|
 |
Year : 2012 | Volume
: 6
| Issue : 2 | Page : 51-60 |
|
|
|
|
REVIEW ARTICLE Biologically based strategies to augment rotator cuff tears
M Schaer1, M Schober1, S Berger2, P Boileau3, MA Zumstein4
1 Department of Orthopaedic Surgery and Traumatology, Inselspital, Bern, Switzerland, 2 Department of Orthopaedic Surgery and Traumatology, Inselspital; Institute of Immunology, University of Bern, Bern, Switzerland, 3 Department of Orthopaedic Surgery and Sports Traumatology, University of Nice Sophia Antipolis, Nice, France, 4 Department of Orthopaedic Surgery and Traumatology, Inselspital, Bern, Switzerland; Department of Orthopaedic Surgery and Sports Traumatology, University of Nice Sophia Antipolis, Nice, France,
Correspondence Address: M A Zumstein Orthopedic Sports Medicine, Department of Orthopaedics and Traumatology, University of Bern, Bern, Switzerland
 Source of Support: None, Conflict of Interest: None

|
|
|
|
Date of Web Publication | 7-Jun-2012 |
Abstract | | |
Lesions of the rotator cuff (RC) are among the most frequent tendon injuries. In spite of the developments in both open and arthroscopic surgery, RC repair still very often fails. In order to reduce the failure rate after surgery, several experimental in vitro and in vivo therapy methods have been developed for biological improvement of the reinsertion. This article provides an overview of the current evidence for augmentation of RC reconstruction with growth factors. Furthermore, potential future therapeutic approaches are discussed. We performed a comprehensive search of the PubMed database using various combinations of the keywords "tendon," "rotator cuff," "augmentation," "growth factor," "platelet-rich fibrin," and "platelet-rich plasma" for publications up to 2011. Given the linguistic capabilities of the research team, we considered publications in English, German, French, and Spanish. We excluded literature reviews, case reports, and letters to the editor.
Keywords: Concentrates, growth factor, matrix, platelets, PRF, PRP, rotator cuff, shoulder
How to cite this article: Schaer M, Schober M, Berger S, Boileau P, Zumstein M A. Biologically based strategies to augment rotator cuff tears. Int J Shoulder Surg 2012;6:51-60 |
Introduction | |  |
Lesions of the rotator cuff (RC) are among the most frequent tendon injuries. More than 50% of the population aged 60 years already show lesions of the RC. [1] There is an increase in frequency with cumulative age, but advanced symptomatic lesions can already be found in younger patients. [1] In addition to pain, these lesions may also restrict function. Many of these patients need an operation on the RC. In spite of the developments in both open and arthroscopic surgery, RC repair still very often fails. [2] In order to reduce the failure rate after surgery, several experimental in vitro and in vivo therapy methods have been developed for biological improvement of the reinsertion. This article provides an overview of the current evidence for augmentation of RC reconstruction with growth factors. Furthermore, potential future therapeutic approaches are discussed. We performed a comprehensive search of the PubMed database using various combinations of the keywords "tendon," "rotator cuff," "augmentation," "growth factor," and "platelet concentrate" for publications up to 2011. Given the linguistic capabilities of the research team, we considered publications in English, German, French, and Spanish. We excluded literature reviews, case reports, and letter to the editor.
The Intact Physiological RC Insertion | |  |
A physiological RC insertion consists of a transition between bone and tendon. This area contains the following four zones: [3],[4],[5]
Zone I: Tendon
Zone II: Unmineralized fiber cartilage
Zone III: Mineralized fiber cartilage
Zone IV: Bone
Collagen I dominates zones I and IV, while zones II and III mainly contain collagens I, II, and X, as well as extracellular matrices with proteoglycans, such as decorin, aggrecan, and biglycan. [3],[6],[7] During the aging process of intact tendons, vascularization decreases around the RC insertion. [8],[9],[10]
Etiology and Histopathology of the RC Lesion | |  |
A distinction is now made between extrinsic and instrinsic causes of RC lesion. An extrinsic cause may be a traumatic tensile load on the RC tendon. Neer first published the extrinsic cause for tendon degeneration in 1972. He described subacromial impingement [11] resulting in extra-articular damage of the tendon, caused by the coracoacromial curve during movements of the upper arm. Intrinsic factors for an RC lesion are found within the RC itself. This is described in a degenerative microtrauma model. [12] Age-related degeneration leads to increased mucoid integration, as well as to hydroxyapatite microcalcification and fatty infiltration. [13]
These changes lead to impairment of the tendon and favor microtraumas, leading to partial and eventually total RC rupture. This process is accelerated by genetic predisposition and metabolic dysfunction. [14] Yuan et al. showed that in RC tendinopathy, a significantly higher proportion of cells with signs of apoptosis (34%) are located at the end of the tendon, as compared to those of healthy patients (13%). Apoptotic cells are found not only in the ruptured tendon, but also in the perivascular areas. [15] Nevertheless, there is no evidence for a correlation between the number of apoptotic cells, the duration of the symptoms, and the age or the size of the rupture. The cause of apoptosis is not yet understood. Possible causes include ischemia, hypoxia, or free radicals. Apoptosis is associated with decreased collagen synthesis and may be one of the intrinsic causes of tendon degeneration.
Furthermore, the RC with tendinopathy frequently exhibits edema in the extracellular matrix, with fragmentation and disorientation of the collagen fibers. [14]
Precise knowledge of the individual anatomical structures is essential if the function of the muscle-tendon unit is to be restored. The limiting factors for the function and outcome of an RC reconstruction are
- the quality of the muscle and
- the integrity of the tendon-bone interface of the repaired tendon.
Biology of RC muscle healing
The quality of muscles and tendons is mainly influenced by the content of fat and connective tissue. [16] After RC rupture, the muscle tends to retract and to become atrophic. Intramuscular connective tissue may increase and the number of capillaries may decrease. [17] The muscle fibers become shorter, the pennation angle increases, and the muscular tissue partially loses its ability to develop tension. In the magnetic resonance image (MRI), the progress of fatty infiltration can be evaluated and its extent measured. [18],[19],[20] The amount of fatty infiltration is a predictor of the probability that ruptured RC may heal, and therefore substantially influences the clinical outcome of the reinsertion. [21],[22] The architectural and histomorphometric consequences of the retraction of the musculotendinous unit cannot be reversed over time. [20],[23],[24],[25] The inelastic and retracted muscle and tendon cannot be brought back by a single operation to its original site of insertion, [16],[24] without further structural damages leading to an increase in connective tissue. [25]
In parallel to the architectural and histomorphometric deterioration, molecular biological analyses have shown an increase in the expression of "alpha-skeletal muscle actin" and "myosin heavy polypeptide-1" with increasing fatty infiltration in RC muscles. [26] This increase correlates with raised tissue metabolism and an increase in oxidative stress. This finding supports the histological and electron microscopic results, [24] providing evidence of an association between the amount of fatty tissue and attempted maximal regeneration.
