 7.41 (t, J = 7.5 Hz, 1H), 6.45 (s, 1H), 5.35 (s, 1H), 4.16 (s, 2H), 2.68 (s, 3H), 2.35 (s, 1H). 13C NMR (101 MHz, CDCl3): δ 159.44, 148.74, 147.92, 129.36, 128.96, 124.36, 119.26, 117.43, 99.98, 79.26, 72.43, 32.96, 25.72. HRMS (ESI): Cacld for [M+H]+ (C13H13N2) requires m/z 197.1073, found 197.1077.
ACKNOWLEDGEMENTS
We are grateful for financial support from the Natural Science Foundation of China (21502025), the Science and Technology Program of Dongguan (2012108102045), the Science and Technology Development Program of Dongguan (2013108101059), and the Program of Guangdong Medical University (M2013017), and the Students' Innovative Program of Guangdong Medical University (XJ105711501, 2015ZYDM017) for financial support of this research.
References
01. S. A. Lawrence, 'Amines: Synthesis, Properties, and Application', Cambridge University Press, Cambridge, 2004.
02. S. A. Lawrence, 'Amines: Synthesis, Properties, and Application', Cambridge University Press, Cambridge, 2004; (b) M. C. Lombard, D. D. N’Da, J. C. Breytenbach, N. I. Kolesnikova, C. Tran Van Ba, S. Wein, J. Norman, P. Denti, H. Vial, and L. Wiesner, Eur. J. Pharm. Sci., 2012, 47, 834;; CrossRef (c) Y. Aubin, C. Fischmeister, C. M. Thomas, and J. L. Renaud, Chem. Soc. Rev., 2010, 39, 4130. CrossRef
03. M. Kosugi, M. Kameyama, and T. Migita, Chem. Lett., 1983, 12, 927. CrossRef
04. A. S. Guram, R. A. Rennels, and S. L. Buchwald, Angew. Chem., Int. Ed. Engl., 1995, 34, 1348. CrossRef
05. (a) F. Paul, J. Patt, and J. F. Hartwig, J. Am. Chem. Soc., 1994, 116, 5969;; CrossRef (b) J. Louie and J. F. Hartwig, Tetrahedron Lett., 1995, 36, 3609. CrossRef
06. (a) J. F. Hartwig, Angew. Chem. Int. Ed., 1998, 37, 2046;; CrossRef (b) C. C. Johansson Seechurn, M. O. Kitching, T. J. Colacot, and V. Snieckus, Angew. Chem. Int. Ed., 2012, 51, 5062;; CrossRef (c) P. Appukkuttan and E. V. Eycken, Eur. J. Org. Chem., 2008, 7, 1133;; CrossRef (d) C. Valente, S. Calimsiz, K. H. Hoi, D. Mallik, M. Sayah, and M. G. Organ, Angew. Chem. Int. Ed., 2012, 51, 3314. CrossRef
07. (a) M. Huang, L. L. Wang, X. H. Zhu, Z. X. Mao, D. Z. Kuang, and Y. Q. Wan, Eur. J. Org. Chem., 2012, 26, 4897;; CrossRef (b) D. Hollmann, S. Bahn, A. Tillack, and M. Beller, Chem. Commun., 2008, 27, 3199;; CrossRef (c) M. Al-Amin, T. Honma, N. Hoshiya, S. Shuto, and M. Arisawa, Adv. Synth. Catal., 2012, 354, 1061;; CrossRef (d) S. Imm, S. Bhn, L. Neubert, H. Neumann, and M. Beller, Angew. Chem., 2010, 122, 8303;; CrossRef (e) D. S. Yadav, B. S. Yadav, and V. K. Rai, Synthesis, 2006, 1868. CrossRef
08. (a) A. Dumrath, X. F. Wu, H. Neumann, A. Spannenberg, R. Jackstell, and M. Beller, Angew. Chem. Int. Ed., 2010, 49, 8988;; CrossRef (b) A. Borzenko, N. L. Rotta-Loria, P. M. MacQueen, C. M. Lavoie, R. McDonald, and M. Stradiotto, Angew. Chem. Int. Ed., 2015, 54, 1;; CrossRef (c) T. Schulz, C. Torborg, S. Enthaler, B. Schaffner, A. Dumrath, A. Spannenberg, H. Neumann, A. Boner, and M. Beller, Chem. Eur. J., 2013, 19, 16760;; CrossRef (d) A. K. Verma, R. R. Jha, R. Chaudhary, R. K. Tiwari, and A. K. Danodia, Adv. Synth. Catal., 2013, 355, 421;; (e) Y. Tan and J. F. Hartwig, J. Am. Chem. Soc., 2010, 132, 3676. CrossRef
09. A. Castello, J. Cervello, J. Marquet, M. Moreno-Manas, and X. Sirera, Tetrahedron, 1986, 42, 4073. CrossRef
10. Y. Sawada, H. Kayakiri, Y. Abe, T. Mizutani, N. Inamura, M. Asano, I. Aramori, C. Hatori, T. Oku, and H. Tanaka, J. Med. Chem., 2004, 47, 2667. CrossRef
11. J. Y. Tsai, C. S. Chang, Y. F. Huang, H. S. Chen, S. K. Lin, F. F. Wong, L. J. Huang, and S. C. Kuo, Tetrahedron, 2008, 64, 11751. CrossRef
12. A. Dumrath, C. Lubbe, H. Neumann, R. Jackstell, and M. Beller, Chem. Eur. J., 2011, 17, 9599. CrossRef
13. (a) S. W. Wu, J. L. Liu, and F. Liu, Org. Lett., 2016, 18, 1;; CrossRef (b) V. Polshettiwar and R. S. Varma, 'Aqueous Microwave Assisted Chemistry Synthesis and Catalysis', Royal Society of Chemistry, Cambridge, 2010;; CrossRef (c) A. Mishra and R. Dubey, Green Chem. Environ. Remed., 2012, 13, 379;; CrossRef (d) G. Y. Yang, J. T. Yang, C. X. Wang, S. F. Fan, P. H. Xie, and C. L. Xu, Heterocycles, 2013, 87, 1327;; CrossRef (e) A. Rohadi, S. A. Hasbullah, A. M. Lazim, and R. Nordin, Heterocycles, 2014, 89, 1017. CrossRef
14. X. Q. Wang, C. L. Xia, S. B. Chen, J. H. Tan, T. M. Ou, S. L. Huang, D. Li, L. Q. Gu, and Z. S. Huang, Eur. J. Med. Chem., 2015, 89, 349 CrossRef