The Immunomodulatory Roles of Vitamin D: New Tricks for an Old Dog
The active metabolite of vitamin D, termed calcitriol or 1,25(OH)2D3, has long been known to participate in mineral homeostasis, bone and skin metabolisms, cell proliferation and cancer progression, and the endocrine system. In the past two decades, however, a growing body of evidence has highlighted the importance of this hormone in a variety of other biological functions, including its paramount role in the modulation of the immune system (1). The vitamin D receptor (VDR) is a member of the nuclear receptor superfamily and exerts its transcriptional regulatory function, when bound to vitamin D, by forming a heterodimer with the retinoid-X-receptor (RXR) and then binding to VDR responsive elements (VDRE). Several enzymes involved in activation, metabolism, and synthesis of calcitriol, including the 25-hydroxyvitamin-D3-1-alpha-hydroxylase (CYP27B1), are expressed in a variety of cells of the immune system (2) (Table 1). Mutations of either the VDR or various Vitamin D–activating enzymes have been described in some autoimmune conditions. Furthermore, calcitriol has proved beneficial as an immunoregulatory hormone for the treatment of several autoimmune disorders (AID), including rheumatoid arthritis (RA), diabetes, multiple sclerosis, inflammatory bowel disease (IBD), and systemic lupus erythematosus. Indeed, topical application of VDR agonists to skin lesions is a common therapeutic regimen in the treatment of mild psoriasis (3). Because vitamin D3 participates in immunoregulation, it has been hypothesized that VDR agonists might be useful in cancer treatment and organ transplantion, providing beneficial clinical outcomes.
Immunomodulatory Targets of Vitamin D3
Owing to the pleiotropic nature of calcitriol’s biological effects, the systemic use of VDR agonists for immunotherapeutic purposes has sometimes proved unsafe or of little effect. Identifying how to fine tune the regulation of VDR signaling will be critical to the therapeutic success of these agonists. This is especially true because of the nature of self-reactive T cells and antigen presenting cells (APCs); the triggering of autoimmune responses by uncontrolled administration of VDR agonists must be prevented.
Calcitriol exhibits inhibitory effects on macrophages, dendritic cells (DCs), and other APCs (Table 1) and it is because of its immunosuppressive actions that calcitriol is useful in AID (4). The primary role of professional APCs, particularly DCs, is the presentation of antigens to naïve T cells, which, via the T-cell receptor (TCR), recognize the antigen–major histocompatibility complex (MHC) aggregates exposed on the cell surface of APCs.
VDR agonists hinder T-helper effector function by supporting the generation of regulatory T cells (5). In addition, calcitriol affects all the three major signals provided by APCs, which are necessary for optimal generation of effector T cells, including Ag-presentation by MHC, signals by costimulatory molecules, and production of the proinflammatory cytokine IL-12 (5–7).
VDR is not present in naive T cells and only becomes expressed following antigen (Ag) priming, with the precise role of calcitriol in T cell differentiation yet to be fully elucidated. Following their first TCR activation, naïve T cells undergo differentiation programs specifically dictated by the cytokines milieu. Interleukin-12 (IL-12) and IL-4 support differentiation into either of the effector T-cell subtypes Th-1 or Th-2, respectively, whereas IL-6 and TGF-β induce the effector Th-17 subset; and TGF-β and IL-10 support the generation and function of regulatory T cells (Treg), which are hyporesponsive (i.e., they possess a diminished capacity toward causing autoimmune responses) than the effector T cell subsets (8). Indeed, skewing the immune system from a Th-1- or a Th-17-supportive to a Th2-supportive milieu could be beneficial in treating AID. It is noteworthy that exposure to calcitriol and VDR agonists inhibits interferon-γ (IFN-γ) production (a Th-1 response) in T-cell clones and in CD4+ cells (CD4 is a known cell-surface marker for a subset of T cells) purified from human peripheral blood mononuclear cells (PBMCs) (9, 10). In addition, more recent data show that calcitriol can inhibit IL-17 production in T cells without a direct effect on Th-17 commitment (11, 12). In vitro data on Th-2 cells are less clear (13, 14); however, VDR agonists can inhibit the production of Th-2-specific cytokines in vivo in asthma patients, by enhancing Treg-mediated IL-10 production (15, 16). Thus, the most relevant cellular effect of calcitriol on T cells is the inhibition of cytokine production [IFN-γ (Th-1), IL-4 (Th-2), and IL-17 (Th-17), respectively] during T-cell activation and differentiation (13). Indeed, the promoters of both IFN-γ and IL-4 genes may be targets VDR-RXR of heterodimers in mouse T cells (13, 17). However, calcitriol might additionally affect the expression of transcription factors that classically direct the differentiation of Th-1, Th-2, and Th-17 cells (respectively, T-bet, Gata-3 and c-maf, and Rorγt). Results indicate this is true at least in the case of Th-2 cells (18).