The atrophy of a muscle is accompanied by fatty infiltration. [27] This atrophy is induced by decreased protein synthesis or increased protein degradation. Various signal proteins and transcription factors regulate muscle atrophy through the Forkhead box O (FOXO) factor, which stimulates atrogin-1 and Muscle RING-finger protein-1 (MURF1) and thereby induces proteolysis through three proteolytic systems (1. calcium-dependent calpain system, 2. the lysosomal protease system (cathepsin), and 3. the ubiquitin-proteasome system). At a molecular level, all three systems exhibit much greater up-regulation in massive RC ruptures than with smaller or absent ruptures. [28]
Biology of tendon-bone interface healing
There is enough experimental and clinical evidence to demonstrate that optimal mechanical fixation - with adequate suture material, suture anchorage, and tendon to bone fixation - is related to higher healing rates. [29] Suture techniques with high tensile strength and tendon anchorage techniques have been evolved in in vitro and in vivo studies. [29] Experimental data have shown that healing is initiated mainly by cells that originate from the osseous footprint [30] and the bursa. [31]
In the fetal development of the RC insertion, the above-named four zones are formed, whereas after an RC lesion, poor quality scar healing takes place in the tendon-bone interface. In comparison to the physiological tendon-bone interface, the regenerative disorientated scar tissue does not show the four typical zones and is more susceptible to mechanical failure. [6],[32] Because current approaches do not lead to physiological restoration of the RC insertion and the conditions at the distal end of the RC tendon and of the bone insertion are suboptimal, [33] new biological treatment strategies are indispensable. These strategies should create an optimized environment for the restoration of a physiological musculotendinous unit.
The prerequisites for healing are:
- cells of the local surrounding area,
- collagen fibers and other extracellular matrix (ECM) proteins of the natural insertion,
- stable three-dimensional matrices as carriers for regenerative cells and growth factors, and
- cytokines, modulators, and growth factors over a period of several weeks.
The role of cells on tendon-bone healing
Cells inducing the healing of RC lesions are either intrinsic cells originating from the insertion area (osteoblasts, tenocytes of the tendon and the peritendineum [31] ), or extrinsic cells such as inflammatory cells (granulocytes), and platelets and stem cell precursors from the bursa or the bone marrow. [34],[35] According to the studies of Uhthoff et al., [36] the fibroblasts of the proximal tendon stump make only a minor contribution to the healing. The cells involved in the healing process originate from the proximal humerus as well as from the surrounding bursa. [31] After a reinsertion, the osteoblasts proliferate and new trabeculae are formed. [36] The spindle-shaped tenocytes in zones I and II at the merging area to the tuberculum majus synthesize and secrete ECM proteins. [37] Because these cells exhibit very low proliferative capacities, [38] one possible approach to improve tendon healing is to enhance their activity by adding growth factors.
Moreover, platelets and inflammatory cells like leucozytesare involved in every healing process. In the initial stage of the tendon repair, they act by secreting growth factors, which initiate the repair cascade. [39]
Under the influence of endogenous and exogenous factors, stem cells can subsequently differentiate into the appropriate cell type. Allogeneic bone marrow stromal cells were able to differentiate into tenocyte-like cells within 5 weeks of implantation into a rabbit patellar tendon. However, the numbers of these cells decreased with time. [40] The necessary precursor stem cells can be isolated during arthroscopic refixation from the proximal humerus. [35] Gulotta et al. [41] demonstrated in a rat model that bone marrow derived mesenchymal stem cells transformed with the protein scleraxis, a transcription factor, improved RC healing. However, further studies are needed to confirm these results.
The role of ECM proteins on tendon-bone healing
The proteins of the ECM form all macromolecules outside the plasma membrane of cells and serve primarily to fix the cells embedded in the ECM. Because the cells influence each other, as well as the ECM, a dynamic instead of a static situation has to be assumed. The ECM consists of collagen fibers, as well as glycosaminoglycans and proteoglycans. [42]
The role of collagens in tendon-bone healing
There are different types of collagen in each of the four zones. In the areas of the insertion of the tendon to the bone (zones II and III), the organization of the collagen fibers is less parallel than in the tendon itself. [43] Collagen can be divided into three groups: fibrillar types (I, II, and III), fibril-related types (IX, XII), which serve as bridges between fibrils and ECM proteins, and short-chained collagen types (X). [5]
- Type I collagen is found mainly in zones I and IV [6] as well as temporarily in the stage of remodeling. [44]
- In contrast, type II collagen is found during fetal development in zone IV and postnatally in zones II and III. With the completion of the development of the tendon insertion, collagen type II can only be detected in zone III. [6]
- Type III collagen is situated in the tendon as well as in the bone, [45] and is already present during the early stages of the healing process. [46] In the healing of tendons and bone, collagen type III is associated with degenerative and scar tissue. [46,47] It is not yet clear to what extent excessive levels of collagen III can impair insertion quality.
- Type V collagen is a regulator of the fibril diameter of collagen type I, [46] and therefore can be found predominantly in zones I and II. [5]
- Type IX collagen is located predominantly on the osseous side of the insertion. It belongs to the fibril-related types and connects mainly with type II collagen.
- Collagen type X is produced during human development by hypertrophic cartilage corpuscles in the mineralized fibrocartilaginous transitional zone (zone III) and appears in the epiphyseal plate during growth. This is interesting because growth and transcription factors, which contribute to the maturation of the epiphyseal plate, influence the development of the insertion. [5,6] Collagen X persists in zone III, even if the hypertrophic chondrocytes are later absent. This indicates that collagen X plays an important role in the transformation of unmineralized to mineralized tissue. However, collagen X is absent in adult tendon to bone healing. [5],[6] Interestingly, collagen type X is also absent during the embryonic and neonatal stage and is not produced until zones II and III have been developed. Therefore, mechanical factors possibly contribute to the expression and production of collagen X. [6] This is supported by the fact that the formation of a fibrocartilaginous transitional zone is disturbed in tendons of patients with paralyzed shoulders. [48]
- Collagen type XII exists on both sides of the insertion. [49] Collagen XII is situated on both sides of the insertion and belongs, like collagen type IX, to the fibril-associated group. It binds to collagen type I. [5]
The role of proteoglycans in tendon-bone healing
Different proteoglycans contribute to the tissue regulation of the ECM. One of the proteoglycans is decorin. Similar to type V collagen, decorin regulates the fibril thickness of the collagen and is found exclusively in the tendinous area of the transition zone, mainly in zone I and II of the intact insertion. [5],[46],[49] Decorin is at reduced levels during the healing process, but can still be detected. Expression of decorin indicates that specific tendon tissue is formed. [5] Furthermore, it contributes to the stabilization of the collagen structure by building bridges between collagen fibrils and by influencing fibrillogenesis through inhibition of collagen type I formation. [5],[49] It also regulates the activity of transforming growth factor-beta (TGFb1). [5]
Aggrecan is a cartilage-specific ECM protein analogous to versican. It is only common in the osseous region of the insertion. [5],[7],[45] Compressive stress triggers the production of aggrecan, [6] which is why this protein is predominant in zone III. [5] Aggrecan has a huge potential to bind water. [5]
Biglycan is a tendon-specific ECM protein that is only located in zone I of the insertion area. [5],[7],[45] Like decorin, biglycan forms bridges between the collagen fibrils and increases their stability. It also determines their structure by influencing fibrillogenesis. [49]
Stable 3D matrix for tendon-bone healing
The first matrix which is developed during wound healing is the blood clot. Its formation results in hemostasis. Together with the primary polymerized fibrin structure, it serves as a temporary matrix for cell migration. The platelets within the blood clot secrete the first growth factors. [39] Five days after injury, fibroblasts migrate along the fibrin to the site of the lesion. They replace fibrin with collagen type I and other ECM proteins. [39] This results in the transformation of the fibroblasts into myofibroblasts and is accompanied by contraction, as soon as their pseudopodia touch ECM proteins such as fibronectin or collagen. After this process is complete, apoptosis of the myofibroblasts follows. [39]
Because the matrix is not able to carry mechanical load, different so-called biological scaffolds have entered the market in recent years. These three-dimensional scaffolds act as a temporary mechanical support and are either metabolized or incorporated and remodeled during the healing process. Therefore, they must be absorbable and/or have to be made of natural and relatively inert materials (dermis, intestinal submucosa). Commercially available scaffolds are used either as interposition scaffolds (in between the tendon end and the greater tuberosity) or as augmentation scaffolds (to augment the tendon substance). In an in vitro study, Shea et al. showed that human tenocytes reacted more favorably to dermal ECM samples without chemical cross-links. Less favorable responses were seen after culture with equine or synthetic ECM. [50] Commercially available scaffolds are still very expensive (1000-2000€) and their role in augmenting RC repair is rather small. Clinical results show a high failure rate and a high rate of clinical complications, including chronic inflammation. [51],[52]
The role of matrix metalloproteinases and tissue inhibitors of metalloproteinase in tendon to bone healing
Aside from growth factors, there is increasing interest in the role of matrix metalloproteinases (MMPs) in the healing process of the RC.
