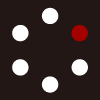
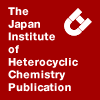
HETEROCYCLES
An International Journal for Reviews and Communications in Heterocyclic ChemistryWeb Edition ISSN: 1881-0942
Published online by The Japan Institute of Heterocyclic Chemistry
e-Journal
Full Text HTML
Received, 14th November, 2008, Accepted, 18th December, 2008, Published online, 22nd December, 2008.
DOI: 10.3987/COM-08-11606
■ A New and Facile Synthesis of Rutaecarpine Alkaloids
Chih-Shone Lee,* Cheng-Kuo Liu, Yen-Yao Cheng, and Che-Ming Teng
Department of Chemistry, National Sun Yat Sen University, Kaohsiung, 80424, Taiwan, R.O.C.
Abstract
Relevant rutaecarpine analogues (1a-d) have been synthesized efficiently from the ring opened β-carboline derivatives (3a-d) as key intermediates. A unique one-pot reductive-cyclization as key reaction furnished the synthesis of rutaecarpine alkaloids in excellent yields. The key intermediates (3a-d) were prepared from tryptamine following acylation, Bischler-Napieralski cyclization, benzoylation, and oxidative cleavage of the exocyclic double bond. This new synthetic approach provides a facile access to rutaecarpine analogues with potent inhibitory effect on platelet aggregation.Large numbers of quinazolinocarboline alkaloids including rutaecarpine (1a) and its analogues,1 euxylophoricine A (1b) and euxylophoricine C (1c), isolated from the dried fruits of Evodia rutaecarpa and callus tissue cultured from the stem of Phellodendron amurense, are characterized by their potent biological activities.2-5 For instance, they are used in Chinese herbal medicine under the name Wu-Chu-Yu for remedy such as headache, dysentery, cholera, worm infections and postpartum.6 Rutaecarpine and its analogues were also found to possess anti-stomachic, anti-emetic, anti-nociceptive, anti-inflammatory, anti-pyretic, analgesic, astringent, anti-hypertensive, uterotonic, cycloxygenase (COX-2) inhibitory and agonist TCDD-receptor activities.7 Furthermore, rutaecarpine can also suppress platelet plug formation in mesenteric venules and increase intracellular Ca2+ in endothelial cells.8 Recently, Don et al. reported that rutaecarpine derivatives selectively inhibited human CYP1A1, CYP1A2 and CYP2B1.9 In view of the interesting biological properties exhibited by these rutaecarpine alkaloids, there have been interests in the development of new synthetic method for quinazolinocarboline alkaloids. Robinson and coworkers reported the first total synthesis of rutaecarpine,10 and since then versatile routes to its derivatives have been achieved.11 For example, Eguchi et al. applied aza-Wittig reaction for various construction of quinazolinone alkaloids including a short and facile synthesis of rutaecarpine.11i In our previous study, we developed a new approach toward the synthesis of quinazolinocarboline analogues using one-pot reductive–cyclization as key step.12 Herein we report our recent studies on the total syntheses of rutaecarpine alkaloids (Figure 1).
The general approach towards the synthesis of rutaecarpine (1a) and various analogues involved the late construction of the indole skeleton (A, B rings) by the widely-used Fischer indole synthesis through an acid-catalyzed or thermal sigmatropic rearrangement of an N-aryl hydrazone. On the other hand, the quinazolinocarboline backbone (Figure 2) has been reported to give the ring opened β-carboline derivative upon addition of base.13 Our strategy is to synthesize a key intermediate similar to the carboline derivative, and develop a concise and new synthesis of 1a-d complementary to efforts on synthesis of rutaecarpine alkaloids described to date.
The retrosynthetic analysis is shown in Scheme 1. We envisage that the reverse cyclization to rutaecarpine analogues (1a-d) from the nitro-intermediate of the ring opened β-carboline derivatives (3a-d) can be carried out in a one-pot reaction via reductive-cyclization. Furthermore, the 2,3,4,9- tetrahydro-β-carbolin-1-one (3a-d) can be readily prepared from 1-methyl-4,9-dihydro-3H-β-carboline (5) in two steps that involved the isomerization of the endocyclic double bond to the exocyclic double bond,14 followed by an oxidative cleavage of the double bond to give the requisite carbonyl group.15 In addition, it is also of interest to investigate the biological activities of these ring opened β-carboline derivatives synthesized. To our knowledge, no approach involving the construction of a β-carboline rings first, followed by the construction of the quinazolinone ring has been reported.