After the first burst of Ag-dependent proliferation, T cells lose proliferative potential and, concomitantly, the ability to secrete IL-2, an important growth factor for T cells. Calcitriol and its analogs inhibit proliferation and IL-2 production, not only in T cell clones and hybridomas (9, 19), but also in human PBMCs and in purified CD4+ and CD8+ T cells (20). However, the addition of IL-2 to calcitriol-treated cells can antagonize the anti-proliferative effect of VDR agonists (9, 21), with different outcomes on cytokine production, depending on the differentiation/polarization state of the T cells. Disparity in the amount of IL-2 added in Th-differentiation protocols might ultimately explain why calcitriol stimulation of purified CD4+ T cells has led to divergent results in separate Th-2 studies (13, 18). Thus, calcitriol susceptibility might differ depending on differentiation state, polarization, and, ultimately, on VDR expression in different T-cell subsets.
A recent publication by von Essen et al. (22) provides important insights into the cross-talk between Ag-mediated T-cell activation and calcitriol-dependent responses in naive vs Ag-primed T cells. The authors uncover a previously unknown direct and positive role of calcitriol in T-cell activity, with important mechanistic implications for the increased functional avidity observed in Ag-primed cells over naïve precursors. The results describe how VDR stimulation participates in T-cell metabolism and sustains Ag-primed T-cell proliferation. In addition, the authors demonstrate how VDR signaling, in conjunction with TCR signaling, is required to enhance TCR-driven responsiveness in human Ag-primed T cells but not naïve T cells. Despite having increased activation of Zap70 (a TCR-specific protein tyrosine kinase) and LAT (a transmembrane protein that acts as a scaffold for signaling proteins at the TCR) in response to TCR triggering, naïve T cells possess lower TCR-responsiveness than do Ag-primed T cells, which can recruit calcium and activate extracellular signal–regulated kinase (ERK) much more efficiently. The reason for this dichotomy is that Ag-primed T-cell signaling machinery is readily organized to integrate TCR signals, having greater amounts of phospholipase C–γ1 (PLC-γ1) when compared to naïve T cells. PLC-γ1 expression in naïve T cells was only detectable starting two days after the initial TCR stimulation (22). In line with previous findings (12), VDR was detectable only in Ag-primed but not in naïve T cells, and the increase in receptor expression preceded PLC-γ1 expression by several hours. Previously, it was found that VDRE are present in the PLC-γ1 gene promoter (23), suggesting that this gene is a direct target of VDR-RXR. Indeed, von Essen et al. (22) found that decreased VDR signaling—achieved using VDR antagonists— correlated with decreased PLC-γ1 expression, diminished T-cell metabolic activity, and a block in T-cell proliferation. PLC-γ1 induction and VDR expression were also inhibited by CYB27B1 antagonists, but such inhibition was reverted with the addition of calcitriol. Notably, naïve T cells could increase VDR expression independently of PLC-γ1, potentially via an alternative pathway involving the mitogen-activated protein kinase (MAPK) p38, which the authors show is required in Ag-primed T cells to maintain VDR expression.
Unfortunately, similar to other recent studies (24), von Essen et al. (22) fall short in dissecting potential differences of calcitriol-dependent events between CD4+ and CD8+ T cells. CD8+ T cells express the VDR and, upon activation, they also secrete IL-2 (albeit to lower extents than do CD4+ T cells), proliferate faster than CD4+ T cells, and differentiate into cytolytic T cells (CTL). Thus, the molecular mechanisms by which VDR-dependent and p38-dependent alternative pathways might differentially affect the proliferation and effector function of CD4+ vs CD8+ T cells remain still unclear.
It is somewhat difficult to reconcile this newly described stimulatory role of calcitriol on purified T cells (22) with the widely accepted paradigm of the inhibitory effects of calcitriol on T cells (11, 19–21). For instance, further studies are required to address whether VDR-dependent T-cell proliferation maintains the potential to differentiate into any of the Th-subsets or mainly differentiate toward a specific T-cell fate, for example, becoming immunoregulatory Treg cells. Importantly, calcitriol-mediated modulation of IL-2 secretion and its effects on the proliferation and differentiation programs achieved in Ag-primed T cells proliferating in response to administered VDR agonists are not tackled in the present study. As discussed above, although IL-2 remains a major target of VDR agonists, this proliferative cytokine can, to a certain extent, counteract calcitriol effects, hence exogenous provision of IL-2 might obstruct a clear understanding of VDR functions.