MMPs are zinc-dependent endopeptidases and belong to the family of the proteases. They shape the ECM and connective tissue and are capable of degrading all the components of the ECM, such as collagen and elastin. Normally, there is equilibrium between the MMPs and the natural inhibitors like tissue inhibitors of metalloproteinase (TIMPs). An imbalance leads to elevated MMP activity and can cause degeneration of tendon and RC tears.
Choi et al. [53] demonstrated that MMP-2 is expressed and activated during the healing process of an acute supraspinatus tendon tear, and Lo et al. [54] found statistically significant alterations in the mRNA levels of MMPs and TIMPs in patients with full-thickness RC tears. Another study has shown an increase in the expression of MMP-1 and MMP-3 in the synovial fluid of patients with RC tears, which correlates with tear size. [55] These studies suggest that reducing the MMP levels during the healing process may reduce tissue degradation and favorably influence healing after RC repairs.
This was confirmed in a study in which MMPs were inhibited experimentally by the application of doxycycline. This improved the biomechanical quality of the scar tissue. [56] The MMPs can also be inhibited by α 2 -macroglobulins, which results in better collagen organization, increased fiber cartilage formation, and decreased collagen degradation. However, these results also showed that there was no increase in the load-to-failure or stiffness. [57]
There is little information on the influence of MMPs on neo-vascularization. The investigations on membrane type 1 MMP (MT1-MMP) seem to be very interesting. MT1-MMP plays a decisive role in the embryonic development of musculoskeletal tissue and seems to have an influence on endochondral ossification. It has been shown in several studies to play a role in the embryonic development of musculoskeletal tissues and is up-regulated in areas of chondrocyte differentiation. [58],[59],[60] The second positive effect of MT1-MMP is the up-regulation of cyclooxygenase-2 (= COX-2), which facilitates regeneration and is known to have a crucial effect on bone-tendon reintegration. [61] This is the object of intense research.
The TIMPs are natural inhibitors of the MMPs, which comprise a family of four protease inhibitors: TIMP1-4. These TIMPS are known to bind and inhibit MMPs. TIMP2-4 seem to be decreased in torn RC tendons, leading to an increase in MMP activity. [54]
It is important to note that even though MMPs and TIMPs are involved in degradation of ECM, degradation is an important part of the healing process of tendons.
The role of growth factors in tendon to bone healing
Growth factors can be produced from intrinsic and extrinsic cells. [44] Their activity is dependent on binding to specific receptors. [44] Growth factors appear at the proximal site of the myotendinous insertion earlier than at the distal defect of the tendon, although they are detectable in the lesion for longer periods. [33] Their initial absence may be linked to an underperfusion of the critical zone. [33]
Würgler-Hauri et al. [62] showed that most growth factors returned to low levels after only 4 weeks. The only factors still exhibiting marked up-regulation are those which are typical for the remodeling phase of tendon tissue. This is explained in detail below. This may be linked to the elevated load on the tendon at that time and would support the concept of the influence of mobilization and load on the tendon-bone healing. [62]
In the tendon-bone healing process, the most important growth factors are the following.
Basic fibroblast growth factor
Basic fibroblast growth factor (bFGF) is a member of the fibroblast growth factor (FGF) family and is composed of polypeptides. The FGFs are a heparin-binding protein family, which interact with cell surface associated heparan sulfate proteoglycans.
bFGF is produced not only by leukocytes but also by tenocytes and fibroblasts. [34],[44] This factor remains highly up-regulated during the whole healing process and has its peak between the seventh and the ninth day. bFGF is particularly important in the proliferation and remodeling phases. [33],[44],[62] It is a potent stimulator of angiogenesis and fibroblast proliferation. [34],[44],[62] It also stimulates collagen III production, the secretion of collagenase by fibroblasts, and the proliferation of endotheliocytes, which are important factors for angiogenesis. It also inhibits production of type I procollagen. [34],[44] There is a second peak around the eighth week, which might be a sign of further tenocyte proliferation. [62]
Takahashi et al. showed in 2003 that bFGF stimulates the proliferation of RC tendon cells (RCC) in a dose-dependent manner and suppresses the secretion of collagens from RCC in vitro. [63]
In two studies published in 2009, the initial tendon-to-bone remodeling was accelerated by local application of bFGF. [64],[65] In an intrasynovial flexor tendon canine model, Thomopoulos et al. showed not only increased vascularity, cellularity, and adhesion, but also an increase in peritendinous scar formation. The administration of bFGF failed to produce improvements in either the mechanical or the functional properties of the repair. [66]
Insulin-like growth factor-1
Insulin-like growth factor-1 (IGF-1), also known as somatomedin C, belongs to the insulin-like growth factor family. This family has sequence homology with insulin. IGF-1 interacts with the membrane-bound IGF receptor. IGF-1 is secreted mainly by stimulated keratinocytes, osteoblasts, and fibroblasts. [34] This growth factor is secreted immediately after a lesion has formed. This fact suggests that IGF-1 originates from blood. [33] This growth factor peaks after 3 weeks [44] and is important in all healing phases, particularly during inflammation, proliferation, and remodeling. [33],[44] It inhibits the migration of the inflammatory cells in the inflammatory phase by negative feedback. [44] Together with platelet-derived growth factor-BB PDGF-BB, its main role in tendon healing is the stimulation of the proliferation and migration of fibroblasts and other local cells. [33],[34],[44] During the remodeling phase, IGF-1 raises the production of collagen and other ECM proteins. [44] Extracellular IGF-1 is bound to proteins and therefore exhibits an extracellular memory. It thus permits an immediate response to an event. [33],[44] Dines et al. used rat tendon fibroblasts that were cultured and transfected with the gene of IGF-1. The in vivo test showed that toughness and maximum load to failure were both statistically significantly improved in these repairs. [67]
Platelet-derived growth factor
Platelet-derived growth factor (PDGF) is composed of a dimeric glycoprotein and contains five different isoforms. It is a potent mitogen for cells of mesenchymal origin. The receptor for PDGF, PDGFR, is classified as a receptor tyrosine kinase.
In wound healing, PDGF is synthesized and secreted not only by platelets, but also by macrophages, endotheliocytes, fibroblasts, and keratinocytes. [34] Together with IGF-1, it is one of the first growth factors next to the lesion. [34]
In the early phases of the healing process, PDGF plays an important role by stimulating and regulating the synthesis of other growth factors (e.g. IGF-1, TGF- β1). [33],[44],[62] In a rat patellar tendon defect model, additional PDGF was added on day 3, which resulted in increased proliferation in tendons. Addition on day 7 improved the peak load, the cross-sectional area, and the pyridinoline content. [68] At later times, PDGF influenced remodeling by enhancing the production of collagen (type I) and non-collagen proteins (e.g. fibronectin), [34],[44],[62] and the migration and proliferation of fibroblasts and endotheliocytes. [34] In a rat model of RC repair, delivery of cells expressing PDGF- b0 with a polyglycolic acid scaffold restored normal crimp patterning and collagen bundle alignment compared to suture repair only. [69] Uggen et al. coated FibreWire sutures with recombinant human PDGF-BB and used this suture for RC tendon to bone repair with an anchor. After a short-term follow-up, histological analysis showed improved tendon-to-bone healing. However, ultimate load to failure was equivalent to standard suture repairs. [70]
Vascular endothelial growth factor
Vascular endothelial growth factor (VEGF) stimulates vasculogenesis and angiogenesis. It is a sub-family of the PDGF family. The most important member is VEGF-A.
