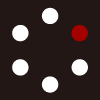
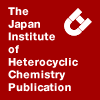
HETEROCYCLES
An International Journal for Reviews and Communications in Heterocyclic ChemistryWeb Edition ISSN: 1881-0942
Published online by The Japan Institute of Heterocyclic Chemistry
e-Journal
Full Text HTML
Received, 11th May, 2016, Accepted, 6th June, 2016, Published online, 9th June, 2016.
DOI: 10.3987/COM-16-13499
■ A Convenient Synthetic Route for Substituted 1-(Aryldiazenyl)Imidazo[1,5-a]Pyridine
Mustafa M. El-Abadelah,* Hamdallah A. Hodali, Monther S. Zreid, Firas F. Awwadi, Mohammed M. Abadleh, and Wolfgang Voelter
Department of Chemistry, Faculty of Science, The University of Jordan, Amman 11942, Jordan
Abstract
In the presence of 2,3-dichloro-5,6-dicyano-1,4-benzoquinone (DDQ), model 2-(N'-aryl)picolinohydrazonamides 7, 8 (derived from interaction of the respective ethyl glycinate and (2-pyridyl)methylamine with the particular hydrazonoyl chloride) underwent didehydrogenation with concomitant heterocyclization to deliver the corresponding 1-(aryldiazenyl)imidazo- [1,5-a]pyridines 9, 10. Structures of the latter bicyclic systems are deduced from spectral data and confirmed by X-ray diffraction analysis of the Pd-complex 11, derived from 9.Nitrogen-bridgehead-fused heterocycles encompassing an imidazole ring are common structural components in several pharmacologically important molecules.1, 2 Recently, increasing attention has been paid to members of this family that contain imidazo[1,5-a]pyridine skeleton.2 Interestingly, this bicyclic heterocycle is found in the antibiotic cribrostatin-6 (Figure 1) isolated from the blue marine sponge Cribrochalina sp.3 Most of the reported routes toward synthesis of imidazo[1,5-a]pyridines utilize suitably 2-substituted pyridines and constructing the imidazole ring thereupon. α-(2-Pyridyl)alkylamines have become widely applicable synthons, whereby functionalization of the NH2 group to anchor various electrophilic reagents is followed by oxidative heterocyclization of the derivatized components such as amides,4 thioamides5 and imines.6 Other approaches include the use of 2-cyanopyridines,7 methyl picolinate,8 2,2'-pyridyls,9 dipyridyl ketones,10 imidazoles,11 1,1-dibromoalkenes,12 and benzotriazole mediated methodology.13
Imidazo[1,5-a]pyridines find potential applications in key areas such as organic light-emitting diodes (OLEDs)14 and organic thin-layer field effect transistors (FETs).15 These heterocycles also display a broad spectrum of biological activities including cardiotonic,16 antibacterial17 and antitumoral18 agents, inhibitors of thromboxane synthetase,19 MEK kinase,20 aromatase21 and HIV-protease.22
In addition, several cationic azo dyestuffs of the general formulas 1-3 (Figure 2) were described and patented23-25 for use in coloration of synthetic polymers (in the form of ribbons, tapes, fibers, films, threads, and textile materials). These azo dyes were prepared by coupling of various arenediazonium salts with the appropriate imidazo[1,5-a]pyridines and subsequent alkylation of the N(2)-position; the coupling reaction occurs preferentially at C-3 position24,25 and at C-1 of 3-substituted systems.23 1-(Aryldiazinyl)imidazo[1,5-a]pyridines, such as 4 (precursors of 3 / Figure 2), are also regarded as N,N-bidentate ligands capable of chelating various metal ions, and the resultant complexes might possess a range of magnetic, photophysical and electrochemical properties. The development of new synthetic methodologies for these and related N,N-bidentate ligands is of paramount importance in chemistry.
Herein we wish to report on a new convenient route towards the synthesis of model 1-(aryldiazinyl)imidazo[1,5-a]pyridines (9,10), skipping the penultimate diazo-coupling step as illustrated in Schemes 1-4, together with a representative Pd-complex 11 derived from 9 (Scheme 5).