1-Methyl-4,9-dihydro-3H-β-carboline (5) can be prepared from commercially available tryptamine. Treatment of tryptamine with acetic anhydride gave the N-acetylated adduct, and subsequent Bischler-Napieralski cyclization in POCl3 gave β-carboline (5) via general procedure in good yield.16 The reaction of β-carboline (5) with various benzoly chloride derivatives (6a-d), prepared from the corresponding known benzoic acid derivatives with SOCl2, afforded the requisite intermediates 4a-d for the synthesis of rutaecarpine alkaloids (Scheme 2). The unstable intermediates 4a-d were not subjected to chromatographic purification. We then first attempted to oxidatively cleave the exocyclic double bond of crude 4a-d under ozonolysis condition, but was unsuccessful presumably due to the reactivity of the indole 2,3-double bond toward ozone to give 2-oxoindole compound via pinacol rearrangement (Scheme 3).17 Johnson-Lemieux oxidative cleavage to the double bond of 4a, employing sodium periodate as an oxidation reagent and osmium tetroxide as a catalyst, gave the corresponding 3a in only 15 % yield.18 Alternatively, the use of potassium permanganate in cold acetone for the oxidative cleavage of the exocyclic double bond of 4a-d successfully gave the desired 2,3,4,9-tetrahydro- β-carbolin-1-one derivatives (3a-d) in moderate yields.
The ring open β-carboline intermediates (3a-d) can be easily prepared in facile steps from tryptamine. A one-pot reaction was sorted for the formation of the quinazolinone ring in the reaction sequence to synthesize rutaecarpine analogues (Scheme 4). This involved the reduction of the nitro- to the amino- group, followed by an in situ condensation of the free amino group with the ring amide to form the quinazolinone ring.
It was found that treatment of 3a-d with tin powder in HCl/MeOH followed by basic work-up can afford rutaecarpine alkaloids (1a-d) in a one-pot reaction with 86 to 94 % yield. In an independent study on the search of antiplatelet aggregatory agent, we found that 3-chlororutaecarpine (1d) exhibited extraordinarily inhibitory effect on platelet aggregation induced by arachidonic acid (Table 1).
In conclusion, a concise and facile procedure for the synthesis of rutaecarpine analogues (1a-d) by applying one-pot reductive-cyclization reaction as key step has been developed. This newstrategy for the general construction of rutaecarpine alkaloids with potent inhibitory effect on platelet aggregation is noteworthy.
EXPERIMENTAL
All melting points were taken with a Thomas-Hoover capillary melting point apparatus and are uncorrected. 1H nuclear magnetic resonance spectra were recorded on Varian Associates VXR-300 (300 MHz) spectrometer and are reported in parts per million from internal tetramethylsilane on the scale. Assignments are based on multiplicity, coupling constant and chemical shift data and in certain instances may be regarded as tentative. 13C nuclear magnetic resonance spectra were rcorded on a VXR-300. 13C-NMR spectra were recorded as Broad-Band, Off-Resonance-Decoupled or DEPT (Distortionless Enhancement by Polarization Transfer) spectra. Infrared spectra were taken with a Bio-Rad FTS-40 instrument. Low resolution mass spectra were recorded on Hitachi M-52 instrument at an ionization energy of 70 ev. Samples on which exact masses were measured exhibited no significant peaks at m/e values greater than those of the parent. Solvents and reagents were dried and purified prior to use when deemed necessary. High resolution mass spectra were recorded on Jeol JMS-HX110 instrument. All reaction temperatures refer to that of the reaction mixture unless indicated otherwise. Column chromatography was performed over EM laboratories silica 60 (70-230 mesh). Ethyl acetate and n-hexane, used as eluents in column chromatography, were distilled prior to use. The order of experimental procedures generally follows their order of appearance in the text.