VEGF is secreted by neutrophilic granulocytes, platelets, keratinocytes, tenocytes, and astrocytes. [34] The production and secretion of VEGF is stimulated by other growth factors (PDGF, bFGF, TGF-β), interleukins, and hypoxia. [34],[44] The VEGF level has its peak on day 7, and from then on, it decreases until the 21st day. [44] VEGF is active during inflammation, proliferation, and remodeling phase and acts as a stimulator of the angiogenesis of epi- and intratendinous vessels. [34],[44] It stimulates the secretion of other growth factors such as bFGF. [34] No studies have directly evaluated its role in RC reconstruction. Those studies that evaluated the effects of locally applied VEGF on anterior cruciate ligament ACL - and Achilles tendon-reconstruction are inconsistent. [71],[72]
Transforming growth factor-β
The protein TGF-β exists in three isoforms (TGF-β1, TGF-β2, and TGF-β3). It is part of the transforming growth factor beta superfamily, which includes inhibin, activin, and bone morphogenetic protein (BMP).
Normal ligaments and tendons show low staining of TGF-β. During the healing process, practically all the cells that are involved in the healing process increasingly produce TGF-β. [34],[44] Together with IGF-1, it is very rapidly released at the site of the lesion. This suggests that TGF-β originates in blood. [33] TGF-β1 remains highly up-regulated for the first 8 weeks [44],[62] and is active in the tendon healing process during all phases. [33],[44] TGF-β1 enhances migration of extrinsic cells, the regulation of protease activity, the transcription of fibronectin, the termination of cell proliferation, and the activation of fibroblasts by PDGF-AB. It also stimulates collagen production (I and III). [34],[44],[62] However, one study reports that the application of TGF-β to a torn tendon increased proliferation without increasing tendon strength. [4]
TGF-β3 plays a decisive role in the scarless fetal healing, but it is undetectable in scar formation in adults. [6] Treatment with TGF-β3 in vivo accelerated the healing process, with increased vascularity, inflammation, cellularity, and cell proliferation at early time points. Moreover, sustained delivery of TGF-β3 to the healing tendon-to-bone insertion led to significant improvements in structural properties at 28 days and in material properties at 56 days compared to controls. [73] TGF-β3 could therefore be an important factor for the regeneration of a bone-tendon insertion in the future.
Bone morphogenetic protein 2, 7, 12, 13, 14 (BMP-2, -7, -12, -13, -14)
There are a number of BMPs. Except BMP-1, all BMPs belong to the superfamily of TGF-β. BMP-12 and -13 are expressed by fibroblasts at the tendon insertion sites and induce the formation of tendon and fibrocartilage. A study by Seehermann et al. [74] showed that recombinant human BMP-12 in a hyaluronic sponge had 2.1 times greater maximal load than the untreated repairs in a sheep model. RhBMP-12 in a collagen types I and III sponge gave a relative increase of 2.7-fold in the maximal load. However, the mechanical properties of the tissues were similar in both augmented and control groups of the study when normalized to the size of the tendon. [74]
In 2011, Gulotta et al. [41] showed in a rat model that the application of mesenchymal stem cells which had been genetically modified to overexpress BMP-13 in a tendon-to-bone repair did not improve healing. [75] In contrast, supraspinatus tendon repairs (tendon-to-tendon) treated with BMP-13 in 48 rats were significantly stronger than the untreated repairs and histological analysis showed more organized healing after 4 and 6 weeks. [76]
In an in vitro study on tenocyte-like cells isolated from human RC tissue samples, BMP-2 increased collagen type I production. An increase in collagen type-1 production and expression, as well as increased cell activity, was observed for BMP-7. Adding BMP-2 and -7 resulted in smaller changes than seen with BMP-7 alone. [77] The application of BMP-2 in an injectable hydrogel into a ruptured RC gave a significantly higher maximum pull-out load at 4 and 8 weeks postoperatively. [78]
Despite the potential advantage of the application of a single growth factor, the healing of the RC involves the long-term interaction between several of these growth factors, with a fixed sequence of peak concentrations. [33]
Combining different growth factors for tendon-bone healing
Several growth factors have been tested independently for their contribution to RC healing.
In the physiological healing process, different growth factors work together to create appropriate tendon-to-bone healing. Newer approaches tend to apply matrixes that are loaded with different growth factors. [67],[79],[80] These therapy forms are known as "tissue engineering" and contain scaffolds, regulators, and cells, which could possibly lower the failure rate after reconstruction of the RC. There is little published evidence on the combination of different growth factors in RC repair. In a sheep infraspinatus tear model, Rodeo [81] implanted growth factors from bovine cortical bone extract (BMP-2 to BMP-7, TGF-β1 to TGF-β3, and FGF) with a type I collagen sponge into a healing RC of 72 mature sheep. After 6 and 12 weeks, the investigators found significantly greater failure loads with increased bone volume and soft tissue volume in the augmented group when compared to non-augmented controls. However, when the loads were normalized for tissue volume, they found no differences between treated and untreated shoulders. These data suggest that the combination of growth factors did not improve the quality of the repair, but might accelerate the healing processes, which is particularly desirable in sports medicine.
Platelet-rich concentrates from centrifuging autologous blood can also be used as a source of different growth factors for biological augmentation of RC repair. These concentrates contain high concentrations of platelets that undergo degranulation and release of growth factors. Once activated, platelet-rich concentrates, such as platelet-rich plasma (PRP) and leukocyte- and platelet-rich fibrin (L-PRF), provide the best possible conditions for restoration of the RC:
- continuous release of anabolic stimulative growth factors,
- high levels of platelets and leukocytes, and
- a stable temporary matrix.
Platelet-Rich Plasma as a Therapy Approach | |  |
There has been much discussion on the possible use of platelet concentrates like PRP for RC refixation. [82],[83] There are different kinds of PRPs, which differ in the manner of their production, as well as in the concentration of the leukocytes [leukocyte- and platelet-rich plasma (L-PRP) and pure platelet-rich plasma (P-PRP) without leukocytes] and the stability of the fibrin matrix. [84],[85] PRP is a concentrate rich in platelets and provides many growth factors locally. The problem with all PRPs is that the growth factors are applied in very high doses for a very short period of time. Excessive concentrations of growth factors can, in principle, activate a negative feedback loop, down-regulate receptors on the target cells, and cause the opposite effects to those desired. Further, the P-PRPs do not contain leukocytes, which are important for the healing of the RC. Leukocytes can produce and secrete growth factors, particularly VEGF, for a longer period of time. However, both PRPs lack a stable three-dimensional matrix because the secondary polymerization only leads to bilateral and not equilateral polymerization. Because the PRP matrixes disintegrate faster, they can be for only a short time span as a temporary matrix between tendon and bone. [85] In a randomized double-blind study, Randelli et al. [86] showed that pain after 2 years was lower in the L-PRP group after an arthroscopic RC repair in comparison to the control group. They also reported that the strength in external rotation was better with small ruptures after 3 months follow up. Constant score, simple shoulder test (SST), and subjective shoulder value (SSV) were raised. On the other hand, Castricini et al. [88] performed a randomized controlled trial of an arthroscopic RC repair on 43 patients with small or medium RC tears, using PRP as biological augmentation. In the follow-up after 16 months, there was no significant difference in total constant score when compared to the group of 45 patients who did not receive biological augmentation. They did not evaluate whether the augmentation with PRP accelerated the healing process or whether PRP-clot releasates are beneficial for large and massive RC tears. Due to the heterogeneity of PRP preparation, it is also possible that other preparations may be more effective.