Chemistry
In this study the N'-(4-nitrophenyl)-2-pyridinecarbohydrazonoyl chloride precursor 623 is prepared by direct chlorination of the respective 2-{[2-(4-nitrophenyl)hydrazono]methyl}pyridine 5 using N-chlorosuccinimide (NCS) (Scheme 1). The latter hydrazone is accessible via condensation of 2-pyridinecarboxaldehyde with 4-nitrophenylhydrazine.26
The reaction of α-amino esters with nitrile imine 1,3-dipolar species is a well-known route towards the synthesis of various 4,5-dihydro-1,2,4-triazin-6-ones.27 Ethyl glycinate, acting as nitrogen nucleophile, adds readily onto N-(4-nitrophenyl)nitrile imine 6A (the respective 1,3-dipole is generated in situ from its hydrazonoyl chloride precursor 6 in presence of triethylamine) to deliver the respective acyclic amidrazone adduct 7 as the lonely isolable product (Scheme 2). However, under the prevailing reaction conditions further intramolecular cyclization of 7 (an allowed 6-exo-trig process involving the amidrazone NH and the ester carbonyl group28) to form the respective dihydrotriazinone did not take place (Scheme 2). This is probably due to the decreased nucleophilicity of the amidrazone NH residue caused by the strong conjugative electron-withdrawing effect exerted by the p-nitro group. The amidrazone adduct 8 is similarly prepared by reacting α-(2-pyridyl)methylamine with the hydrazonoyl chloride (6) in the presence of triethylamine (Scheme 2).
The new compounds 7, 8 were characterized by elemental analysis (Table 1), MS and NMR spectral data. These data, given in the experimental section, are in conformity with the assigned structures. Thus, the mass spectra display the correct molecular ion peaks for which the measured high resolution (HRMS-ESI) m/z data are in good agreement with those calculated from the respective molecular formulas. The 1H NMR spectrum of 7 displays two sets of signals for each type of protons (with relative integration peak areas 3 : 2 ) indicative for the presence of E / Z diastereomers (with a ratio 3 : 2) originating from the restricted rotation around the amidrazone C=N bond (Figure 3). The existence of both diastereomers is also stressed by the presence of signal doubling for each of the different carbons in the 13C NMR spectrum of 7. This phenomenon of Z- and E-isomerism has recently been revealed by spectroscopic NMR and X-ray studies on the structures of closely related N'-aryl and N'-acyl amidrazones.29 Likewise, the NMR spectra of 8 show signal doubling arising from E / Z isomerism with a ratio 3 : 1 for the various protons and carbons. DEPT and 2D (COSY, HMQC, HMBC) experiments showed correlations that helped in the 1H and 13C signal assignments to the different carbons and their attached and / or neighboring hydrogen atoms.
The amidrazone adducts (7 / 8) were subjected to oxidation using 2,3-dichloro-5,6-dicyano- 1,4-benzoquinone (DDQ) in ethyl acetate at reflux. Work-up of the reaction mixture gave, to our delight, good yields of the respective 1-(aryldiazenyl)imidazo[1,5-a]pyridines (9 and 10, Scheme 3), the structures of which were evidenced from analytical and spectral data (given in the experimental part) and unambiguously confirmed by single-crystal X-ray crystallography of the representative Pd-complex 11 derived from 9 (Scheme 5 and Figure 4 / vide infra). The formation of the nitrogen donor ligands 9 / 10 is suggestive of two consecutive dehydrogenation processes leading to the formation of the intermediates 7A/ 8A that transform to 7B / 8B with concomitant heterocyclization as depicted in the annexed mechanism (Scheme 4). Each dehydrogenation process by DDQ is essentially bimolecular proceeding via a two-step heterolytic pathway that includes the transfer of hydride to the quinone oxygen followed by the transfer of a proton to the phenolate ion.
Noteworthy is that DDQ stands among the most important reagents in synthetic organic chemistry;30 it is commercially available and is frequently utilized for the dehydrogenation of hydroaromatics and also for the synthesis of unsaturated heterocycles. In this context, it is worth mentioning that diaminomaleonitrile Schiff bases underwent oxidative cyclization to the corresponding imidazoles by the action of DDQ.31 Likewise, 2-substituted benzimidazoles were prepared through one-pot reaction of o-phenylenediamine with various aldehydes in the presence of DDQ,32 while thiocarbohydrazones were readily cyclized to the corresponding 1,3,4-thiadiazoles upon treatment with DDQ.33 To the best of our knowledge, the present findings represent the first report on the successful application of DDQ ''as an oxidative agent'' in the synthesis of imidazo[1,5-a]pyridines and related nitrogen-bridgehead-fused heterocycles. Currently, we are expanding the scope of the reaction to other amidrazones and related nitrogen-bridgehead-fused heterocycles.
X-Ray Structure
An X-ray crystal structure determination was performed to confirm the structure of 11 (Scheme 5), a Pd-complex of 9 as a representative example of the new synthetic 1-(aryldiazenyl)imidazo[1,5-a]pyridines. A summary of data collection and refinement parameters is given in Table 1, while selected bond lengths and angles are provided in Table 2. The molecular structure of 11, based on crystallographic data, is displayed in Figure 4. The geometry around the Pd ion is distorted square planar. The average Pd-Cl bond distances is 2.272 Å, and the average Pd-N distances is 2.049 Å (Table 2); these values agree with average reported values in similar complexes.34 Due to the rigidity of the organic ligand, the N-Pd-N bite angle (78.96(10)) is more acute than that of Cl-Pd-Cl angle (88.28(3)) and N-Pd-Cl angles (97.15(7) and 95.48(7)).