The involvement of p38 in VDR signaling remains of particular interest for several reasons. Firstly, von Essen et al. (22) seem to resolve the historical conundrum by which a non-responsive naïve T cell is able to develop a robust Ag-driven response and turn into a fully competent Ag-primed T cells with increased functional avidity. Furthermore, they reveal a hitherto unknown pro-inflammatory potential of VDR agonists in the proliferation of autoreactive T cells, which is opposite to the well-known inhibitory effects of VDR agonists on APCs in vivo. Finally, they shed new light on the significance of the alternative p38 pathway (Figure 1), opening the ground to novel therapeutic applications. Further investigation is required to dissect the role of VDR signals and the upstream p38 alternative pathway in proliferation and effector functionality of autoreactive CD4+ Th-subsets and CD8+ CTLs. Indeed, the specific inhibition of VDR signaling through the p38 alternative pathway might prove to have therapeutic value.
New roles for the alternative p38 pathway and vitamin D3 in T cells. In naïve T cells, TCR signaling, coupled with costimulation of CD28, results in the activation of the mitogen-activated protein kinase termed p38 either by upstream MKKs, in a LAT-dependent mechanism (classical pathway; in blue), or, alternatively, by the protein tyrosine kinase Zap70, which is, itself, activated by Lck (alternative pathway; in orange). von Essen et al. (22) have identified a previously unknown mechanistic role for the latter alternative p38 pathway, uncovering a novel homeostatic, and potentially pro-inflammatory, role for vitamin D3 in T cells. Following Ag-driven TCR signaling, alternative p38 activation induces the expression of VDR. Consequently, T cells become susceptible to calcitriol, which is responsible for promoting PLC-γ expression, which, in turn, enhances the response to calcium, therefore contributing to the transition from a naïve to a fully competent Ag-primed T cell with increased functional avidity for antigen. Additional VDR-responsive genes in T cells are IL-2 and the Th-defining cytokines IFN-γ, IL-4, and IL-17.
Upon TCR triggering and in the presence of CD28 costimulatory signaling, p38 is canonically phosphorylated by the upstream MAPK kinases (MKKs) MKK3 and MKK6, which phosphorylate the different isoforms of p38 (α and β) at both Thr180 and Thr182 via the classic LAT pathway (Figure 1). However, recent studies have identified an alternative pathway which, in the absence of costimulatory molecules, leads to an activation of p38 that is independent of MKK3 and MKK6 (25, 26). This alternative pathway relies on the activity of the protein tyrosine kinase Lck and is initiated upon phosphorylation of p38α/β by the proximal kinase Zap70 that triggers autophosphorylation of Thr180. Because of its involvement in the regulation of several pro-inflammatory molecules (27)—including cytokines, iNOS, and COX2—p38 has been long identified as a suitable target in the treatment of cancer and AID, including RA, chronic obstructive pulmonary disease (COPD), Crohn’s disease, and, recently, however, clinical studies with p38 inhibitors have often proven unsuccessful either because of their toxicity in vivo or absence of clinical outcomes despite encouraging in vivo preclinical studies (28). It is now clear that the wide range of effects that VDR agonists have on the individual immune cell–types might cause contrasting outcomes in disease or health. The chances for successful intervention with VDR agonists or antagonists are crucially affected by the distribution of the different cell-types which may vary in location and number in different immunopathological conditions. In addition, unidentified VDR ligands, different from calcitriol and other known agonists, might exist in vivo (1); these might mediate and maintain a basal, homeostatic anti-inflammatory state, possibly via the p38 alternative pathway, in VDR-expressing cell-types. Thus, the pharmacological inhibition of p38, although beneficial in hampering inflammatory processes regulated via the classical pathway in many immune cell–types, may be detrimental to other homeostatic balances and might have implications for tumorigenesis. The identification and use of specific inhibitors that would target only the classical p38 pathway would, therefore, have a potential positive effect. A possible alternative to inhibiting specific p38 isoforms could be the modulation of other proteins involved in the alternative p38-dependent pathway, such as Zap70 and the adaptors of the Gadd45 protein family (Figure 1).