In a case report, [89] PRP was sutured side-by-side to close a massive RC tear. Six months after surgical repair, an MRI showed the complete integrity of the cuff under the PRP membrane. Further studies are needed to prove the potential of PRP in RC healing.
Leukocyte- and Platelet-Rich Fibrin as a Therapy Approach | |  |
L-PRF consists of a stable fibrin network, rich in platelets and leukocytes, in which the cells are not damaged. [84] L-PRF can be easily produced without additives, from the patient's own blood, so that no foreign body reaction is expected. The production is not expensive and is simple. As the preparation time is only 20 minutes, L-PRF can be produced easily during surgery [Figure 1]a, b. If the blood is centrifuged slowly, a matrix is generated, which is optimal for cell migration and cell proliferation by means of primary polymerization. [90] In an as yet unpublished study, we could show that L-PRF is able to release growth factors in vitro over several weeks [Figure 2]a, b. [83] L-PRF therefore exhibits the qualities required for a physiological restoration.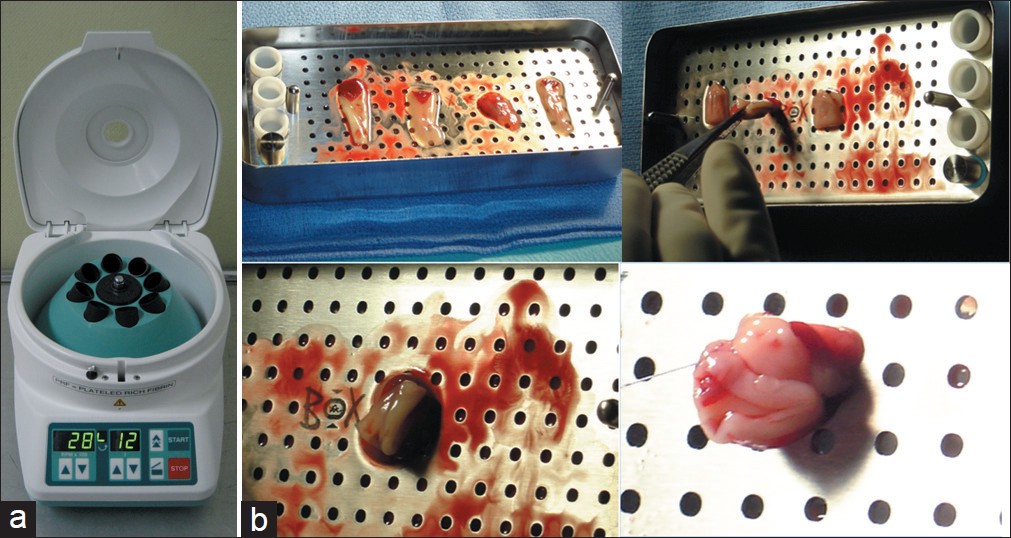 | Figure 1: (a) Preparation of L-PRF: A blood sample is taken without anticoagulants in 10 ml tubes which are immediately centrifuged at 400g for 12 minutes. (b) After the centrifugation, the red layer is removed and the L-PRF is folded, stacked, and fixed with an absorbable 4-0 PDS suture
Click here to view |
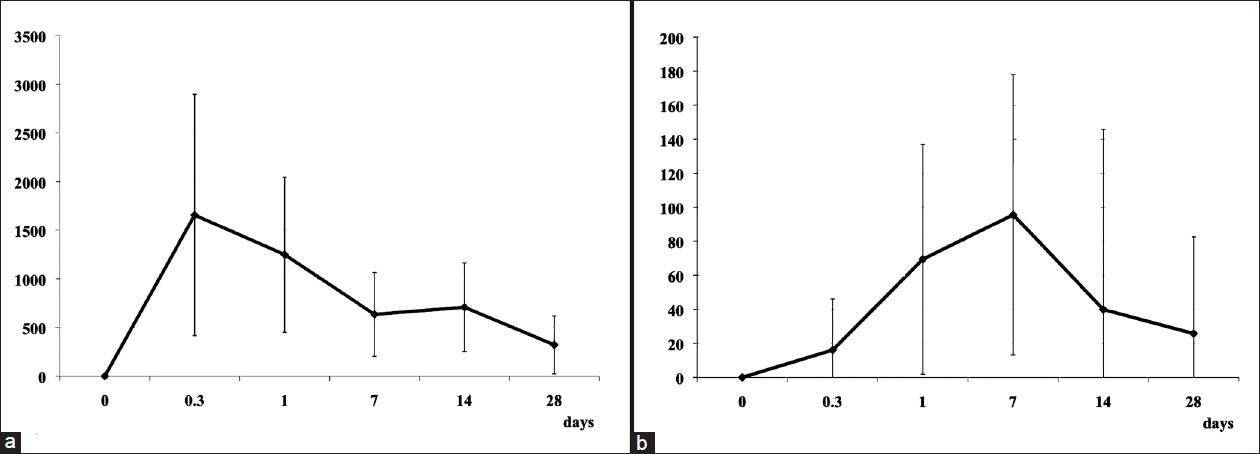 | Figure 2: (a) Release of PDGF in L-PRF clots: Kinetics of the release was significantly increased between 0.3 and 7 days and then decreased closer to time 0 levels at 28 days. (b) Kinetics of the release of VEGF was significantly increased between 0.3 and 7 days and then decreased closer to time 0 levels at 28 days
Click here to view |
In an ongoing prospective randomized study in the Department of Orthopedic Sports Traumatology in Nice, France, an arthroscopic transoseous equivalent double-row-repairs in cronic rotator cuff tears were performed. During the conventional preparation, the L-PRF is produced in the operation room. After its production, it is folded, stacked, and fixed with an absorbable thread.
After placing the medial anchor, a guidance thread is introduced medial to the anchor and the L-PRF is brought in position lateral to the anchor. This is a reservoir of growth factors and a matrix between the tendon end and the osseous insertion. Then, the tendon is fixed as usual with a lateral anchor and is closed. The excess of the matrix production is injected with a syringe directly into the L-PRF.
The first postoperative examinations of 20 arthroscopic double-row RC refixations (10 patients in the PRF group, 10 patients in the control group) showed a significant increase in the vascularization in the area of reinsertion in the PRF group after 6 weeks, which could have led to accelerated rehabilitation. [91] No difference between the two groups in the vascularization level could be found after 3 months. However, the impact of PRF on the RC healing process needs to be confirmed with larger controlled clinical trials.
Concluding Remarks | |  |
The incidence of degenerative RC tears is increasing as the population ages. Failure to heal and retearing after surgery is still a common problem, since the four fibrocartilaginous transition zones of the RC insertion site are not restored properly following surgical repair. It is rather the case that less stable scar tissue bridges the torn tendon to bone insertion. There seems to be a great potential to enhance RC tendon healing using biological agents such as growth factors and modified stem cells.
Studies have shown that different growth factors are capable of increasing the strength of repairs in animal models. Unfortunately, this seems to be accomplished through an increase in scar tissue production instead of by forming a physiological tendon-to-bone insertion. The optimal timing, concentration, and combination of the different growth factors is still unclear. As in transduced and modified stem cell applications, the optimal dosage and vehicle for delivery has remained elusive. Modified stem cells and growth factors for RC repairs are promising therapeutic agents for the future. The use of leukocyte and platelet concentrates seems to be a promising approach to biological augmentation of RC tears, as these concentrates contain many of the fundamental growth factors at high dosages, can release the growth factors over time, and can be a potential carrier for stem cell application. Further research is needed to define the correct dosage, quantification, and timing, and to fully understand the teamwork between modified stem cells and different growth factors.