The non-classical hydrogen bonding interactions (C-H…Cl and C-H…O) and chlorine…chlorine contacts connect the molecular units to form the three-dimensional structure. The C-H…Cl interactions link the molecular units to form chain structure (Figure 5). The dichloromethane molecule is bonded to the chain structure by different C-H…Cl hydrogen bonding interactions which robust the chain structure. Two crystallographically equivalent dichloromethane molecules are connected via chlorine …chlorine contacts (Figure 5). The inter-chlorine distance is 3.326 Å which is 0.174 Å less than the sum of van der Waals radii. The C-Cl…Cl angle is 151.3 which is within the preferred arrangements of chlorine…chlorine contacts.35 The Cl…Cl interactions link the chains to form ladder structures. These ladders aggregate via non-classical hydrogen bonding interactions to form the final three-dimensional structure.
EXPERIMENTAL
2-Pyridinecarboxaldehyde, 4-nitrophenylhydrazine, glycine ethyl ester hydrochloride, (2-pyridyl)methylamine, DDQ, and NCS were purchased from Acros and used as received. Melting points (uncorrected) were determined on a Stuart scientific melting point apparatus in open capillary tubes. 1H, 13C, and 2D NMR spectra were recorded on a 500 MHz spectrometer (Bruker Avance-III) with TMS as internal standard. Chemical shifts are expressed in δ units; J values for 1H-1H coupling constants are given in Hertz. High-resolution mass spectra (HRMS) were measured (in positive ion mode) using the electrospray ion trap (ESI) technique by collision-induced dissociation on a Bruker APEX-IV (7 Tesla) instrument. The samples were dissolved in chloroform and infused using a syringe pump with a flow rate of 2 µL / min. External calibration was conducted using an arginine cluster at a mass range of m/z 175-871. Microanalyses data (for C, H, N) were performed on a Euro Vector elemental analyzer, model EA 3000, and the results agreed with the calculated percentage values within experimental error (±0.4 %).
N'-(4-Nitrophenyl)-2-pyridinecarbohydrazonoyl chloride (6). This hydrazonoyl chloride is prepared by the following procedure which is essentially similar to that reported in the patent publications36 with slight modifications: To a stirred and cooled solution (-4 to 0 oC) of 2-{[2-(4-nitrophenyl)hydrazono]- methyl}pyridine (5,26 6.0 g, 2.5 mmol) in DMF (30 mL) was added dropwise a solution of NCS (3.67 g, 2.75 mmol) in DMF (25 mL) over 30 min. Thereafter, the resulting dark brown mixture was stirred at rt for 6 h, then poured onto ice-cold water (100 mL) with stirring. The resulting light brown solid product was filtered and dried in a vacuum oven at 40 oC for 5 h. Yield 5.66 g (82 %); mp 210-212 oC. 1H NMR (500 MHz, CDCl3) : δ = 7.47 (dd, J = 4.8 Hz, 7.8 Hz, 1H, H-5), 7.53 (d, J = 9.2 Hz, 2H, H-2' / H-6'), 7.90 (ddd, J = 8 Hz, 4.8 Hz, 1 Hz, 1H, H-4), 8.13 (d, J = 8 Hz, 1H, H-3), 8.17 (d, J = 9.2 Hz, 2H, H-3'/H-5'), 8.65 (dd, J = 4.8 Hz, 1 Hz, 1H, H-6), 10.88 (s, 1H, N-H, exchangeable with D2O). 13C NMR (125 MHz, CDCl3): δ = 113.4 (C-2'/C-6'), 121.4 (C-3), 124.5 (C-5), 125.6 (C-3' / C-5'), 127.8 (C-4'), 136.9 (C-4), 140.3 (Cl-C=N), 149.1 (C-6), 149.3 (C-1'), 150.2 (C-2). HRMS ((+)-ESI): m/z = 277.04835 (calcd. 277.04868 for C12H10ClN4O2, [M + H]+). Anal. Calcd for C12H9ClN4O2: C, 52.09; H, 3.28; N, 20.25. Found: C, 52.04; H, 3.50; N, 19.96.