General procedure for preparation of 3a-d
To a stirred solution of 2-nitrobenzoic acid (1.085 g, 6.50 mmol) in CH2Cl2 (47 mL) stirred at rt was slowly added SOCl2 (5.57 mL). The reaction mixture was refluxed for 1h and concentrated in vacuo to obtain the acid chloride (6a) as a crude residue. The residue was dissolved in 40 mL of CH2Cl2 and slowly added to a solution of compound 5 (1000 mg, 5.95 mmol) in 57 mL of CH2Cl2. The reaction mixture was stirred at rt for 2 h and concentrated in vacuo to afford the crude residue. The residue was worked-up in brine and extracted with CHCl3. The organic layer was dried and concentrated in vacuo to give the crude product 4a ready for the following oxidative cleavage without further purification. The crude product 4a was dissolved in cold acetone, slowly added KMnO4 and the reaction mixture was monitored by TLC. After the requisite reaction was done, the reaction mixture was concentrated in vacuo, the residue was worked-up with aqueous ammonium chloride solution and extracted with CHCl3. The organic layer was washed with brine, dried and concentrated in vacuo to give the crude product, which was subjected to column chromatography to obtain the pure product 3a (996 mg, 2.97 mmol) with a total yield of 50 %.
N-(2-Nitrobenzoyl)-1-oxo-1,2,3,4-tetrahydro-β-carboline (3a): mp 109-111 °C (MeOH/CH2Cl2); 1H NMR (300 MHz, CDCl3) δ 3.21 (t, J = 6.0 Hz, 2H), 4.56 (br, 2H), 7.16-7.21 (m, 1H), 7.25-7.27 (m, 1H), 7.33-7.39 (m, 2H), 7.49-7.55 (m, 1H), 7.71-7.83 (m, 2H), 8.19 (dd, J = 1.2, 8.4 Hz, 1H), 8.76 (brs, 1H, NH); 13C NMR (75 MHz, CDCl3) δ 20.51, 44.01, 112.58, 120.93, 120.98, 123.99, 124.67 (2C), 125.16, 126.83, 126.88, 129.12, 134.09, 135.29, 138.62, 144.60, 160.03, 168.22; IR (CHCl3, cm-1) 3457 (NH), 1684 (C=O), 1680 (C=O), 1530 (NO2), 1347 (NO2); MS m/z (%) 335 (M+, 35.6%), 289 (M+-46, 91.6%), 185 (M+-150, 51.9%), 184 (M+-151, 45.8%), 56 (M+-179, 67.3%), 150 (M+-85, 50.4%), 129 (M+-206, 100%), 128 (M+-207, 85.5%); HRMS m/z: Calcd for C18H13N3O4: 335.0890. Found: 335.0893. Anal. Calcd for C18H13N3O4: C, 64.47; H, 3.91; N, 12.53; Found: C, 64.52; H, 3.93; N, 12.45.
N-(4,5-Dimethoxy-2-nitrobenzoyl)-1-oxo-1,2,3,4-tetrahydro- β-carboline (3b): mp 117-119 °C (MeOH/CH2Cl2); 1H NMR (300 MHz, CDCl3) δ3.22 (t, J = 6.3 Hz, 2H), 3.94 (s, 3H), 3.95 (s, 3H), 4.57 (t, J = 6.3Hz, 2H), 6.87 (s, 1H), 7.18 (t, J = 6.9Hz, 1H), 7.27 (d, J = 8.4Hz, 1H), 7.36 (t, J = 6.9Hz, 1H), 7.64 (d. J = 8.1Hz, 1H), 7.68 (s, 1H), 8.81 (brs, 1H, NH); 13C NMR (75 MHz, CDCl3) δ20.53, 44.11, 56.36, 56.54, 106.73, 108.55, 112.49, 121.00, 121.04, 124.70, 124.76, 125.32, 126.95, 129.65, 137.60, 138.58, 148.74, 154.02, 160.05, 168.10; IR (CHCl3, cm-1) 3456 (NH), 1704 (C=O), 1684 (C=O), 1518 (NO2), 1333 (NO2); MS m/z (%) 395 (M+, 0.1%), 350 (M+-45, 2.37%), 349 (M+-46, 100%), 136 (M+-259, 39.1%), 129 (M+-266, 32.6%), 128 (M+-267, 26.7%), 93 (M+-302, 25.3%); HRMS m/z: Calcd for C20H17N3O6: 395.1126. Found: 395.1124. Anal. Calcd for C20H17N3O6: C, 60.76; H, 4.33; N, 10.63. Found: C, 60.81; H, 4.37; N, 10.58.