References | |  |
1. | Sher JS, Uribe JW, Posada A, Murphy BJ, Zlatkin MB. Abnormal findings on magnetic resonance images of asymptomatic shoulders. J Bone Joint surg Am 1995;77:10-5.  |
2. | Duquin TR, Buyea C, Bisson LJ. Which method of rotator cuff repair leads to the highest rate of structural healing? A systematic review. Am J Sports Med 2010;38:835-41.  |
3. | Woo SL, Hildebrand K, Watanabe N, Fenwick JA, Papageorgiou CD, Wang JH. Tissue engineering of ligament and tendon healing. Clin Orthop Relat Res 1999(367 Suppl):S312-23.  |
4. | Galatz LM, Sandell LJ, Rothermich SY, Das R, Mastny A, Havlioglu N, et al. Characteristics of the rat supraspinatus tendon during tendon-to-bone healing after acute injury. J Orthop Res 2006;24:541-50.  |
5. | Thomopoulos S, Hattersley G, Rosen V, Mertens M, Galatz L, Williams GR, et al. The localized expression of extracellular matrix components in healing tendon insertion sites: An in situ hybridization study. J Orthop Res 2002;20:454-63.  |
6. | Galatz L, Rothermich S, VanderPloeg K, Petersen B, Sandell L, Thomopoulos S. Development of the supraspinatus tendon-to-bone insertion: Localized expression of extracellular matrix and growth factor genes. J Orthop Res 2007;25:1621-8.  |
7. | Vogel KG, Sandy JD, Pogany G, Robbins JR. Aggrecan in bovine tendon. Matrix Biol 1994;14:171-9.  |
8. | Adler RS, Fealy S, Rudzki JR, Kadrmas W, Verma NN, Pearle A, et al. Rotator cuff in asymptomatic volunteers: contrast-enhanced US depiction of intratendinous and peritendinous vascularity. Radiology 2008;248:954-61.  |
9. | Lohr JF, Uhthoff HK. The microvascular pattern of the supraspinatus tendon. Clin Orthop Relat Res 1990;254:35-8.  |
10. | Rudzki JR, Adler RS, Warren RF, Kadrmas WR, Verma N, Pearle AD, et al. Contrast-enhanced ultrasound characterization of the vascularity of the rotator cuff tendon: age- and activity-related changes in the intact asymptomatic rotator cuff. J Shoulder Elbow Surg 2008;17(1 Suppl):96S-100S.  |
11. | Neer CS 2 nd . Impingement lesions. Clin Orthop Relat Res 1983;173:70-7.  |
12. | Yadav H, Nho S, Romeo A, MacGillivray JD. Rotator cuff tears: pathology and repair. Knee Surg Sports Traumatol Arthrosc 2009;17:409-21.  |
13. | Hashimoto T, Nobuhara K, Hamada T. Pathologic evidence of degeneration as a primary cause of rotator cuff tear. Clin Orthop 2003(415):111-20.  |
14. | Matthews TJ, Hand GC, Rees JL, Athanasou NA, Carr AJ. Pathology of the torn rotator cuff tendon. Reduction in potential for repair as tear size increases. J Bone Joint Surg Br 2006;88:489-95.  |
15. | Yuan J, Murrell GA, Wei AQ, Wang MX. Apoptosis in rotator cuff tendonopathy. J Orthop Res 2002;20:1372-9.  |
16. | Gerber C, Meyer DC, Schneeberger AG, Hoppeler H, Von Rechenberg B. Effect of tendon release and delayed repair on the structure of the muscles of the rotator cuff: An experimental study in sheep. J Bone Joint Surg Am 2004;86-A:1973-82.  |
17. | Jozsa L, Kannus P, Thoring J, Reffy A, Jarvinen M, Kvist M. The effect of tenotomy and immobilisation on intramuscular connective tissue. A morphometric and microscopic study in rat calf muscles. J Bone Joint Surg Br 1990;72:293-7.  |
18. | Fuchs B, Weishaupt D, Zanetti M, Hodler J, Gerber C. Fatty degeneration of the muscles of the rotator cuff: assessment by computed tomography versus magnetic resonance imaging. J Shoulder Elbow Surg 1999;8:599-605.  |
19. | Goutallier D, Postel JM, Bernageau J, Lavau L, Voisin MC. Fatty muscle degeneration in cuff ruptures. Pre- and postoperative evaluation by CT scan. Clin Orthop 1994;304:78-83.  |
20. | Meyer DC, Hoppeler H, von Rechenberg B, Gerber C. A pathomechanical concept explains muscle loss and fatty muscular changes following surgical tendon release. J Orthop Res 2004;22:1004-7.  |
21. | Jost B, Zumstein M, Pfirrmann CW, Gerber C. Long-term outcome after structural failure of rotator cuff repairs. J Bone Joint Surg Am 2006;88:472-9.  |
22. | Zumstein MA, Jost B, Hempel J, Hodler J, Gerber C. The clinical and structural long-term results of open repair of massive tears of the rotator cuff. J Bone Joint Surg Am 2008;90:2423-31.  |
23. | Bjorkenheim JM. Structure and function of the rabbit's supraspinatus muscle after resection of its tendon. Acta Orthop Scand 1989;60:461-3.  |
24. | Gerber C, Meyer DC, Frey E, von Rechenberg B, Hoppeler H, Frigg R, et al. Neer Award 2007: Reversion of structural muscle changes caused by chronic rotator cuff tears using continuous musculotendinous traction. An experimental study in sheep. J Shoulder Elbow Surg 2009;18:163-71.  |
25. | Stauber WT, Miller GR, Grimmett JG, Knack KK. Adaptation of rat soleus muscles to 4 wk of intermittent strain. J Appl Physiol 1994;77:58-62.  |
26. | Fuchs B, Zumstein MA, Regenfelder F, Steinmann P, Fuchs T, Husmann K, et al. Upregulation of α-skeletal muscle actin and myosin heavy polypeptide gene products in degenerating rotator cuff muscles. J Orthop Res 2008;26:1007-11.  |
27. | Rowshan K, Hadley S, Pham K, Caiozzo V, Lee TQ, Gupta R. Development of fatty atrophy after neurologic and rotator cuff injuries in an animal model of rotator cuff pathology. J Bone Joint Surg Am 2010;92:2270-8.  |
28. | Schmutz S, Fuchs T, Regenfelder F, Steinmann P, Zumstein M, Fuchs B. Expression of atrophy mRNA relates to tendon tear size in supraspinatus muscle. Clin Orthop Relat Res 2009;467:457-64.  |
29. | Gerber C, Schneeberger AG, Beck M, Schlegel U. Mechanical strength of repairs of the rotator cuff. J Bone Joint Surg Br 1994;76:371-80.  |
30. | Uhthoff HK, Seki M, Backman DS, Trudel G, Himori K, Sano H. Tensile strength of the supraspinatus after reimplantation into a bony trough: An experimental study in rabbits. J Shoulder Elbow Surg 2002;11:504-9.  |
31. | Hirose K, Kondo S, Choi HR, Mishima S, Iwata H, Ishiguro N. Spontaneous healing process of a supraspinatus tendon tear in rabbits. Arch Orthop Trauma Surg 2004;124:374-7.  |
32. | Gao J, Rasanen T, Persliden J, Messner K. The morphology of ligament insertions after failure at low strain velocity: an evaluation of ligament entheses in the rabbit knee. J Anat 1996;189 (Pt 1):127-33.  |