Ethyl 2-[N'-(4-nitrophenyl)picolinohydrazonamido]acetate (7). To a stirred solution of the hydrazonoyl chloride (6, 1.0 g, 3.6 mmol) and NEt3 (1.0 g, 10 mmol) in EtOH (20 mL), cooled to 0 oC, was added glycine ethyl ester hydrochloride (0.60 g, 4 mmol). The reaction mixture was stirred for 20–30 min at 0-4 oC and then at rt for 3-4 h. Thereafter, ice-cold water (100 mL) was added to the reaction mixture and the resulting red precipitate was filtered and dried. Yield 1.0 g (83 %); mp 228-230 oC. 1H NMR (500 MHz, CDCl3): E / Z : δ = 1.37 / 1.34 (t / t, J = 7.1 Hz / 7.1 Hz, 3H, CH3CH2), 4.13 / 4.05 (d / d, J = 4.7 Hz / 6.2 Hz, 2H, N-CH2), 4.31 / 4.33 (q / q, J = 7.1 Hz / 7.1 Hz, 2H, CO2CH2Me), 4.66 / 6.47 (pseudo-t /pseudo- t, 1H, N(3'')-H, exchangeable with D2O), 7.12 / 6.99 (d / d, J = 8.7 Hz / 8.7 Hz, 2H, H-2' + H-6'), 7.45 / 7.33 (dd / dd, J = 4.7 Hz, 7.8 Hz / 4.7 Hz, 7.8 Hz, 1H, H-5), 7.74 / 8.23 (d / d, J = 8.1 Hz / 8.1 Hz, 1H, H-3), 7.94 / 7.77 (ddd /ddd, J = 8.1 Hz, 7.8 Hz, 1.6 Hz / 8.1 Hz, 7.8 Hz, 1.6 Hz, 1H, H-4), 8.19 / 8.15 (d / d, J = 8.7 Hz / 8.7 Hz, 2H, H-3' + H-5'), 8.73 / 8.55 (d / d, J = 4.7 Hz / 4.7 Hz, 1H, H-6), 12.37 / 8.77 (s / s, 1H, N(1'')-H, exchangeable with D2O). 13C NMR (125 MHz, CDCl3): E / Z: δ = 14.3 / 14.2 (CH3CH2-), 44.6 / 46.1 (-NH-CH2-), 61.3 / 62.0 (-CH2-Me), 111.9 / 110.6 (C-2' / C6'), 121.9 / 120.7 (C-3), 124.4 / 124.1 (C-5), 126.3 / 126.1 (C-3' / C-5'), 138.0 / 136.7 (C-4), 140.4 / 138.3 (C-4'), 146.6 / 146.8 (C-2), 148.3 / 148.0 (C-6), 150.8 / 150.6 (-HN-C=N-), 150.9 / 151.0 (C-1'), 171.4 / 173.0 (-CO2Et). HRMS ((+)-ESI): m/z = 344.13391 (calcd. 344.13533 for C16H18N5O4, [M + H]+), 366.11731 (calcd. 366.11728 for C16H17N5NaO4, [M + Na]+), 709.24538 (calcd. 709.24533 for C32H34N10NaO8, [2M + Na]+). Anal. Calcd for C16 H17 N5 O4: C, 55.97; H, 4.99; N, 20.40. Found: C, 56.23; H, 5.12; N, 20.37.
N'-(4-Nitrophenyl)-N-(pyridin-2-ylmethyl)picolinohydrazonamide (8). To a stirred solution of the hydrazonoyl chloride (6, 1.0 g, 3.6 mmol) and NEt3 (1.0 g, 10 mmol) in EtOH (20 mL), cooled at 0 oC, was added picoline amine (0.45 g, 4 mmol). The reaction mixture was stirred at 0-4 oC for 20–30 min, and then at rt for 3-4 h. Thereafter, ice-cold water (100 mL) was added to the reaction mixture and the resulting red precipitate was filtered and dried. Yield 0.8 g (64%); mp 173-175 oC. 1H NMR (500 MHz, CDCl3): E / Z : δ = 4.58 / 4.66 (d / d, J = 7.9 Hz / 4.3 Hz, 2H, N-CH2), 6.81 / 5.58 (t / t , J = 7.9 Hz / 4.3 Hz, 1H, -NH-CH2, exchangeable with D2O), 7.15 / 6.92 (d / d, J = 8.9 Hz / 8.9 Hz, 2H, H-2' / H-6'), 7.25 / 7.21 (pseudo-t / pseudo-t , 1H, H-5''), 7.28 (d, J = 8.1 Hz, 1H, H-3''), 7.35 / 7.41 (pseudo-t / pseudo-t , 1H, H-5), 7.73 / 7.69 (pseudo-t / pseudo-t, 1H, H-4''), 7.78 / 7.91 (pseudo-t / pseudo-t, 1H, H-4), 8.13 / 8.09 (d / d, J = 8.9 Hz / 8.9 Hz, 2H, H-3' + H-5'), 8.27 (d, J = 8.1 Hz, 1H, H-3), 8.47 / 8.56 (d / d, J = 4.5 Hz / 4.5 Hz, 1H, H-6''), 8.74 (d, J = 4.5 Hz, 1H, H-6), 11.78 / 12.12 (s / s, 1H, NH-N=C-, exchangeable with D2O). 13C NMR (125 MHz, CDCl3): E / Z: δ = 47.9 / 47.3 (-NH-CH2-), 111.1 / 110.4 (C-2' / C6'), 120.6 (C-3), 122.8 / 122.1 (C-5), 123.3 / 122.3 (C-3''), 123.8 / 124.3 (C-5''), 126.3 / 126.4 (C-3' / C-5'), 136.6 / 136.7 (C-4''), 137.8 / 138.0 (C-4), 138.6 / 138.0 (C-4'), 144.2 (-HN-C=N-), 147.6 / 148.0 (C-6''), 149.0 / 148.9 (C-6), 151.5 (C-2''), 151.7 /151.4 (C-1'), 158.7 / 157.3 (C-2). HRMS ((+)-ESI): m/z = 349.14079 (calcd. 349.14075 for C18H17N6O2, [M + H]+), 371.12266 (calcd. 371.12269 for C18H16N6NaO2, [M + Na]+), 719.25594 (calcd. 719.25617 for C36H32N12NaO4, [2M + Na]+). Anal. Calcd for C18H16N6O2: C, 62.06; H, 4.63; N, 24.12. Found: C, 61.88; H, 4.54; N, 23.96.