N-(4,5-Dimethylenedioxy-2-nitrobenzoyl)-1-oxo-1,2,3,4-tetrahydro- β-carboline (3c): mp 111-113 °C (MeOH/CH2Cl2); 1H NMR (300 MHz, CDCl3) δ 3.22 (t, J = 6.3 Hz, 2H), 4.57 (t, J = 6.3 Hz, 2H), 6.14 (s, 2H), 6.75 (s, 1H), 7.19-7.21 (m, 1H), 7.30-7.40 (m, 2H), 7.64 (s, 1H), 7.65 (d, J = 3.6 Hz, 1H), 8.68 (brs, 1H, NH); 13C NMR (75 MHz, CDCl3) δ20.57, 44.10, 103.46, 104.81, 106.16, 112.46, 121.12, 121.17, 124.73, 124.86, 125.31, 127.09, 131.98, 138.52, 139.26, 148.11, 152.71, 159.98, 167.62; IR (CHCl3, cm-1) 3464 (NH), 1674 (C=O), 1669 (C=O), 1552 (NO2), 1340 (NO2); MS m/z (%) 379 (M+, 80.4%), 129 (M+-250, 63.59%), 120 (M+-259, 71%), 55 (M+-324, 98.9%), 44 (M+-335, 100%); HRMS m/z: Calcd for C19H13N3O6: 379.0784. Found: 379.0788. Anal. Calcd for C19H13N3O6: C, 60.16; H, 3.45; N, 11.08. Found: C, 60.20; H, 3.50; N, 11.02.
N-(5-Chloro-2-nitrobenzoyl)-1-oxo-1,2,3,4-tetrahydro- β-carboline (3d): mp 108-110 °C (MeOH/CH2Cl2); 1H NMR (300 MHz, CDCl3) δ 3.24 (t, J = 6.0 Hz, 2H), 4.57 (t, J = 6.0 Hz, 2H), 7.20-7.23 (m, 1H), 7.32-7.39 (m, 3H), 7.65-7.68 (m, 2H), 8.21 (s, 1H), 8.62 (brs, 1H, NH); 13C NMR (75 MHz, CDCl3) δ20.58, 44.07, 112.48, 121.17, 124.32, 124.79, 124.92, 125.04, 127.25, 128.03, 133.66, 134.16, 135.20, 138.58(2C), 145.25, 159.93, 167.26; IR (CHCl3, cm-1) 3461 (NH), 1695 (C=O), 1686 (C=O), 1530 (NO2), 1347 (NO2); MS m/z (%) 369 (M+, 18%), 307 (M+-62, 24.6%), 154 (M+-215, 100%), 136 (M+-233, 96.0%), 89 (M+-280, 65.6%), 77 (M+-292, 66.7%); HRMS m/z: Calcd for C18H12ClN3O4: 369.0502. Found: 369.0515. Anal. Calcd for C18H12ClN3O4: C, 58.47; H, 3.27; N, 11.36. Found: C, 58.55; H, 3.30; N, 11.29.
General procedure for preparation of 1a-d
To a stirred solution of 3a (87 mg, 0.26 mmol) in 16 mL of MeOH cooled with ice-bath was slowly added tin powder (90.6 mg, 0.78 mmol). The reaction mixture was slowly added 2 mL of concentrated HCl in ice-bath. The solution was then stirred at rt for extra 12 h followed by basic work-up with NaOH solution, and then was added small amount of CHCl3 and triethyl amine to stir another 3 h. The reaction mixture was added brine and extracted with CHCl3. The organic layer was washed with brine, dried and concentrated in vacuo to afford the crude product 1a, which was subjected to chromatograhpy to obtain the pure 1a (69.9 mg, 0.24 mmol) with a total yield of 94 %.