33. | Kobayashi M, Itoi E, Minagawa H, Miyakoshi N, Takahashi S, Tuoheti Y, et al. Expression of growth factors in the early phase of supraspinatus tendon healing in rabbits. J Shoulder Elbow Surg 2006;15:371.  |
34. | Hsu C, Chang J. Clinical implications of growth factors in flexor tendon wound healing. J Hand Surg Am 2004;29:551-63.  |
35. | Mazzocca AD, McCarthy MB, Chowaniec DM, Cote MP, Arciero RA, Drissi H. Rapid Isolation of Human Stem Cells (Connective Tissue Progenitor Cells) From the Proximal Humerus During Arthroscopic Rotator Cuff Surgery. Am J Sports Med 2010;38:1438-47.  |
36. | Uhthoff HK, Sano H, Trudel G, Ishii H. Early reactions after reimplantation of the tendon of supraspinatus into bone. A study in rabbits. J Bone Joint Surg Br 2000;82:1072-6.  |
37. | Soslowsky LJ, Thomopoulos S, Esmail A, Flanagan CL, Iannotti JP, Williamson JD 3 rd , et al. Rotator cuff tendinosis in an animal model: role of extrinsic and overuse factors. Ann Biomed Eng 2002;30:1057-63.  |
38. | Chuen FS, Chuk CY, Ping WY, Nar WW, Kim HL, Ming CK. Immunohistochemical characterization of cells in adult human patellar tendons. J Histochem Cytochem 2004;52:1151-7.  |
39. | Laurens N, Koolwijk P, de Maat MP. Fibrin structure and wound healing. J Thromb Haemost 2006;4:932-9.  |
40. | Ouyang HW, Goh JC, Lee EH. Viability of allogeneic bone marrow stromal cells following local delivery into patella tendon in rabbit model. Cell Transplant 2004;13:649-57.  |
41. | Gulotta LV, Kovacevic D, Packer JD, Deng XH, Rodeo SA. Bone marrow-derived mesenchymal stem cells transduced with scleraxis improve rotator cuff healing in a rat model. Am J Sports Med 2011;39:1282-9.  |
42. | Ulrich Meyer TM, Handschel J, Wiesmann HP. Fundamentals of tissue engineering and regenerative medicine. Kidlington, Oxford, UK: Springer-Verlag; 2009.  |
43. | Carpenter JE, Thomopoulos S, Flanagan CL, DeBano CM, Soslowsky LJ. Rotator cuff defect healing: a biomechanical and histologic analysis in an animal model. J Shoulder Elbow Surg 1998;7:599-605.  |
44. | Molloy T, Wang Y, Murrell G. The roles of growth factors in tendon and ligament healing. Sports Med 2003;33:381-94.  |
45. | Thomopoulos S, Williams GR, Gimbel JA, Favata M, Soslowsky LJ. Variation of biomechanical, structural, and compositional properties along the tendon to bone insertion site. J Orthop Res 2003;21:413-9.  |
46. | Butler DL, Juncosa-Melvin N, Boivin GP, Galloway MT, Shearn JT, Gooch C, et al. Functional tissue engineering for tendon repair: A multidisciplinary strategy using mesenchymal stem cells, bioscaffolds, and mechanical stimulation. J Orthop Res 2008;26:1-9.  |
47. | Liu SH, Panossian V, al-Shaikh R, Tomin E, Shepherd E, Finerman GA, et al. Morphology and matrix composition during early tendon to bone healing. Clin Orthop 1997;339:253-60.  |
48. | Thomopoulos S, Zampiakis E, Das R, Silva MJ, Gelberman RH. The effect of muscle loading on flexor tendon-to-bone healing in a canine model. J Orthop Res 2008;26:1611-7.  |
49. | Thomopoulos S, Williams GR, Soslowsky LJ. Tendon to bone healing: differences in biomechanical, structural, and compositional properties due to a range of activity levels. J Biomech Eng 2003;125:106-13.  |
50. | Shea KP, McCarthy MB, Ledgard F, Arciero C, Chowaniec D, Mazzocca AD. Human tendon cell response to 7 commercially available extracellular matrix materials: an in vitro study. Arthroscopy 2010;26:1181-8.  |
51. | Valentin JE, Badylak JS, McCabe GP, Badylak SF. Extracellular matrix bioscaffolds for orthopaedic applications. A comparative histologic study. J Bone Joint Surg Am 2006;88:2673-86.  |
52. | Derwin KA, Baker AR, Spragg RK, Leigh DR, Iannotti JP. Commercial extracellular matrix scaffolds for rotator cuff tendon repair. Biomechanical, biochemical, and cellular properties. J Bone Joint Surg Am 2006;88:2665-72.  |
53. | Choi HR, Kondo S, Hirose K, Ishiguro N, Hasegawa Y, Iwata H. Expression and enzymatic activity of MMP-2 during healing process of the acute supraspinatus tendon tear in rabbits. J Orthop Res 2002;20:927-33.  |
54. | Lo IK, Marchuk LL, Hollinshead R, Hart DA, Frank CB. Matrix metalloproteinase and tissue inhibitor of matrix metalloproteinase mRNA levels are specifically altered in torn rotator cuff tendons. Am J Sports Med 2004;32:1223-9.  |
55. | Yoshihara Y, Hamada K, Nakajima T, Fujikawa K, Fukuda H. Biochemical markers in the synovial fluid of glenohumeral joints from patients with rotator cuff tear. J Orthop Res 2001;19:573-9.  |
56. | Bedi A, Fox AJ, Kovacevic D, Deng XH, Warren RF, Rodeo SA. Doxycycline-mediated inhibition of matrix metalloproteinases improves healing after rotator cuff repair. Am J Sports Med 2010;38:308-17.  |
57. | Bedi A, Kovacevic D, Hettrich C, Gulotta LV, Ehteshami JR, Warren RF, et al. The effect of matrix metalloproteinase inhibition on tendon-to-bone healing in a rotator cuff repair model. J Shoulder Elbow Surg 2010;19:384-91.  |
58. | Holmbeck K, Bianco P, Chrysovergis K, Yamada S, Birkedal-Hansen H. MT1-MMP-dependent, apoptotic remodeling of unmineralized cartilage: a critical process in skeletal growth. J Cell Biol 2003;163:661-71.  |
59. | Sekiya I, Larson BL, Smith JR, Pochampally R, Cui JG, Prockop DJ. Expansion of human adult stem cells from bone marrow stroma: Conditions that maximize the yields of early progenitors and evaluate their quality. Stem Cells 2002;20:530-41.  |
60. | Sekiya I, Vuoristo JT, Larson BL, Prockop DJ. In vitro cartilage formation by human adult stem cells from bone marrow stroma defines the sequence of cellular and molecular events during chondrogenesis. Proc Natl Acad Sci U S A 2002;99:4397-402.  |
61. | Annabi B, Laflamme C, Sina A, Lachambre MP, Beliveau R. A MT1-MMP/NF-kappaB signaling axis as a checkpoint controller of COX-2 expression in CD133+ U87 glioblastoma cells. J Neuroinflammation 2009;6:8.  |
62. | Wurgler-Hauri CC, Dourte LM, Baradet TC, Williams GR, Soslowsky LJ. Temporal expression of 8 growth factors in tendon-to-bone healing in a rat supraspinatus model. J Shoulder Elbow Surg 2007;16(5, Supplement 1):S198-203.  |
63. | Takahasih S, Nakajima M, Kobayashi M, Wakabayashi I, Miyakoshi N, Minagawa H, et al. Effect of recombinant basic fibroblast growth factor (bFGF) on fibroblast-like cells from human rotator cuff tendon. Tohoku J Exp Med 2002;198:207-14.  |