Ethyl 1-[(4-nitrophenyl)diazenyl]imidazo[1,5-a]pyridine-3-carboxylate (9). A solution of 7 (1.0 g, 2.9 mmol) and DDQ (1.4 g, 6.0 mmol) in EtOAc (30 mL) was refluxed for 8 h. The solvent was then evaporated under reduced pressure, the residue dissolved in CH2Cl2 (200 mL), washed with saturated aqueous sodium carbonate (2 × 120 mL) and dried over anhydrous magnesium sulfate. Evaporation of the solvent gave 0.7 g (70%) of the title product; mp 236-238 oC. 1H NMR (500 MHz, CDCl3) : δ = 1.53 (t, J = 7.1 Hz, 3H, CH3), 4.56 (q, J = 7.1 Hz, 2H, CH2-Me), 7.24 (pseudo-t, 1H, H-6), 7.56 (pseudo t, 1H, H-7), 8.12 (d, J = 8.9 Hz, 2H, H-2' / H-6'), 8.38 (d, J = 8.9 Hz, 2H, H-3' / H-5'), 8.56 (dd, J = 5.2 Hz, 1.2 Hz, 1H, H-8), 9.54 (dd, 7.2 Hz, 1.6 Hz, 1H, H-5). 13C NMR (125 MHz, CDCl3) : δ = 14.4 (CH3), 62.1 (CH2-Me), 117.8 (C-6), 120.1 (C-8), 123.0 (C-2' / C-6'), 124.7 (C-3' / C-5'), 126.5 (C-5), 127.9 (C-1'), 128.8 (C-4'), 129.1 (C-7), 143.4 (C-1), 148.0 (C-8a), 156.6 (C-3), 159.7 (CO2Et). HRMS ((+)-ESI): m/z = 362.08579 (calcd. 362.08598 for C16H13N5NaO4, [M + Na]+), 701.18277 (calcd. 701.18273 for C32H26N10NaO8, [2M + Na]+). Anal. Calcd for C16H13N5O4: C, 56.64; H, 3.86; N, 20.94. Found: C, 56.47; H, 3.81; N, 20.86.
1-[(4-Nitrophenyl)diazenyl]-3-(pyridin-2-yl)imidazo[1,5-a]pyridine (10). A solution of DDQ (0.98 g, 4.32 mmol) in EtOAc (10 mL) was slowly added to a hot solution (60 oC) of 8 (0.75 g, 2.15 mmol) in EtOAc (20 mL). The reaction mixture was maintained at 60-65 oC for 5-10 min, then allowed to cool down to room temperature. The resulting dark violet precipitate was filtered, washed with saturated aqueous sodium hydrogencarbonate (2 × 50 mL), water (2 × 20 mL) and dried. Yield 0.50 g (70%); mp 290-292 oC. 1H NMR (500 MHz, CDCl3) : δ = 7.08 (pseudo-t, 1H, H-6), 7.32 (dd, J = 7.1 Hz, 4.5 Hz, 1H, H-5''), 7.43 (dd, J = 8.7 Hz, 7.2 Hz 1H, H-7), 7.85 (ddd, J = 8.1 Hz, 7.1 Hz, 1.5 Hz, 1H, H-4''), 8.08 (d, J = 8.9 Hz, 2H, H-2' / H-6'), 8.34 (d, J = 8.9 Hz, 2H, H-3' / H-5'), 8.45 (d, J = 8.7 Hz, 1H, H-8), 8.59 (d, J = 8.1 Hz, 1H, H-3''), 8.69 (d, J = 4.5 Hz, 1H, H-6''), 10.25 (d, J = 7.2 Hz, 1H, H-5). 13C NMR (125 MHz, CDCl3) : δ = 116.4 (C-6), 119.3 (C-8), 122.7 (C-2' / C-6'), 123.3 (C-3''), 123.5 (C-5''), 124.7 (C-3' / C-5'), 128.1 (C-5), 128.2 (C-7), 131.2 (C-8a), 136.9 (C-4''), 137.4 (C-3), 143.7 (C-1), 147.4 (C-4'), 148.3 (C-6''), 149.9 (C-2''), 157.4 (C-1'). HRMS ((+)-ESI): m/z = 345.10926 (calcd. 345.10945 for C18H13N6O2, [M + H]+). Anal. Calcd for C18H12N6O2: C, 62.79; H, 3.51; N, 24.41. Found: C, 62.58; H, 3.44; N, 24.23.