The two SH2 domains of Zap70 would be the ideal targets for therapeutic intervention because they control Zap70 localization and its substrate specificity. Indeed, structure-based design of Zap70 inhibitors has proved successful in initial drug-discovery studies. Small-molecule inhibitors of Gadd45 proteins could also represent an appealing solution. Gadd45 proteins are involved in multiple stress signaling responses, and altered activity of Gadd45 has been linked to hematopoietic malignancies and solid tumors (29). Changes in Gadd45 expression may also alter Th-1 type responses (30, 31). Induced expression of Gadd45β inhibits MKK4/7–dependent activation of Jun N-terminal kinase (JNK), an important pro-inflammatory and pro-apoptotic stress kinase. On the other hand, Gadd45α induces the classical LAT-p38 pathway (30) and has a positive effect on JNK (30), while inhibiting the alternative ZAP70-p38 pathway (25). Consequently, inhibition of Gadd45α should prove beneficial in reducing the pro-inflammatory outcomes of the classical p38/JNK pathway in both T cells and APCs by affecting IL-12 production and Th1 responses (32), despite a positive effect on the alternative p38 pathway to VDR activation in T cells.
Another aspect of VDR signaling with potential for therapeutic intervention is the epigenetic regulation of VDR target genes and of VDR itself. Interestingly, some VDR-associated co-activators have intrinsic histone acetyl transferase (HAT) activity (33). Furthermore, inhibition of histone deacetylases (HDACs) is important in restoring VDR transcriptional responses by recovering expression of anti-proliferative genes, including Gadd45α. HDAC inhibitors (HDACi) restore the VDR ligand–mediated switch from co-repressor to co-activator, which plays a central role in VDR signaling (and that of other nuclear receptors); VDR signaling is disregulated in some forms of cancer that are insensitive to the anti-proliferative effects of VDR (34). Moreover, a novel targeting approach incorporating HDACi activity into the structure of a VDR agonist showed encouraging results, suppressing cell proliferation in cancer models in vitro (35). However, a more detailed understanding of the epigenetic modifications occurring on histones at the regulatory site of VDR-dependent genes is necessary to exploit the therapeutic potential of targeting HDACs and other epigenetic enzymes, in association with VDR agonists, particularly downstream of the alternative p38 pathway that provides distinct targets for intervention in T cells but not APCs.
In conclusion, von Essen and colleagues (22) shift back the focus on the importance of vitamin D in the modulation of the immune system. In particular, they raise the intriguing possibility that there may exist opposed (and some as yet undefined) effects of natural and synthetic VDR agonists on a wide variety of immune responses in cells expressing VDR and enzymes involved in vitamin D metabolism. In doing so, they underscore the call for more detailed studies in order to identify specific and effective therapeutic tools for targeting VDR and small molecules that modulate VDR signal transduction.
- Copyright © 2010
References
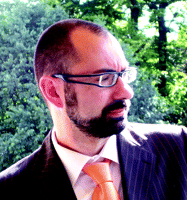
Valentino Parravicini, PhD, is currently Principal Scientist-Immunologist in the EpiNova DPU, immune inflammation CEDD, GlaxoSmithKline (GSK) in Stevenage, UK. After his degree at the Universita’ degli Studi di Milano, he joined Juan Rivera at the National Institute of Arthritis and Musculoskeletal and Skin Diseases (NIAMS) at the NIH, Bethesda. There he contributed to the identification of a novel alternative pathway downstream the high affinity receptor for IgE in mast cells. He then worked with Rose Zamoyska at the NIMR-MRC, London, UK, studying the modulatory role of Itch in B, αβ-, and γ-T cells. His current research interests focus on the epigenetics of immune responses. E-mail valentino.x.parravicini{at}gsk.com
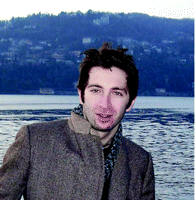
Stefano Caserta, PhD, is a Cancer Research UK Postdoctoral Fellow and works on TCR signaling in memory T cells with Rose Zamoyska (Institute of Infection and Immunology Research, University of Edinburgh, UK). His doctorate in Basic and Applied Immunology (Vita-Salute San Raffaele University, Italy) focused on cytokines and memory T cells in anti-cancer therapy. He previously joined Anna Mondino’s Lab (San Raffaele Scientific Institute, Italy) to study anti-tumor endogenous T-cell responses and to develop artificial antigen presenting cells for cancer immunotherapy. His research focuses on generation and maintenance of memory T cells in conditions of chronic antigen exposure. E-mail stefano.caserta{at}ed.ac.uk