64. | Ide J, Kikukawa K, Hirose J, Iyama K, Sakamoto H, Mizuta H. The effects of fibroblast growth factor-2 on rotator cuff reconstruction with acellular dermal matrix grafts. Arthroscopy 2009;25:608-16.  |
65. | Ide J, Kikukawa K, Hirose J, Iyama K, Sakamoto H, Fujimoto T, et al. The effect of a local application of fibroblast growth factor-2 on tendon-to-bone remodeling in rats with acute injury and repair of the supraspinatus tendon. J Shoulder Elbow Surg2009;18:391-8.  |
66. | Thomopoulos S, Kim HM, Das R, Silva MJ, Sakiyama-Elbert S, Amiel D, et al. The effects of exogenous basic fibroblast growth factor on intrasynovial flexor tendon healing in a canine model. J Bone Joint Surg Am 2010;92:2285-93.  |
67. | Dines JS, Grande DA, Dines DM. Tissue engineering and rotator cuff tendon healing. J Shoulder Elbow Surg Am 2007;16(5 Suppl):S204-7.  |
68. | Chan BP, Fu SC, Qin L, Rolf C, Chan KM. Supplementation-time dependence of growth factors in promoting tendon healing. Clin Orthop Relat Res 2006;448:240-7.  |
69. | Uggen JC, Dines J, Uggen CW, Mason JS, Razzano P, Dines D, et al. Tendon gene therapy modulates the local repair environment in the shoulder. J Am Osteopath Assoc 2005;105:20-1.  |
70. | Uggen C, Dines J, McGarry M, Grande D, Lee T, Limpisvasti O. The effect of recombinant human platelet-derived growth factor BB-coated sutures on rotator cuff healing in a sheep model. Arthroscopy 2010;26:1456-62.  |
71. | Yoshikawa T, Tohyama H, Katsura T, Kondo E, Kotani Y, Matsumoto H, et al. Effects of local administration of vascular endothelial growth factor on mechanical characteristics of the semitendinosus tendon graft after anterior cruciate ligament reconstruction in sheep. Am J Sports Med 2006;34:1918-25.  |
72. | Zhang F, Liu H, Stile F, Lei MP, Pang Y, Oswald TM, et al. Effect of vascular endothelial growth factor on rat Achilles tendon healing. Plast Reconstr Surg 2003;112:1613-9.  |
73. | Manning CN, Kim HM, Sakiyama-Elbert S, Galatz LM, Havlioglu N, Thomopoulos S. Sustained delivery of transforming growth factor beta three enhances tendon-to-bone healing in a rat model. J Orthop Res 2011;29:1099-105.  |
74. | Seeherman HJ, Archambault JM, Rodeo SA, Turner AS, Zekas L, D'Augusta D, et al. rhBMP-12 accelerates healing of rotator cuff repairs in a sheep model. J Bone Joint Surg Am 2008;90:2206-19.  |
75. | Gulotta LV, Kovacevic D, Packer JD, Ehteshami JR, Rodeo SA. Adenoviral-mediated gene transfer of human bone morphogenetic protein-13 does not improve rotator cuff healing in a rat model. Am J Sports Med 2011;39:180-7.  |
76. | Murray DH, Kubiak EN, Jazrawi LM, Araghi A, Kummer F, Loebenberg MI, et al. The effect of cartilage-derived morphogenetic protein 2 on initial healing of a rotator cuff defect in a rat model. J Shoulder Elbow Surg 2007;16:251-4.  |
77. | Pauly S, Klatte F, Strobel C, Schmidmaier G, Greiner S, Scheibel M, et al. BMP-2 and BMP-7 affect human rotator cuff tendon cells in vitro. J Shoulder Elbow Surg 2011 in press.  |
78. | Chen CH, Chang CH, Wang KC, Su CI, Liu HT, Yu CM, et al. Enhancement of rotator cuff tendon-bone healing with injectable periosteum progenitor cells-BMP-2 hydrogel in vivo. Knee Surg Sports Traumatol Arthrosc 2011;19:1597-607.  |
79. | Bensaid W, Triffitt JT, Blanchat C, Oudina K, Sedel L, Petite H. A biodegradable fibrin scaffold for mesenchymal stem cell transplantation. Biomaterials 2003;24:2497-502.  |
80. | Karaoglu S, B Fisher M, Woo SL, Fu YC, Liang R, Abramowitch SD. Use of a bioscaffold to improve healing of a patellar tendon defect after graft harvest for ACL reconstruction: A study in rabbits. J Orthop Res 2008;26:255-63.  |
81. | Rodeo S, Potter H, Kawamura S, Turner A, Kim H, Atkinson B. Biologic augmentation of rotator cuff tendon-healing with use of a mixture of osteoinductive growth factors. J Bone Joint Surg 2007;89:2485-97.  |
82. | Lopez-Vidriero E, Goulding KA, Simon DA, Sanchez M, Johnson DH. The use of platelet-rich plasma in arthroscopy and sports medicine: optimizing the healing environment. Arthroscopy 2010;26:269-78.  |
83. | Zumstein MA, Berger S, Schober M, Boileau P, Nyffeler RW, Horn M, et al. Leukocyte-and platelet-rich fibrin (L-PRF) for long-term delivery of growth factor in rotator cuff repair: Review, preliminary results and future directions. Curr Pharm Biotechnol 2011 in press  |
84. | Dohan Ehrenfest DM, Del Corso M, Diss A, Mouhyi J, Charrier JB. Three-dimensional architecture and cell composition of a Choukroun's platelet-rich fibrin clot and membrane. J Periodontol 2010;81:546-55.  |
85. | Dohan Ehrenfest DM, Rasmusson L, Albrektsson T. Classification of platelet concentrates: from pure platelet-rich plasma (P-PRP) to leucocyte- and platelet-rich fibrin (L-PRF). Trends Biotechnol 2009;27:158-67.  |
86. | Randelli P, Arrigoni P, Ragone V, Aliprandi A, Cabitza P. Platelet rich plasma in arthroscopic rotator cuff repair: a prospective RCT study, 2-year follow-up. J Shoulder Elbow Surg 2011;20:518-28.  |
87. | Zhang J, Wang JH. Platelet-rich plasma releasate promotes differentiation of tendon stem cells into active tenocytes. Am J Sports Med 2010;38:2477-86.  |
88. | Castricini R, Longo UG, De Benedetto M, Panfoli N, Pirani P, Zini R, et al. Platelet-rich plasma augmentation for arthroscopic rotator cuff repair: a randomized controlled trial. Am J Sports Med 2011;39:258-65.  |
89. | Maniscalco P, Gambera D, Lunati A, Vox G, Fossombroni V, Beretta R, et al. The "Cascade" membrane: a new PRP device for tendon ruptures. Description and case report on rotator cuff tendon. Acta Biomed 2008;79:223-6.  |
90. | Dohan DM, Choukroun J, Diss A, Dohan SL, Dohan AJ, Mouhyi J, et al. Platelet-rich fibrin (PRF): a second-generation platelet concentrate. Part I: technological concepts and evolution. Oral Surg Oral Med Oral Pathol Oral Radiol Endod 2006;101:e37-44.  |
91. | Zumstein MA, Rumian A, Lesbats V, Trojani C, Boileau P. A new technique of biologic augmentation in repair of chronic rotator cuff tears with autologous platelet rich fibrin (PRF): Vacularization Response and Tendon in a Prospective randomized trial. Paper presented at: New Orleans: American Academy of Orthopedic Surgeons; 2010.  |
[Figure 1], [Figure 2]
|