Dichloro{ethyl 1-[(4-nitrophenyl)diazenyl]imidazo[1,5-a]pyridine-3-carboxylate}palladium(II) (11). A solution of 9 (0.33 g, 1 mmol) and dichlorobis(benzonitrile)palladium(II) (0.38 g, 1 mmol) in CH2Cl2 (50 mL) was refluxed for 2 h. The volume of the solvent was then reduced to the half by evaporation in vacuo. Thereafter, the red precipitate was collected under suction in a sintered glass funnel, washed with Et2O (2 × 10 mL) and dried. Yield 0.26 g (52%); mp 255-257 oC (decomp). 1H NMR (500 MHz, DMF-d7) : δ = 1.44 (t, J = 7.1 Hz, 3H, CH3CH2-), 4.53 (q, J = 7.1 Hz, 2H, CH2Me), 7.75 (pseudo-t, 1H, H-6), 8.06 (d, J = 8.8 Hz, 2H, H-2' / H-6'), 8.20 (pseudo t, 1H, H-7), 8.41 (d, J = 8.8 Hz, 2H, H-3' / H-5'), 8.62 (d, J = 8.9 Hz, 1H, H-8), 9.11 (d, 6.9 Hz, 1H, H-5). 13C NMR (125 MHz, DMF-d7) : δ = 14.6 (-CH2CH3), 61.9 (CH2Me), 119.1 (C-6), 120.1 (C-8), 123.2 (C-2' / C-6'), 125.6 (C-3' / C-5'), 127.4 (C-5), 128.6 (C-1'), 129.0 (C-4'), 131.5 (C-7), 143.3 (C-1), 148.0 (C-8a), 156.9 (C-3), 159.5 (CO2Et). Anal. Calcd for C16 H13 Cl2 N5 O4 Pd.CH2Cl2: C, 33.94; H, 2.51; N, 11.94. Found: C, 34.12; H, 2.46; N, 11.82.
X-Ray structure analysis of Pd-complex 11. Crystals were grown slowly in two weeks from a dilute solution of 11 in CH2Cl2 by vapor diffusion method using benzene. The title compound crystallized out as rhombic red crystals. A suitable crystal, with approximate dimensions of 0.3 × 0.2 × 0.15 mm, was epoxy-mounted on a glass fiber. Data were collected at room temperature (293 K) using an Oxford Xcalibur diffractometer. Data were acquired and processed to give SHELX-format-hkl files using CrysAlisPro software.37 Cell parameters were determined and refined using CrysAlisPro.37 A multiscan absorption collection was applied with maximum and minimum transmission factors of 1.00000 and 0.97677, respectively. The structure was solved by direct methods and refined by full-matrix least-squares on F2 using all unique data.38 All nonhydrogen atoms were refined anisotropically with the hydrogen atoms placed on the calculated positions using riding model.
Crystallographic data for the structural analysis of the Pd-complex 11 have been deposited with the Cambridge Crystallographic Data Center under the depository No. 1445000. Copies of information may be obtained free of charge from the Director, CCDC, 12 Union Road, Cambridge CB2 IEZ, UK (Fax: +44-1223-336033; e-mail: (deposit@ccdc.com.ac.uk or http://www.ccdc.ac.uk).
ACKNOWLEDGEMENTS
We wish to thank the Deanship of Scientific Research at The University of Jordan, Amman-Jordan, for financial support.
References
1. J. A. Montgomery and J. A. Secrist, 'In Comprehensive Heterocyclic Chemistry III', Vol. 5, ed. by A. R. Katritzky and C. W. Rees, 1984, Pergamon Press, Oxford, p. 634.