Rutaecarpine (1a): mp 254-255 °C (lit.,11q mp 257-259 °C); 1H NMR (300 MHz, CDCl3) δ3.24 (t, J = 6.9 Hz, 2H), 4.59 (t, J = 6.9 Hz, 2H), 7.19 (t, J = 8.4 Hz, 1H), 7.34 (dt, J = 1.2, 6.8 Hz, 1H), 7.41-7.67 (m, 2H), 7.63-7.73 (m, 3H), 8.32 (dd, J = 1.2, 7.8 Hz, 1H), 9.27 (brs, 1H,NH); 13C NMR (75 MHz, CDCl3) δ19.68, 41.12, 112.07, 118.33, 120.08, 120.62, 121.37, 125.58, 125.64, 126.19, 126.61, 127.23, 134.32(2C), 138.27, 144.95, 147.52, 161.59; IR (CHCl3, cm-1) 3463 (NH), 1676 (C=O); MS m/z (%) 287 (M+, 79.2%), 286 (M+-1, 100%); HRMS m/z: Calcd for C18H13N3O: 287.1054. Found: 287.1055.
Euxylophoricine A (1b): mp 293-295 °C (lit.,13 mp 294-295 °C); 1H NMR (300 MHz, CDCl3) δ3.23 (t, J = 7.0 Hz, 2H), 3.98 (s, 3H), 4.01(s, 3H), 4.59 (t, J = 7.0 Hz, 2H), 7.06 (s, 1H), 7.19 (t, J = 7.8Hz, 1H), 7.33 (dd, J = 7.8, 8.1 Hz, 1H), 7.42 (d, J = 8.1 Hz, 1H), 7.63 (d, J = 8.1 Hz, 1H), 7.66 (s, 1H), 9.22 (brs, 1H, NH); 13C NMR (75 MHz, CDCl3) δ19.69, 41.10, 56.22, 56.35, 106.41, 107.12, 111.97, 114.50, 117.64, 119.99, 120.62, 125.36, 125.73, 127.35, 138.13, 143.68, 143.99, 148.76, 154.98, 160.90; IR (CHCl3, cm-1) 3461 (NH), 1663 (C=O); MS m/z (%) 347 (M+, 100%), 332 (M+-25, 22.7%), 128 (M+-219, 13.4%), 55 (M+-292, 48.6%), 43 (M+-304, 74.6%); HRMS m/z: Calcd for C20H17N3O3: 347.1264. Found: 347.1265.
Euxylophoricine C (1c): mp 307-308 °C (lit.,13 mp 310-312 °C); 1H NMR (300 MHz, CDCl3) δ3.27 (t, J = 6.9 Hz, 2H), 4.57 (t, J = 6.9 Hz, 2H), 6.10 (s, 2H), 7.03 (s, 1H), 7.18 (t, J = 6.9 Hz, 1H), 7.36 (t, J = 6.9 Hz, 1H), 7.44 (d, J = 6.9 Hz, 1H), 7.63 (d, J = 6.9 Hz, 1H), 7.64 (s, 1H), 9.07 (brs, 1H, NH); 13C NMR (75 MHz, CDCl3) δ19.88, 41.89, 102.53, 104.29, 105.96, 111.97, 116.10, 117.64, 120.00, 120.60, 125.38, 125.70, 127.24, 138.11, 143.81, 143.58, 147.10, 153.42, 160.87; IR (CHCl3, cm-1) 3469 (NH), 1663 (C=O); MS m/z (%) 331 (M+, 100%), 330 (M+-1, 94%); HRMS m/z: Calcd for C19H13N3O3: 331.0957. Found: 331.0957.
3-Chlororutaecarpine (1d): mp 290-291 °C (lit.,13 mp 290-293 °C); 1H NMR (300 MHz, DMSO-d6) δ3.17 (t, J = 6.9 Hz, 2H), 4.43 (t, J = 6.9 Hz, 2H), 7.09 (t, J = 7.2-7.5 Hz, 1H), 7.28 (t, J = 7.5-7.8 Hz, 1H), 7.46-7.50 (m, 2H), 7.62-7.66 (m, 2H), 8.13 (d, J = 8.4 Hz, 1H), 11.86 (brs, 1H,NH); 13C NMR (75 MHz, DMSO-d6) δ19.05, 41.09, 112.82, 118.79, 119.68, 120.02, 120.27, 124.99, 125.22, 125.51, 126.25, 126.93, 128.86, 139.01, 139.09, 146.73, 148.73, 160.26; IR (CHCl3, cm-1) 3451 (NH), 1675 (C=O); MS m/z (%) 323 (M++2, 27.0%), 322 (M++1, 42.4%), 321 (M+, 80.8%), 320 (M+-1, 100%), 128 (M+-195, 19.2%); HRMS m/z: Calcd for C18H12ClN3O: 321.0632. Found: 321.0643.