2. A. S. Howard, 'In Comprehensive Heterocyclic Chemistry', Vol. 8, ed. by A. R. Katritzky, C. W. Rees, and E. F. Scriven, Pergamon Press, Oxford, 1995, p 272.
3. G. R. Pettit, J. C. Collins, J. C. Knight, D. L. Herald, R. A. Nieman, M. D. Williams, and R. K. Pettit, J. Nat. Prod., 2003, 66, 544; CrossRef D. Knueppel and S. F. Martin, Angew. Chem. Int. Ed., 2009, 48, 2569. CrossRef
4. J. D. Bower and G. R. Ramage, J. Chem. Soc., 1955, 2834; CrossRef K. Winterfeld and H. Franzke, Angew. Chem. Int. Ed., 1963, 2, 736; CrossRef J. J. Li, J. J. Li, J. Li, A. K. Trehan, H. S. Wong, S. Krishnananthan, L. J. Kennedy, Q. Gao, A. Ng, J. A. Robl, B. Balasubramanian, and B.-C. Chen, Org. Lett., 2008, 10, 2897; CrossRef J. M. Crawforth and M. Paoletti, Tetrahedron Lett., 2009, 50, 4916. CrossRef
5. F. Shibahara, A. Kitagawa, E. Yamaguchi, and T. Murai, Org. Lett., 2006, 8, 5621; CrossRef H. S. El-Khadem, J. Kawai, and D. L. Swartz, Heterocycles, 1989, 28, 239; CrossRef J. Bourdais and A.-M. M. E. Omar, J. Heterocycl. Chem., 1980, 17, 555; CrossRef A. Moulin, S. Garcia, J. Martinez, and J.-A. Fehrentz, Synthesis, 2007, 2667. CrossRef
6. A. P. Krapcho and J. R. Powell, Tetrahedron Lett., 1986, 27, 3713; CrossRef M. E. Bluhm, M. Ciesielski, H. Görls, and M. Döring, Angew. Chem. Int. Ed., 2002, 41, 2962; CrossRef F. Shibahara, R. Sugiura, E. Yamaguchi, A. Kitagawa, and T. Murai, J. Org. Chem., 2009, 74, 3566. CrossRef
7. F. Palacios, C. Alonso, and G. Rubiales, Tetrahedron, 1995, 51, 3683. CrossRef
8. V. S. Arvapalli, G. Chen, S. Kosarev, M. E. Tan, D. Xie, and L. Yet, Tetrahedron Lett., 2010, 51, 284. CrossRef
9. J. Wang, R. Mason, D. VanDerveer, K. Feng, and X. R. Bu, J. Org. Chem., 2003, 68, 5415. CrossRef
10. J. Wang, L. Dyers, Jr., R. Mason, Jr., P. Amoyaw, and X. R. Bu, J. Org. Chem., 2005, 70, 2353; CrossRef S. A. Siddiqui, T. M. Potewar, R. J. Lahoti, and K. V. Srinivasan, Synthesis, 2006, 2849. CrossRef
11. D. D. Davey, J. Org. Chem., 1987, 52, 1863. CrossRef
12. A. Zhang, X. Zheng, J. Fan, and W. Shen, Tetrahedron Lett., 2010, 51, 828. CrossRef
13. A. R. Katritzky and G. Qiu, J. Org. Chem., 2001, 66, 2862. CrossRef
14. M. Nakatsuka and T. Shimamura, Jpn. Kokai Tokkyo Koho, 2001, JP 2001035664 (Chem. Abstr., 2001, 134, 170632); T. Tominaga, T. Kohama, and A. Takano, Jpn. Kokai Tokkyo Koho, 2001, JP 2001006877 (Chem. Abstr., 2001, 134, 93136); D. Kitasawa, T. Tominaga, and A. Takano, Jpn. Kokai Tokkyo Koho, 2001, JP 2001057292 (Chem. Abstr., 2001, 134, 200276).
15. H. Nakamura and H. Yamamoto, PCT Int. Appl., 2005, WO 2005043630 (Chem. Abstr., 2005, 142, 440277).
16. D. Davey, P. W. Erhardt, W. C. Lumma, Jr., J. Wiggins, M. Sullivan, D. Pang, and E. Cantor, J. Med. Chem., 1987, 30, 1337. CrossRef
17. Y. Q. Ge, F. R. Li, Y. J. Zhang, Y. S. Bi, X. Q. Cao, G. Y. Duan, J. W. Wang, and Z. L. Liu, Luminescence, 2014, 29, 293. CrossRef
18. A. Kamal, A. V. S. Rao, V. L. Nayak, N. V. S. Reddy, K. Swapna, G. Ramakrishna, and M. Alvala, Org. Biomol. Chem., 2014, 12, 9864; CrossRef A. Kamal, G. Ramakrishna, P. Raju, A. V. S. Rao, A. Viswanath, V. L. Nayak, and S. Ramakrishna, Eur. J. Med. Chem., 2011, 46, 2427. CrossRef
19. K. Brännström and W. J. Arendshorst, Am. J. Physiol., 1999, 276, F758; N. F. Ford, L. J. Browne, T. Campbell, C. Gemenden, R. Goldstein, C. Gude, and J. W. F. Wasley, J. Med. Chem., 1985, 28, 164. CrossRef