ACKNOWLEDGMENT
We thank National Science Council, R.O.C. for financial support (NSC82-0208-M110-066).
References
1. Y. Asahina, Acta Phytochim., 1922, 1, 67.
2. T. Kamikado, S. Murakoshi, and S. Tamura, Agric. Biol. Chem., 1978, 42, 1515.
3. J. Bergman, The Alkaloids, Academic Press, New York, 1983, Vol. 21, pp. 29-54.
4. (a) A. Ikuta, H. Urabe, and T. Nakamura, J. Nat. Prod., 1998, 61, 1012; CrossRef (b) A. Ikuta, T. Nakamura, and H. Urabe, Phytochemistry, 1998, 48, 285. CrossRef
5. J. P. Michael, Nat. Prod. Rep., 1999, 16, 697. CrossRef
6. A. L. Chen and K. K. Chen, J. Am. Pharm. Assoc., 1933, 22, 716.
7. (a) C. L. King, Y. C. Kong, N. S. Wong, H. W. Yeung, H. H. S. Fong, and U. Sankawa, J. Nat. Prod., 1980, 43, 577; CrossRef (b) M. Gillner, J. Bergman, C. Cambillau, and J. A. Gustafsson, Carcinogenesis, 1989, 10, 651; CrossRef (c) U. Rannug, M. Sjögren, A. Rannug, M. Gillner, R. Toftgard, J. A. Gustafsson, H. Rosenkranz, and G. Klopman, Carcinogenesis, 1991, 12, 2007; CrossRef (d) H. Matsuda, M. Yoshikawa, S. Ko, M. Iinuma, and M. Kubo, Nat. Med., 1998, 52, 203.
8. (a) G. J. Wang, J. Shan, P. K. T. Pang, M. C. M. Yang, C. J. Chou, and C. F. Chen, J. Pharmacol. Exp. Therap., 1996, 270, 1016; (b) J. R. Sheu, W. C. Hung, C. H. Wu, Y. M. Lee, and M. H. Yen, Br. J. Haematol., 2000, 110, 110. CrossRef
9. M. J. Don, D. F. V. Lewis, S. Y. Wang, M. W. Tsai, and Y. F. Ueng, Bioorg. Med. Chem. Lett., 2003, 13, 2535. CrossRef
10. Y. Asahina, R. H. F. Manske, and R. Robinson, J. Chem. Soc., 1927, 1708.
11. (a) T. Kametani, T. Higa, C. V. Loc, M. Ihara, M. Koizumi, and K. Fukumoto, J. Am. Chem. Soc., 1976, 98, 6186; CrossRef (b) T. Kametani, C. V. Loc, T. Higa, M. Koizumi, M. Ihara, and K. Fukumoto, J. Am. Chem. Soc., 1977, 99, 2306; CrossRef (c) B. Danieli and G. Palmisano, Heterocycles, 1978, 9, 803; CrossRef (d) J. Kökösi, I. Hermecz, G. Szász, and Z. Mészáros, Tetrahedron Lett., 1981, 22, 4861; CrossRef (e) C. Kaneko, T. Chiba, K. Kasai, and C. Miwa, Heterocycles, 1985, 23, 1385; CrossRef (f) J. Kökösi, I. Hermecz, B. Podanyi, G. Szász, and Z. Mészáros, J. Heterocycl. Chem., 1985, 22, 1373; CrossRef (g) M. Mori, H. Kobayashi, M. Kimura, and Y. Ban, Heterocycles, 1985, 23, 2803; CrossRef (h) I. Hermecz, J. Kökösi, A. Horvath, D. I. Vasavari, B. Podanyi, G. Szász, and Z. Mészáros, J. Heterocycl. Chem., 1987, 24, 1045; CrossRef (i) S. Eguchi, H. Takeuchi and Y. Matsushita, Heterocycles, 1992, 33, 153, and references cited therein; CrossRef (j) I. Hermecz, J. Kökösi, D. I. Vasavari, B. Podanyi, and G. Szász, Heterocycles, 1994, 37, 903; CrossRef (k) L. M. Yang, C. F. Chen, and K. H. Lee, Bioorg. Med. Chem. Lett., 1995, 5, 465; CrossRef (l) S. H. Lee, S. I. Kim, J. G. Park, E. S. Lee, and Y. Jahng, Hetereocycles, 2001, 55, 1555; CrossRef (m) P. K. Mohanta and K. Kim, Tetrahedron Lett., 2002, 43, 3993; CrossRef (n) A. Witt and J. Bergman, Curr. Org. Chem., 2003, 7, 659; CrossRef (o) E. S. Lee, J. G. Park, and Y. Jahng, Tetrahedron Lett., 2003, 44, 1883, and refs. cited therein; CrossRef (p) S. P. Chavan and R. Sivappa, Tetrahedron Lett., 2004, 45, 997; CrossRef (q) S. B. Mhaske and P. A. Narshinha, Tetrahedron, 2004, 60, 3417; CrossRef (r) T. Harayama, A. Hori, G. Serban, Y. Morikami, T. Matsumoto, H. Abe, and Y. Takeuchi, Tetrahedron, 2004, 60, 10645; CrossRef (s) D. J. Connolly, D. Cusack, T. P. O'Sullivan and P. J. Guiry, Tetrahedron, 2005, 61, 10153; CrossRef (t) J. F. Liu, J. Lee, A. M. Dalton, G. Bi, L. Yu, C. M. Baldino, E. McElory, and M. Brown, Tetrahedron Lett., 2005, 46, 1241; CrossRef (u) M. K. Jeon, D. S. Kim, H. J. La, D. C. Ha, and Y. D. Gong, Tetrahedron Lett., 2005, 46, 7477; CrossRef (v) A. Hamid, A. Elomri, and A. Daïch, Tetrahedron Lett., 2006, 47, 1777; CrossRef (w) A. Kamal, N. Shankaraiah, V. Devaiah, and K. L. Reddy, Tetrahedron Lett., 2006, 47, 9025; CrossRef (x) U. A. Kshirsagar, S. B. Mhaske, and N. P. Argade, Tetrahedron Lett., 200, 48, 3243; CrossRef (y) W. R. Bowman, M. R. J. Elsegood, T. Stein, and G. W. Weaver, Org. & Biomol. Chem., 2007, 5, 103; CrossRef (z) K. C. Jahng, S. I. Kim, D. H. Kim, C. S. Seo, J. K. Son, S. H. Lee, E. S. Lee, and Y. Jahng, Chem. Pharm. Bull., 2008, 56, 607. CrossRef
12. C.-S. Lee, C.-K. Liu, Y.-L. Chiang, and Y.-Y. Cheng, Tetrahedron Lett., 2008, 49, 481. CrossRef
13. J. Bergman and S. Bergman, J. Org. Chem., 1985, 50, 1246. CrossRef
14. T. Naito, Y. Hirata, O. Miyata and I. Ninomiya, J. Chem. Soc., Perkin Trans. I., 1988, 2219. CrossRef
15. F. Yamada, Y. Saida, and M. Somei, Heterocycles, 1986, 24, 2619. CrossRef
16. (a) A. Bischler and B. Napieralski, Chem. Ber., 1893, 26, 1903; (b) L. L. Miller, F. R. Stermitz, and J. R. Falck, J. Am. Chem. Soc., 1973, 95, 2651; CrossRef (c) B. Pal, P. Jaisankar, and V. S. Giri, Synth. Commun., 2003, 33, 2339. CrossRef
17. M. Schroder, Chem. Rev., 1980, 80, 187. CrossRef
18. (a) W. Yu, Y. Mei, Y. Kang, Z. Hua, and Z. Jin, Org. Lett., 2004, 6, 3217; CrossRef (b) T. E. Nielsen and M. Meldal, Org. Lett., 2005, 7, 2695. CrossRef