20. S. Price, R. Heald, and P. P. A. Savy, 2009, WO 2009085980 (Chem. Abstr., 2009, 151, 123970).
21. L. J. Browne, C. Gude, H. Rodriguez, R. E. Steele, and A. Bhatnager, J. Med. Chem., 1991, 34, 725. CrossRef
22. D. A. Degoey, C. A. Flentge, W. J. Flosi, D. J. Grampovnik, D. J. Kempf, L. L. Klein, M. C. Yeung, J. T. Randolph, X. C. Wang, and S. Yu, US Pat. Appl. Publ., US 2005148623 (Chem. Abstr., 2005, 143, 133693).
23. B. Parton and F. L. Rose, Brit. Pat., 1978, 1 528 801 (Chem. Abstr., 1979, 91, 124859).
24. B. Parton and F. L. Rose, Brit. Pat., 1978, 1 531 752; (Chem. Abstr., 1979, 90, 205761).
25. W. Groß, H. Giesa, and A. Kroos, Deutsche Patent, 2011, DE 10 2010 062 029 A1.
26. R. N. Butler, S. M. Johnston, J. Chem. Soc., Perkin Trans. 1, 1984, 2109. CrossRef
27. M. M. El-Abadelah, A. Q. Hussein, and B. A. Thaher, Heterocycles, 1991, 32, 1879. CrossRef
28. J. E. Baldwin, J. Chem. Soc., Chem. Commun., 1976, 18, 734. CrossRef
29. P. Frohberg, C. Wagner, R. Meier, and W. Sippi, Tetrahedron, 2006, 62, 6050; CrossRef S. Ianelli, G. Pelosi, G. Ponticelli, M. T. Cocco, and V. Onnis, J. Chem. Crystallogr., 2001, 31,149. CrossRef
30. P. P. Fu and R. G. Harvey, Chem. Rev., 1978, 78, 317; CrossRef D. Walker and J. D. Hiebert, Chem. Rev., 1967, 67, 153; CrossRef D. R. Buckle, S. J. Collier, and M. D. McLaws, r-EROS Encyclopedia of Reagents for Organic Synthesis, 2005, DOI: 10.100/047084289s.rd114.pub2. CrossRef
31. R. W. Begland, D. R. Hartter, F. N. Jones, D. J. Sam, W. A. Sheppard, O. W. Webster, and F. J. Weigert, J. Org. Chem., 1974, 39, 2341. CrossRef
32. H. Naeimi, and Z. Babaei, J. Chin. Chem. Soc., 2015, 62, 41. CrossRef
33. T. Sugawara, H. Masuya, T. Matsuo, and T. Miki, Chem. Pharm. Bull., 1979, 27, 2544. CrossRef
34. R. A. Gutiérrez-Márquez, C. Crisóstomo-Lucas, D. Morales-Morales, and S. Hernández-Ortega, Acta Cryst., 2014, E70, m218; J. Elguero, A. Guerrero, F. G. de la Torre, A. de la Hoz, F. A. Jalón, B. R. Manzano, and A. Rodríguez, New J. Chem., 2001, 25, 1050. CrossRef
35. F. F. Awwadi, R. D. Willett, K. A. Peterson, and B. Twamley, Chem. Eur. J., 2006, 12, 8952. CrossRef
36. N. Y. Bamaung, A. Basha, S. W. Djuric, E. J. Gubbins, J. R. Luly, N. P. Tu, D. J. Madar, U. Warrior, P. E. Wiedeman, X. Zhou, R. J. Sciotti, and F. L. Wagenaar, US Pat., 2001, US 2001 / 004445 A1; N. Y. Bamaung, A. Basha, S. W. Djuric, E. J. Gubbins, J. R. Luly, N. P. Tu, D. J. Madar, U. Warrior, P. E. Wiedeman, X. Zhou, F. L. Wagenaar, R. J. Sciotti, 1999, International Patent, 8 April 1999, PCT, US 99 / 07766 and 14 October 1999, WO 99 / 51580.
37. CrysAlis PRO, Version 1.171.35.11 (2011) Agilent Technologies, Yarnton, England.
38. SHELXTL (XPREP, XP, XCIF), Version 6.10 (2002) Bruker AXS Inc, Madison, WI